Implant surface micro and macro topography plays a key role in early osseointegration. The physicochemical features of the implant surface (ie, chemical composition, hydrophobicity/hydrophilicity and roughness) influence the deposition of extracellular matrix proteins, the precipitation of bone mineral, and the stimulation of cells. Modification of the implant topography provides better primary stability and faster osseointegration, allowing for immediate placement or immediate loading. Randomized clinical trials are warranted to compare the response of osseointegration with various implant micro and macro surface topographies in people with various local or systemic risk factors.
Key points
- •
Micro and macro properties of an implant surface determine the magnitude of the biologic response. Therefore, they influence the rate and quality of early osseointegration.
- •
Modifications to implant surfaces have enhanced long-term implant success. Because of the risk of peri-implantitis, the identification of an optimal implant surface topography is needed.
- •
Selection of an implant system and topography is based on the clinical circumstances, as well as the clinician’s skills and familiarity with the manufacturer’s protocols.
Introduction
The biological fixation between an implant surface and bone is defined as osseointegration (OI). During OI, direct bone-to-implant contact (BIC) is established without an intervening connective tissue layer. Over the last few decades, the influence of implant surface characteristics on the biological response has been extensively investigated. The physicochemical properties of the implant surface influence the reaction of surrounding tissue. This occurs between 4 and 14 days after implant insertion. The reactions include upregulation of gene expression and induction of angiogenesis, osteogenesis, and neurogenesis, through cellular responses such as cell adhesion, proliferation, differentiation, and migration. Enhancement of implant surfaces to improve early OI has led researchers to investigate OI in relation to bone mineral precipitation, protein deposition, and cell stimulation. The exact implant micro and macro surface characteristics necessary for optimum OI remain to be identified.
The topographic parameters that have been identified to determine the success of OI include (1) the chemical composition, (2) the hydrophobicity/hydrophilicity of the surface, and (3) the roughness of the implant surface. This article focuses on the effect of implant surface designs and characteristics on cellular responses that relate directly to early OI. This is the basis for current treatment protocols, including immediate or early loading.
Chemical composition
Biocompatibility
A biomaterial is determined to be biocompatible when the material or its degradation products do not induce inflammatory reactions. A biocompatible material also allows for the regulation of adsorption and configuration of proteins within the extracellular matrix (ECM). The molecular composition and 3-dimensional structure of the ECM forms a tissue scaffold for cell migration and anchorage. Extracellular matrix proteins, such as collagen, fibronectin, and vitronectin, bind to integrins on osteoblasts, stimulating intracellular signaling pathways within the cytoplasm, cytoskeleton, and nucleus. These signaling pathways result in the upregulation of transcription factors that are responsible for the expression of bone matrix formation genes.
Commercially Pure Titanium and Titanium Alloy Implants
The primary factor contributing to the biocompatibility of commercially pure titanium (cpTi) is the spontaneous build-up of a stable and inert oxide layer. This oxide coating is highly adherent and is spontaneously capable of repairing itself if damaged by the surrounding environment. However, physicochemical differences exist when a titanium alloy is created. Table 1 .
Grade | Composition | Physical Properties |
---|---|---|
1 | Commercially pure titanium Oxygen 0.18% max Nitrogen 0.03% max Carbon 0.08% max Iron 0.2% max Hydrogen 0.015% max Titanium 99% |
|
4 | Commercially pure titanium Oxygen 0.4% max Nitrogen 0.05% max Carbon 0.08% max Iron 0.5% max Hydrogen 0.015% max Titanium 99% |
|
5 | Ti 6Al-4V titanium alloys Oxygen 0.2% max Nitrogen 0.05% max Carbon 0.08% max Iron 0.4% max Hydrogen 0.015% max Aluminum 5.5%–6.75% Vanadium 3.5%–4.5% Titanium 90% |
|
Titanium (Ti) grade 1 has the lowest strength, but it has the highest formability and corrosion resistance. Titanium grade 4 has very high strength, with moderate formability. On the other hand, Ti grade 5 (also known as Ti-6Al-4V alloy) is the strongest among other Ti alloys. It has superior corrosion resistance, high fatigue strength, improved fracture resistance, and low elastic modulus, which reduces stress shielding. The Ti-6Al-4V alloy is preferred in the posterior jaw, where occlusal forces are high. However, cpTi implants have demonstrated higher removal torque values and higher bone contacts than Ti-6Al-4V implants.
Studies have shown that osteoclasts corrode Ti surfaces. In addition, extracellular fluids contain metal-binding proteins. The combination of corrosion and metal-binding proteins causes the release of titanium debris into the surrounding soft tissues, thereby inducing inflammatory reactions. Ti alloys release vanadium and aluminum (detectable locally and systemically in tissues), causing possible toxic effects. Vanadium-free and aluminum-free Ti alloys have been introduced, and nontoxic elements such as zirconium, niobium, tantalum, palladium, and indium have been incorporated to enhance biocompatibility. Binary titanium zirconium (TiZr) alloy has been shown to retain biocompatibility by maintaining the same alpha structure as cpTi. In addition, it is not affected by sandblasting, large grit, acid-etched (SLA), and SLActive treatments. TiZr is particularly indicated for use in small-diameter implant applications because of improved strength. However, further ongoing comparative studies on the different surface characteristics need to be conducted to assess these new Ti alloys for their potential use in clinical application.
Zirconium implants
Zirconium is highly reactive with water and oxygen. During this reaction, a crystalline dioxide, zirconia (ZrO 2 ), is formed. The alloying of ZrO 2 with yttrium (Y 2 O 3 ) produces a stable tetragonal structure at room temperature known as yttrium tetragonal zirconia polycrystal (Y-TZP). Y-TZP has increased fracture strength and toughness, increased corrosion and wear resistance, high flexural strength, and low thermal conductivity. Y-TZP can also be toughened by adding alumina. ZrO 2 has been shown to be as osteoconductive and biocompatible as Ti, provoking less tissue reaction and minimum ion leakage when compared with Ti.
Bone healing around ZrO 2 implants is similar to that around Ti implants. Intramembranous osteogenesis occurs and leads to the generation of woven bone. This process is initially accompanied by the formation of parallel-fibered and lamellar bone. Thus, trabecular bone is created earlier than compact bone.
ZrO 2 has been suggested to be clinically suitable because of its tooth-like color, which has the advantage of avoiding the complication of a bluish discoloration of the overlying gingiva. This can be seen and ascribed to crestal bone loss and gingival recession associated with metal implants. However, the clinical use of ZrO 2 dental implants is limited because surface modifications are difficult, and smooth ZrO 2 implant surfaces do not promote OI. Clinical trials with long-term follow-ups are warranted to evaluate the clinical performance and predictability of ZrO 2 implants before recommending them for routine clinical use.
Hydrophobicity/hydrophilicity (surface wettability)
The property of hydrophobicity and/or hydrophilicity (surface wettability) of an implant surface is important to OI. This is expressed by the water contact angle (CA) that ranges from 0° on very hydrophilic surfaces to greater than 90° on hydrophobic surfaces. Wettability is directly influenced by surface free energy (SFE), which is defined as the interaction between the forces of adhesion and the forces of cohesion, that is, the spreading of a liquid over the implant surface. Surface free energy is measured indirectly (quantified) by the liquid-solid CA. It is affected by the surface chemical composition, surface charge, and microstructural topography.
When surfaces are roughened by means of anodization, etching, blasting, or a combination of blasting and etching, CAs are changed, thus affecting the surface wetability. Increased CAs are usually associated with increased roughness on nontreated surfaces. However, modified surfaces that incorporate differences in roughness can show different CAs. A high SFE promotes increased wettability, thereby enhancing the interaction between the implant surface and the biologic environment. Extremely high SFE can promote cell adhesion, but decreases cell motility and impairs subsequent cell functions. Although hydrophilic surfaces maintain the function of proteins and possess a higher affinity for these proteins than hydrophobic surfaces, hydrophobic surfaces can cause partial denaturation of proteins by exerting conformational changes. As a result, a disturbance of their tertiary structure occurs causing cell-binding sites to be less accessible, consequentially diminishing cell adhesion. Furthermore, hydrophobic surfaces foster hydrocarbon contamination, which can lead to the entrapment of air bubbles thereby interfering with protein adsorption and cell receptor adhesion/activation.
Surface Wettability and Initial Blood Protein/Cellular Interactions
Hydrophilicity influences the selective adhesion of blood proteins and their composition, bonding strength, conformation, and orientation. This leads to the adhesion and activation of thrombocytes and subsequent formation of a blood clot. Activated platelets interact with the implant surface as well as with the fibrin scaffold and then degranulate into the extracellular environment. Degranulation causes the release of growth factors responsible for wound healing as well as the release of differentiation factors responsible for the recruitment, differentiation and proliferation of undifferentiated mesenchymal stem cells (MSCs). This then enhances the differentiation of osteogenic cells and angiogenesis, which promote bone regeneration.
Extracellular matrix proteins, such as fibronectin and albumin, bound to the conditioning layer of dental implants, show a marked reduction in their cell-adhesive function when adsorbed to hydrophobic surfaces. However, the cell-adhesive functionality of fibronectin is enhanced on hydrophilic surfaces, thereby improving a cellular response. The adsorption of type I collagen and vitronectin on hydrophilic surfaces is essential for osteoblast differentiation.
The interaction between blood and biomaterial also initiates the migration of polymorphonuclear leukocytes (PMNs) and macrophages to the wound site, which induces wound healing. Receptor expression and adhesion of PMNs are influenced by surface wettability. CD16 receptors on PMNs bind to hydrophilic surfaces, whereas CD162 receptors bind to hydrophobic surfaces. Macrophages involved in inflammation and maintenance of tissue homeostasis are activated by interferon gamma secreted from other inflammatory cells and lipopolysaccharide. A balance between pro-inflammatory macrophages (M1) and anti-inflammatory macrophages (regenerative) (M2) is also required for proper healing and biomaterial integration of the implant.
Smooth Ti induces M1 activation, as indicated by increased levels of interleukin-1b (IL-1b), IL-6, and tumor necrosis factor alpha. Hydrophilic rough titanium surfaces induce M2 activation, as depicted by increased levels of IL-4 and IL-10. M2 cells express fibroblast growth factors, transforming growth factors, epithelial growth factor, as well as bone morphogenetic proteins (BMPs), which induce angiogenesis. A decrease in gene expression of M1 markers on hydrophilic rough Ti surfaces has been shown compared with rough hydrophobic surfaces. In addition, increased wettability of microrough surfaces has shown to stimulate more anti-inflammatory cytokine released by macrophages than those on hydrophilic smooth surfaces. The synergistic effect of increased surface roughness and hydrophilicity may induce a microenvironment that reduces healing time and accelerates OI.
Dendritic cells remain in a more immature state when interacting with hydrophilic surfaces. As a result, there is a reduction in the innate immune response causing a noninflammatory environment, which promotes clinical peri-implant bone formation. Multinucleated giant cells derived from either osteoclasts or macrophages have been quantified on different surfaces and seem to be an integral part of the normal OI process. However, whether these cells are responsible for any true foreign body reaction around dental implants is still not clear, and their presence may not predict any future implant loss.
Surface Wettability and Cell Interactions with Preconditioned Surfaces
Adsorbed serum proteins possess cell-binding domains and function as biological signals that activate receptors (integrins) located on the outer membrane of cells. Integrins, which recognize a particular amino acid sequence within a protein, may also vary based on cell type and differentiation stage. After initial cellular adhesion, the short- and long-term processes of cellular proliferation and differentiation are determined.
Mesenchymal stem cells initially invade the blood clot and colonize the implantation site. The osteoblastic differentiation of MSCs is promoted when hydrophilic surfaces increase the expression of osteocalcin, osteoprotegerin, and integrins. Surface nanomodification induced by differences in surface wettability may be partly responsible for different contributions from osteoblast lineage cells at different stages of osteoblast commitment.
Increased osteogenic differentiation and maturation, as depicted by a significant upregulation of genes associated with the transforming growth factor β/BMP signaling cascade, is associated with increased hydrophilicity, thereby contributing to accelerated OI. Furthermore, implants with moderately rough surfaces with a high level of energy/wettability show greater trabecular density of newly formed woven bone, leading to an increase in the BIC ratio, as well as in the bonding strength of such contact.
Superhydrophilic surfaces, such as smoother acid-etched surfaces, compared with rougher blasted ones, may positively influence the epithelial seal around implants, as well as enhance keratinocyte proliferation, cell spreading, and motility. This results in faster surface coverage, leading to a faster restoration of a tight epithelial seal. Surface nanomodification, enhancing keratinocyte adhesion and spreading and filopodium extension, has been shown on e-beam-evaporated (nanorough) surfaces with intermediate surface energy compared with anodized (nanotubular) surfaces. Fibroblast morphology and activity may be enhanced on Ti surfaces coated with titanium nickel by physical vapor deposition compared with surfaces that were thermally oxidized or laser irradiated.
Surface Wettability and Bacterial Adhesion
There are contradictory data regarding the influence of surface roughness (Ra), SFE, and chemical alterations of Ti implants on biofilm formation. Ra values greater than 0.2 μm have been shown to lead to an increased rate of biofilm formation, whereas Ra values less than 0.2 μm may have no effect on supragingival and subgingival plaque formation, including no further quantitative or qualitative effects on the microflora. Hydrophilicity and Ra have been shown to drive initial biofilm adhesion and formation, whereas superhydrophilic, chemically modified acid-etched surfaces have shown significantly less initial biofilm formation. Moreover, hydrophobic SLA and machined surfaces increase hydrophobicity, which may be the main force for bacterial adhesion. This is attributed to interactions between hydrophobic implant surface properties and bacterial hydrophobic cell membrane components, as well as adhesins located on fimbria or pili.
Despite surfaces having high hydrophilicity, surface topography has the predominant effect on bacterial attachment, whereas surface charge and wetting have less influence. Microtopography may have a highly erratic influence on supragingival plaque biofilm formation. The attachment of certain bacteria has been shown to be higher on surfaces with nanophase topographies compared with conventional topographies. However, other studies have found nanophase materials to have a bacteria-repelling effect.
Although specific oral bacteria may have high adhesion affinity for titanium surfaces, the clinical situation is far more complex. The in vivo formation of conditioning films by serum proteins may alter the surface properties of biomaterials as soon as they encounter biofluids. Serum proteins may exert a greater effect on the surface adhesion and biofilm formation of oral bacteria in vivo than that observed in vitro. Bacteria use specific adhesion mechanisms to attach to specific serum proteins, such as fibrinogen, fibronectin, collagen, vitronectin, and laminin. Any conformational changes of these proteins during implant surface conditioning may also influence bacterial adhesion. Therefore, indirect biomaterial surface properties, such as chemical composition and nanoscale features, will influence the molecular conformation in the conditioning film during surface protein adsorption, with the latter having a greater influence on bacterial adhesion. However, bacterial attachment remains dependent on multiple factors. Different preferences for attachment to implant surfaces may be attributed to the specific bacteria present, as well as the physicochemical properties of implant surfaces.
Surface Wettability and Rate of Osseointegration
Hydrophilicity has a greater role in promoting early bone formation by enhancing osteoblastic differentiation in the early stages of OI compared with hydrophobic surfaces. A synergistic effect has been described between surface microroughness, superhydrophilicity, and bioactive implant surfaces. This leads to enhanced OI, thereby facilitating early loading capacity. Anodized surfaces displaying well-defined nanostructures, which have the lowest surface roughness and highest hydrophilicity, have been shown to promote the highest removal torque. This suggests that hydrophilicity elicits a stronger response compared with surface roughness.
Roughness of implant surfaces
A positive correlation has been described between surface roughness and bone response compared with the mechanical quality of the BIC interface. Surface-roughening procedures cause a modification of the surface chemistry. Therefore, it is still unclear whether a predictable implant-to-bone response is mainly because of surface roughness or the related changes in surface chemistry. Surface engineering methods (ie, mechanical, chemical, and physical) have been developed to create various surface compositions to potentially control the biological activity of the implant surface. In vitro studies showed that surface roughness affects the spreading, proliferation, and differentiation of osteoblastic lineage cells and protein synthesis, which leads to an improved BIC outcome. However, in vivo studies do not clearly differentiate whether de novo bone formation occurs by means of distance or contact osteogenesis. Histologic studies have shown signs of bone formation directly on implant surfaces; however, 2-dimensional tissue sections cannot reveal the true 3-dimensional features of the BIC. Depending on the dimension of the measured surface features, implant surface roughness can be divided into microroughness, macroroughness, and nanoroughness.
Biological Response to Macrorough Implant Surfaces
Macroroughness is directly related to implant geometry. Threaded screw and macroporous surface treatments range in scale from millimeters to tens of microns (μm). They present a relatively smooth surface and incorporate surface defects, such as grooves, ridges, and marks. Macroroughness enhances mechanical interlocking between the implant surface and surrounding bone, thereby enhancing primary implant fixation and long-term mechanical stability. Macrorough features include implant body shape (parallel and tapered) and various geometric thread patterns. In an attempt to preserve crestal bone, implants were designed with a tapered apex and larger cervical diameter (Nobel Speedy Groovy implants). However, tapered implants have greater crestal bone loss compared with parallel implants. Thread geometry affects the distribution of stress forces around the implant. Table 2 summarizes the effects and the clinical relevance of various macrorough geometric thread patterns.
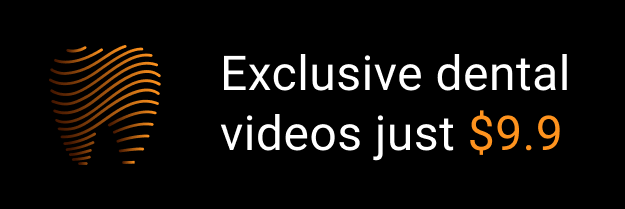