25
Zirconia as a Viable Implant Material in Implant Dentistry
Sammy Noumbissi1,2 and Saurabh Gupta1,3
1 Zirconia Implant Research Group, Silver Spring, MD, USA
2 Private Practice Dental Implantology, Silver Spring, MD, USA
3 Private Practice Dentistry, Bangalore, Karnataka, India
Current Problems in Implant Dentistry
There have been over five decades of successful implant dentistry. Starting in the sixties, the material of choice was and continues to be titanium and titanium alloys. Dental implants simply revolutionized dentistry and as time went by dental implants evolved micro and macroscopically. Thread design and surface enhancements all contributed to successful and predictable implantation. The improvements continued with the alloying providing implants with improved biomechanical performance and stability. All these improvements and developments led to implants being adopted and used at an exponential rate; teeth replacement with dental implants became more widespread with increasingly diverse and divergent outcomes. One of the emerging concerns with dental implants among others was a rising trend toward degradation of peri‐implant tissues which initially was mostly explained as a result of systemic health issues or poor oral hygiene. Then reports came about with regard to some patients being sensitive to the alloy components of the implants, a fact that has been very well investigated and established in orthopedics. It has also been well documented that just like orthopedic devices, dental implants are vulnerable to corrosion and release in the process alloy components which can lead to a wide range of health issues including peri‐implant inflammation and bone loss. This has led to the search for alternative materials started in the sixties by Prof. Sami Sandhaus who investigated ceramics (Alumina) as implant materials. In the mid to late nineties, many of the initial challenges with ceramics as implant materials were solved; today there is a growing popularity and demand especially from patients for metal‐free teeth replacement solutions and an increasing number of manufacturers are adding ceramic implants to their portfolio. The trend recently is pointing toward a fast adoption of zirconia implants; however, the few questions that remain in the minds of many clinicians are among others can zirconia be regarded as a viable implant material in implant dentistry, does zirconia have the same micro‐ and macroscopic features as their metal alloy counterparts. In this chapter, we will present and discuss the evolution and challenges faced by metals (especially the complications due to peri‐implant diseases) and how ceramics are living up or offering to address those challenges.
Corrosion
Corrosion is a phenomenon that is characterized by the gradual degradation of metals by chemical and/or electrochemical reaction with their environment. The type of corrosion observed with dental implants falls under the category of wet corrosion since they are placed and function within the oral cavity which is a wet environment with saliva and bodily fluids acting as electrolytes. Altogether implant corrosion is an electromechanical event most specifically with regards to restored dental implants. Given the number of dissimilar alloys used in implant prosthetic components, the most prominent factor of corrosion on restored implants in the oral cavity is galvanism. The use of fluoride‐containing oral hygiene products has also been found to have an impact on the corrosion behavior of implants and higher incidence of corrosion have been reported with increased anion concentrations [1]. There are two major factors in the breakdown of titanium alloys: first, the environment in which they are placed and second the material’s chemical and physical properties. In the sixties, Ferguson et al. first documented elevated titanium levels in peri‐implant tissues and 30 years later Bumgardner and co‐workers reported that there was increased release of metal ions from implants because of galvanic couples correlated with increased implant and peri‐implant tissue breakdown [2, 3].
Furthermore, it is well known that diet, lifestyle, and oral habits greatly influence the pH level of the oral cavity thus the implant’s reaction to the environment within which it is placed. Another factor in implant corrosion is electrical since upon prosthetic rehabilitation implants are connected to components such as abutments and screws, which alloys are dissimilar to that of the implants themselves. This results in coupling between the implants and the prosthetic components resulting in a galvanic reaction where the implant generally is the anode and releases ions in the peri‐implant tissues. It has been found and proven that titanium and various alloying elements leach into the crevicular space around implants [4]. Cortada et al. used the inductively coupled plasma‐atomic emission spectroscopy (ICP‐AES) technique to confirm the release of metallic ions as a result of titanium implant galvanism [5]. Other factors such as scratching of the implant surface, wear, fretting, erosion, cracking, mechanical stress, and material fatigue have been documented to accelerate or at the very least facilitate the corrosion process on dental implants (Figure 25.1) [6, 7]. Titanium, like other active‐passive metals when exposed to oxygen, is covered by a protective oxide layer of TiO2; even though TiO2 is thermodynamically stable it remains vulnerable to chemical and mechanical challenges which result in the release of alloy elements through a passive dissolution process.

Figure 25.1 Tribo‐Corrosion on the implant platform and prosthesis.
Clinical Significance of Corrosion
Although titanium alloys have better corrosion resistance properties compared to Co–Cr and other implant materials, their corrosion leads not only to the dissolution of titanium but also to other alloys in their composition, such as aluminum, vanadium, chromium, etc. Kirkpatrick et al. in an in vitro study isolated and demonstrated the complex sequence of events at the biomaterial‐tissue interface. In the study, they evaluated the modulation role and influence of specific metal ions and corrosion products in the function of the participating cell types. They investigated and presented the mechanism and pathophysiology of impaired wound healing around dental and maxillofacial implants and their impact on healing and maintaining peri‐implant health [8]. Galvanic corrosion in dental implants manifests itself in two ways; first through biological effects, such as soft tissue discoloration, pigmentation, and localized inflammatory reaction to the leached alloy components, and secondly by the loss of bone as a result of osteolysis. The osteoclastic activity increases in presence of inflammatory mediators. The release of these alloy elements and metal ions induces a host response that can be limited to the peri‐implant tissues or extend to distant organs and even the brain in the case of aluminum. The leached metal ions may induce potentially osteolytic cytokines into tissues leading to implant loosening and may even cause severe allergic reactions or hypersensitivity. There have also been reports in the literature of tumors and malignancies related to metal ions released from dental implants [9].
Osteolysis and Bone Loss
Olmedo et al. demonstrated that corrosion‐induced ionic leaching could be responsible for peri‐implantitis and implant failure. The particles that are leached as a result of the corrosion process are phagocytized by macrophages as part of host response release mediators of inflammation in the form of cytokines through the inflammatory cascade. These cytokines interfere with the production of osteoblasts thereby causing an elevated osteoclastic activity which leads to peripheral osteolysis and eventual loosening of the implant. Cytotoxic tissue response to corrosion release of several elements from various titanium alloys used in dentistry has been widely investigated and documented [10]. Furthermore, ions released because of corrosion have been demonstrated to contribute to peri‐implant bone loss by inducing localized pain and inflammation without the presence of infection around the implant, this interesting phenomenon is called aseptic osteolysis. Osteolysis takes place because of the phagocyte cascade activation when osteoclastic activity is increased [11, 12] but without the presence of infection. Several studies have further shown that the cellular uptake of hexavalent chromium is many folds greater than the trivalent chromium ion and its increased uptake causes a reduction of the alkaline phosphatase activity of the osteoblastic cells [13]. Furthermore, there have been reports of peri‐implant carcinogenic and mutagenic activity associated with these metal ions release [14].
Peri‐implantitis
Articles on the prevalence of peri‐implant diseases showed that 90% of peri‐implant tissues had some form of inflammatory response and a prevalence of peri‐implantitis from 28 to 51% according to a recent paper [15]. Peri‐implantitis is an inflammatory process around an osseointegrated implant, including soft tissue inflammation and progressive loss of supporting bone beyond biological bone remodeling. This inflammatory process is the leading cause of implant failures in the long term. Peri‐implantitis results from biofilm dysbiosis deposits surrounding the peri‐implant mucosa, meaning that bacterial deposition is essential to develop the inflammatory reaction. In addition, research on bacterial adhesion on titanium has revealed that titanium corrosion increases with plaque accumulation. On this matter, zirconia implants have been suggested as a peri‐implant mucosa‐friendly alternative. Recent studies show intriguing results promoting the advantage of zirconia implants in this matter regarding biofilm formation and bacterial adhesion on the surface of zirconia implants. An in vitro study comparing biofilm formation on zirconia versus titanium disks using three‐species biofilm or human plaque samples in an anaerobic flow chamber mode found that zirconia showed a lesser biofilm thickness and mass compared with titanium [16]. However, the biofilm metabolism was similar for both materials. These results suggest that zirconia implants accumulate less plaque therefore leading to less peri‐implant inflammation compared to titanium implants. With regards to the bacterial adhesion on zirconia, the Grossner–Schreiber group found that bacterial counts were higher on titanium discs compared to zirconia, while Scarano et al. showed that bacterial adhesion was significantly higher with pure titanium surfaces as compared with zirconium dioxide surfaces [17]. Similar data by Sadid‐Zadeh and collaborators also supported these findings. Moreover, these results are also supported in animal models [18]. A study comparing ligature‐induced peri‐implantitis around zirconia and titanium implants in dogs found that the first presented less crestal peri‐implant bone loss and no implant failure. Whereas the titanium implant group showed greater levels of bone loss and one implant failure due to peri‐implantitis [19]. Additionally, a recent systematic review of 15 studies with meta‐analysis indicated that zirconia accumulated less initial oral biofilm parameters thanks to the lesser roughness of the zirconia surface [20]. On the other hand, taking into consideration the role of host response in peri‐implantitis, a recent study compared the expression of immunoinflammatory markers in the tissues around ceramic and titanium implants. The authors found a similar number of inflammatory cells (T‐ and B‐cells) in mucosa surrounding ceramic implants when compared with titanium ones, concluding that the cellular response to the inflammatory challenge provoked by the biofilm around implants is the same, independent of the material with which the implant is made [21]. Besides, the hypothesis has been raised in the literature that not only biofilm is responsible for peri‐implantitis around titanium implants, but also foreign body reaction, allergies, cement excess, or even metallosis. Considering all the factors discussed above, peri‐implantitis is multifactorial and primarily a clinical manifestation of a complex inflammatory response. However, it could be assumed that zirconia implants present a lower incidence of peri‐implantitis when compared with titanium implants. Furthermore, when loosening occurs with ceramic implants, it is mostly aseptic, possibly pointing to a different mechanism of de‐osseointegration. Nevertheless, according to a systematic review and meta‐analysis, owing to the heterogeneity of assessment and short‐term evaluation of zirconia implants in clinical studies, results are found to be inconclusive with regards to inflammatory markers in zirconia peri‐implant mucosa [22]. On the other hand, one prospective study that followed up zirconia implants for 7.8 years found no difference between teeth (control group) and implants regarding the bleeding index, attachment level, or bacterial colonization. Interestingly, zirconia implants presented less plaque accumulation and recession, but greater probing depths when compared with natural dentition. Moreover, another systematic review concluded that zirconia implants had less plaque accumulation and less inflammation around the peri‐implant mucosa [21–23].
Immune Reactions to Metal Alloy Implants
Cellular and humoral immunity are the principal pathway mechanisms of the immune system. The immune system response and inflammation require well‐engaged and active biochemical processes to restore homeostasis, which ultimately leads to osseointegration of the implant. The immune response has critical factors such as cytokines and soluble substances produced by different kinds of immunocompetent cells whereby, they affect each other. Up to this date, there are several studies carried out in the field of implant rejection from the immunologic viewpoint and the alterations in the peri‐implant environment.
Cellular Immunity
Poor osseointegration and chronic inflammation are two primary and interrelated factors that cause peri‐implantitis. Infiltration of immune cells is an important component of the immune response that significantly impacts the biocompatibility of dental implants and can lead to implant failure or the absence of osseointegration. The initial injury of peri‐implant tissue triggers an inflammatory response mediated by the cells of innate immunity, such as macrophages, dendritic cells (DC), mast cells, and neutrophils [24].
Humoral Immunity
Most of the interrelation between cells of the immune system is modulated by several factors such as cytokines, growth factors, and hormones. Cytokines have an undeniable role in the intercommunication of the immune cells in the inflammatory cascade, which manifest itself clinically. Cytokines are messengers in cell‐to‐cell interaction that are critical for the pathogenic process of peri‐implantitis.
TNF‐α is a proinflammatory cytokine and is at the beginning of almost every immune response based on the individual’s sensitization to titanium. Thus, TNF‐α plays a key role in the failure of implants especially in the case of genetic incompatibility. During the function, metal particles are released from the implant as a result of wear and fatigue, they mostly accumulate in the peri‐implant tissues causing an increase in proinflammatory activity of non‐specific immune cells (tissue macrophages and monocytes), as a result of the exposure to particulate debris, i.e. Ti particles. It is known that such particles (diameter 1–10 μm) are consistently released into the environment surrounding implants as a result of mechanical abrasion, chemical, bacterial, and galvanic corrosion which as mentioned above promote hyperinflammatory conditions. Sterner et al. investigated the effects of clinically relevant aluminum ceramic, zirconium ceramic, and titanium particles of varying size and concentration on TNF release in a human macrophage system. They suggested that analysis of cytokines in the peri‐implant crevicular fluid (PICF) may be a good indicator to estimate the prognosis of dental implant treatment [25]. Several studies confirmed the negative role of proinflammatory cytokines on the longevity of dental implants; therefore, measuring these cytokine levels, especially IL‐1β, TNF‐ɑ, and IL‐6, seems more reliable to predict the fate of implants [26].
Allergies/Metal Hypersensitivity
The scientific literature has for the longest reported on the success of titanium implants; however, in the last ten years, the amount of research and clinical reports on peri‐implantitis and titanium sensitivity has increased significantly. A general search of the literature containing the word “peri‐implantitis” on PubMed between 2012 and 2022 returned 2995 results and for the first half of 2022, 345 results were returned. This is reflective of the seriousness of the peri‐implantitis problem (Figure 25.2).
With regards to metal sensitivity, it has not been directly correlated with peri‐implantitis but appears to have some common causative factors such as release of ions which in the case of dental implants is a result of corrosion. Dental research and literature on metal hypersensitivity related to dental implants have also been gaining momentum, between 2006 and 2022 there were 83 results on the subject (Figure 25.3) and in the last four years 37 publications were published on the problem (Figure 25.4).
Nevertheless, the tribo‐corrosion process releases titanium ions into the surrounding tissues that trigger a cascade of reactions that can be localized, in distant organs or even systemic reactions. As demand grows for implant‐based dental treatments, the range of implants available on the market is expanding exponentially [27]. When it comes to product development, the choice of implant material, surface treatment, and post‐manufacturing cleaning techniques, and packaging are key factors that will characterize the finished product. However, competition between brands is tough, a situation that sadly sometimes places business interests and cost‐cutting before quality control. Duddeck et al. showed greater carbon contamination, possibly related to cleaning procedures after surface treatments, which affect the protein and cell adsorption process [28]. Likewise, Massaro et al. mention that the presence of Na, K, and Cl contaminants, occasionally detected by X‐ray Photoelectron Spectroscopy (XPS) and High Vacuum X‐ray Energy Dispersive Microanalysis (HV‐EDX) on implant surfaces, may be residues of proprietary cleaning treatments performed after sandblasting. The biological role of these contaminants remains unclear [29]. Various tests for allergy to titanium are described in the literature, the patch test being the most frequently used [30]. There are other modalities to assess or predict the likelihood of sensitivity to certain metals; the MELISA test is one that uses lymphocytes’ response to metals and assists with an 85% accuracy in determining the likelihood an individual will be sensitive to a given metal. The scientific literature on this topic is very scarce, which highlights the need to establish a protocol for those patients who are sensitized to titanium before and after the placement of dental implants. In patients with a history of metal allergies, it is advisable to perform an allergy test and preferably the implant panel from the MELISA test. The alloy and its purity must be identified together with any trace elements it may contain in order to optimize diagnosis. When dental implants are placed in the jaws, and the patient eventually proves allergic to titanium or its alloys, the option of explanation must be considered, basing the decision on the ratio of risk/benefit to the patient. After having removed titanium implants, if the patient shows no clinical improvement, the option of removing other metal prostheses from the organism should also be considered. In this context, zirconium dioxide implants (ZrO2) offer a promising alternative to titanium as no case of allergy to zirconium oxide has ever been reported. Nevertheless, in animal experiments, zirconia implants have been shown to release negligible non‐reactive particles [27].

Figure 25.2 Trends in the Peri‐implantitis literature. (a) Last 10 year data, (b) 2022 (till date).

Figure 25.3 Dental implant metal hypersenstivity [2006–2022].

Figure 25.4 Dental implant metal hypersentivity [2017–2022].
Zirconia as an Implantable Material
Over the past few decades, dental implant procedures were applied to support single crowns as well as fixed or removable partial or full dentures. Early osseointegration is a critical factor for the clinical success of oral implants. Titanium has shown a high biocompatibility, resistance to corrosion, and favorable mechanical properties, these characteristics are particularly relevant in the early osseointegration phase of the healing period. Nevertheless, with the popularity and the exponential number of implants placed, failures of titanium implants have become quite common. To improve titanium surfaces and enhance osseointegration, several methods have been used; the most commonly used method is to coat the surface of a titanium implant with a non‐loading bioceramic, such as hydroxyapatite. Zirconia was first introduced to implant dentistry in the form of coatings to improve osseointegration of the titanium implants. As it was further investigated, the unique characteristics of zirconia such as its superior toughness, strength, corrosion resistance, and good aesthetic value made it an ideal implant material [16, 30, 32–36]. Once, material scientists, clinicians, and patients realized that the accumulation of titanium particles was detectable in tissues close to the implants, local lymph nodes, and even distant organs, they began to reconsider their view of titanium as the ultimate, viable, and biocompatible implant material. Reports of sensitivity and allergy resulting from the placement of titanium implants have been on the rise [36–39]. As of now, there are no reports on the toxicity of zirconia implants and its tooth‐like color offers the possibility of staining zirconia thereby elevating the aesthetic scores and patient satisfaction. The successful use of zirconia as a material for dental implants also lies in its excellent osseointegration. In vivo studies have shown that zirconia osseointegration is on par with that of titanium with regard to cell metabolism and positive tissue response under immediate and delayed loading conditions [39–43]. Furthermore, the inflammatory response and bone loss around zirconia implants are less intense than that induced by titanium implants, suggesting its superior biocompatibility [44]. In the quest to achieve more predictable osseointegration, especially in difficult clinical sites, researchers have attempted to modify zirconia surfaces to enhance bone cell attachment, growth, proliferation, and differentiation. Various methods of surface enhancement of zirconia implants have been investigated and reported for zirconia, some protocols have been reported as preclinical results and others are commercially available. Most of the surface modification procedures studied promoted superior cell responses and qualitative osseo‐ and muco‐integration [45, 46].
Manufacturing Processes of Zirconia
It should be noted that zirconium, like titanium in its earthen state, is also a metal. However, they both belong to the group of transitional metals that when exposed to oxygen under controlled conditions and proprietary protocols produce the ceramic zirconium dioxide which is also known as zirconia. This process is not reversible therefore zirconium can be formed from the zirconia and reversal to the metal state and properties is not possible. However, not all zirconium dioxides are the same. There are major differences in the manufacturing process, powder selection, surface design, and handling of individual systems [46].
Whether its tetragonal zirconia polycrystals (TZP), tetragonal zirconia polycrystals‐Alumina (TZP‐A), or Alumina toughened zirconia (ATZ), processing of the starting material is important. Grain size, purity, and density have a decisive influence on hardness, stability, and quality. Although very recently 3D printing of ceramics has appeared, hard machining and injection molding are the two main processes for the manufacture of commercially available zirconia implants [47]. Zirconia at 100% responds poorly to humidity and will age prematurely under such conditions. Therefore, modern ceramic implants consist of partially stabilized tetragonal zirconia polycrystals (TZP), which are polycrystalline ceramic composites and consist of zirconia, yttria, and alumina; depending on the manufacturer these components vary in percentages with good mechanical properties and have an average bending strength of 1100 MPa. To increase the bending strength to 1200 MPa, 0.5% by volume of aluminum oxide (alumina) is added to the starting powders (TZP‐A). Zirconia being hydrophobic, most manufacturers add Yttria (Yttrium dioxide) to the composite to stop the low‐temperature aging process caused by hydrothermal degradation [48]. In the case of ATZ ceramics, manufacturers will increase the volume percentage of alumina to 20% thereby obtaining a hybrid polycrystalline ceramic that can achieve flexural strength up to 2000 MPa. This ATZ‐ceramic significantly reduces the risk of fracture. The aging process (phase transformation) from tetragonal to monoclinic (t → m) no longer has any clinical relevance thanks to these procedures and the addition of yttria [49].
The state of zirconia implants once the manufacturing is complete is in the tetragonal phase which is maintained with the addition of stabilizers such as yttria. Stabilizers are included to limit the phase transformation of the ceramic which occurs with the ceramic reverting to a less stable and fracture‐prone phase also known as monoclinic. However, once the implant is placed, clinicians must be careful with manufacturers’ recommendations as phase transformation of the material must be expected when chairside grinding of the zirconia with rotating instruments. Uncontrolled grinding with coarse‐grained diamonds and insufficient cooling of the material can induce micro‐cracks within the outer surface layer of the material that can propagate and grow into the underlying material structure. However, the stabilizers confer “self‐healing” properties to zirconia by volume expansion as a result of phase transformation which effectively helps stop the propagation of mechanically induced micro‐cracks. There are however limits to this “self‐healing mechanism” of zirconium dioxide because once this unique compensatory capacity has been exceeded, it weakens the zirconia, makes it vulnerable to humidity, and leads to premature aging and ultimately material catastrophic failure [46, 49].
During the early stages of improvement of zirconia implant surfaces, high blasting pressure and large particles were used to roughen the surfaces of ceramic implants. As a result, micro‐cracks were found in the surface structure of the implants, which led to fractures under function. A great number of fractures of ceramic implants can be traced back to an improper industrial manufacturing process or the result of poor post‐production mechanical handling by the clinician. The processes by which the surface micro‐roughness of a zirconia implant is achieved have evolved with the advances in material processing and a better understanding of phase transformation. Today manufacturers are using proprietary acid etching techniques or in the case of injection molded manufacturing, the surface roughness is built into the mold thereby negating the need to grind or use etchants. Some manufacturers claim this is a way to avoid manufacturing‐induced contamination of the implant surface [50].
Hard Machining Process
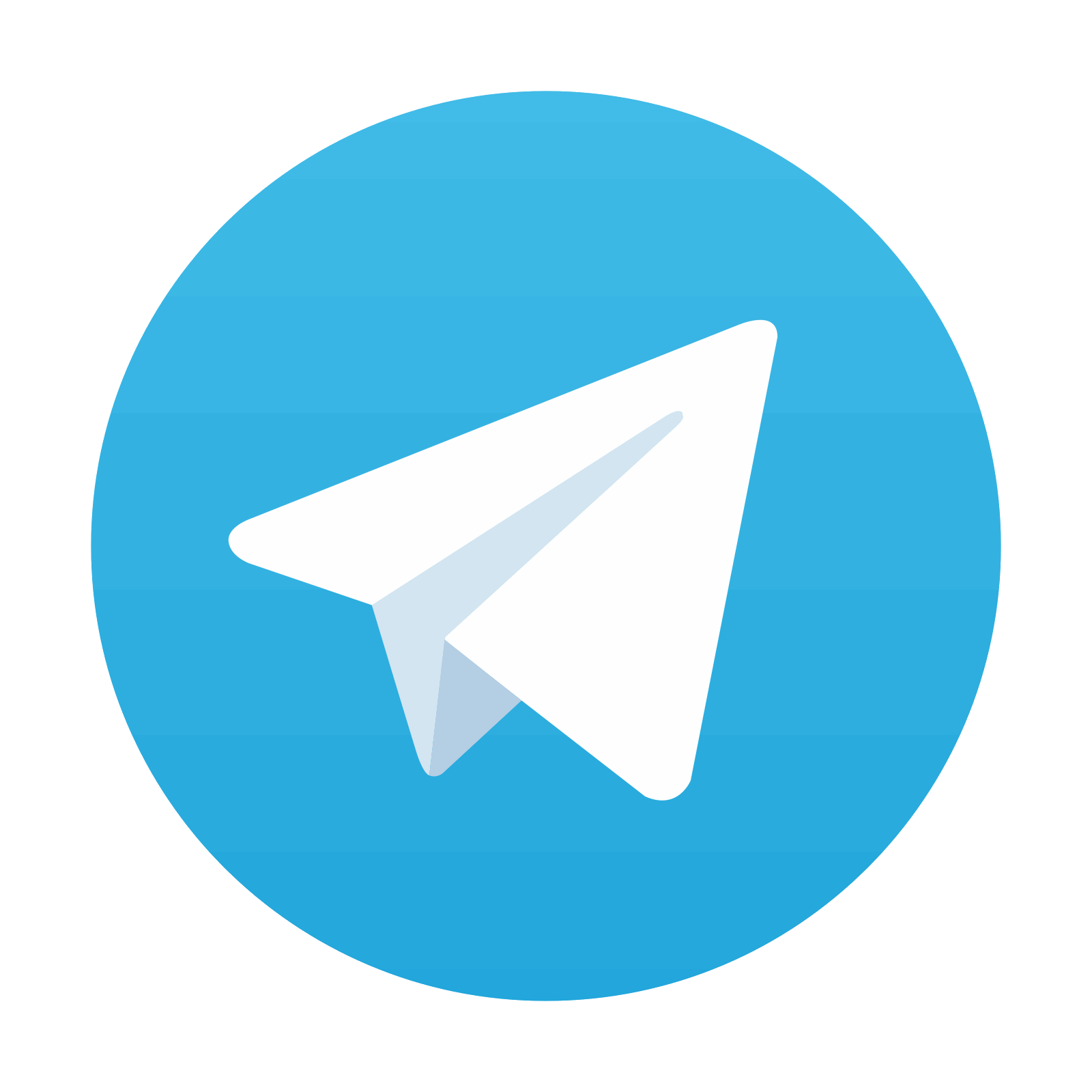
Stay updated, free dental videos. Join our Telegram channel

VIDEdental - Online dental courses
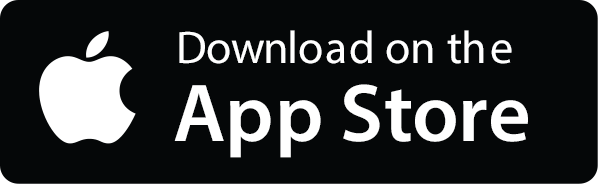
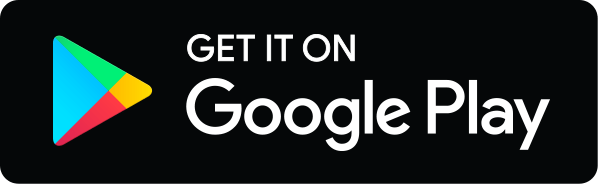