9
What Can Orthodontists Learn from Orthopedists Engaged in Basic Research?
Carlalberta Verna1 and Birte Melsen2,3
1 Department of Pediatric Oral Health and Orthodontics, University Centre of Dental Medicine UZB, University of Basel, Basel, Switzerland
2 Department of Orthodontics, University of Western Australia, Perth, Australia
3 New York University College of Dentistry, New York, NY, USA
A common language
As correctly indicated by the editors of this book, the face is a part of the whole body. Therefore, there is no reason to believe that the stomatognathic system would not follow general physiopathological rules that apply to the entire body. Basic research carried out by orthopedists can therefore be utilized by orthodontists. However, a precondition for a learning process is a common language.
Modeling
Regarding the biological reaction occurring as a result of orthodontic force systems, there is no lexical consistency between orthodontists and orthopedists, the former describing it as remodeling, the latter as modeling. In bone‐biological terms, bone remodeling and modelling are two distinctly separate processes, with different physiological roles.
Modeling is the term applied to changes in the shape and size of bones (macro‐modeling) resulting from resorption and formation activities, and is the dominant biological activity during growth. Modeling also occurs at the trabecular level, so‐called mini‐modeling (Frost, 1990). Resorption and formation drifts do not necessarily follow a given sequence; that is, they are biologically uncoupled. In adult life the physiological mesial drift of the alveolar socket is a result of modeling. Bone modeling reflects the structural adaptation of bone mass and shape to varying demands. It is thus largely controlled by functional and applied loads, and less by systemic metabolic requests. Formation drifts may occur in both lamellar and woven bone. Woven bone is a result of larger strain values than lamellar bone, and usually appears after fracture healing, in response to neoplasms or infections, and in reaction to large mechanical loads produced by orthodontic appliances (Frost, 1994; Figure 9.1).
Clinical implications of modelling
When teeth are moved “with bone,” modeling of the alveolar bone occurs, and thus the teeth can be moved beyond the immediate alveolar envelope while maintaining the marginal bone level. This principle underpins the treatment of partially dentate adult patients with atrophied alveolar bone areas (Mantzikos and Shamus, 1999; Chandler and Rongey, 2005). An example of so‐called tooth movement with bone is illustrated in Figure 9.2, where the tooth has been moved into an atrophic edentulous area, with “rebuilding” of the alveolar process, giving the impression that the tooth has carried the alveolus along to the new position.

Figure 9.1 Alveolar bone modeling following the application of 25 g of force. After 14 days of treatment, the microradiographic analysis (top) shows new bone formation ahead of the tooth in the direction of the force (red arrows). On the buccal side, new periosteal bone formation is observed, as shown by the wide strips of bone markers (white arrows).
Source: Verna et al. (1999b) / with permission from ELSEVIER.
When determining the force system necessary for a planned tooth movement, the quantity and quality of the bone surrounding the tooth should be taken into consideration. High‐resolution micro‐computed tomography (CT) scans of alveolar bone samples show that the bone surrounding the roots is highly variable in density and that the so‐called lamina dura is a nonuniform structure (Dalstra et al., 2006; Figure 9.3). Therefore, even very light forces may result in overcompression over small surfaces of the alveolar bone. This is in line with the finding that hyalinization is rarely avoidable (von Bohl et al., 2004), although the amount may vary.
Particular attention should be paid to the origin of the cells that will activate the modeling process. In the case of indirect resorption in areas dominated by dense cortical bone, for example in labial movement of lower incisors, tooth movement requires modeling of the cortical bone, and the osteoclasts should originate from the cortical bone or from the periosteum. Labial tipping of incisors in the presence of thin cortical bone increases the probability of bone fenestration (Baron, 1975). To reduce the risk of iatrogenic dehiscence, the initial force system should be directed at displacement of the apex of the root, which is usually surrounded by trabecular bone. Thereafter, the bending of the alveolar process will generate formation on the buccal side of the alveolar process. The use of controlled, uniformly distributed, low‐magnitude forces is recommended and, since the modeling rate of cortical bone is slower than that of trabecular bone, adequate time should be allowed for initiation of cellular activation and subsequent bone formation at the periosteal side. An example is shown in Figure 9.4, where the system was checked monthly to adjust the line of action of the force without activation.
In sum, the drift of alveolar bone occurring following application of an orthodontic force can be described as modeling, in response to modified functional demand. Since the anatomy of the teeth differs both within an individual dental arch and between patients, modeling of the alveolar bone without bone loss can only be achieved by use of customized biomechanical systems. However, based on this premise, it is difficult, if not impossible, to agree on absolute standardization of treatments.
Remodeling
As already mentioned, orthodontists do not distinguish between modeling and remodeling. Remodeling is a phenomenon that occurs throughout life in discrete locations in the whole skeleton. It involves cycles of resorption and subsequent formation, spatially and temporally coupled. Remodeling allows bone adaptation to mechanical stresses by minimizing fatigue damage and is influenced by the action of hormones and cytokines (Burr, 1993; Mori and Burr, 1993; Frost, 1998).

Figure 9.2 (a, b) Tooth movement with bone in an edentulous alveolar crest, where no bone is present. The alveolar drift allows for the movement of the whole alveolus (c, d), and the osteogenic potential of the periodontal ligament is fully expressed in the formation of bone.
Source: Melsen and Garbo (2004) / with permission from Quintessence Publishing Co., Inc.

Figure 9.3 (a) Mesiodistal and (b) buccolingual views of the alveolus of the canine. Note the uneven thickness of the alveolar bone proper and the sparse trabecular support.
Source: Dalstra et al. (2006) / with permission from John Wiley & Sons.
The bone remodeling cycle is carried out by a team of cells called the bone multicellular unit (BMU; Frost, 1986). The cycle in humans lasts 120–160 days, and is composed of four phases: activation, resorption, reversal, and formation (Eriksen et al., 1984). Although the stimulus necessary for the initiation of a new BMU is still not fully identified, it has been shown that microcracks can initiate remodeling via the sensory input to the osteocytes. In the activation phase, the lining cells, the preosteoblasts, change to a cuboidal shape and secrete a receptor ligand, receptor activator of nuclear factor kappa B ligand (RANKL), on the cell surface. The preosteoclasts within the bone marrow present receptors for RANKL on their cell membrane, called RANK. The interaction between RANK and RANKL determines the activation of the preosteoclasts, which then fuse and differentiate into mature multinucleated osteoclasts and resorb bone. The resorption of bone also occurs as a result of inhibitory activities directed toward osteoblasts, as in the case of the release of sclerostin (SOST), which inhibits bone formation and enhances osteoblast apoptosis (Winkler et al., 2003). The RANKL present on the cell surface of the preosteoblast is neutralized by a free‐floating decoy receptor belonging to the tumor necrosis factor (TNF) family, osteoprotegerin (OPG), thus controlling further activation of the preosteoclasts via RANK. Bone resorption lasts for about two weeks, after which the osteoclasts undergo programmed cell death or apoptosis.

Figure 9.4 (a, b) Modeling of the alveolar bone achieved by bodily movement of a premolar in an atrophic area. (c, d) Following the insertion of a mini‐implant (Aarhus® system, Medicon, Tuttlingen, Germany), the biomechanical system consisted of a box loop in titanium molybdenum in a 0.017 × 0.025 in. archwire activated to apply a distal force and uprighting moment. This enabled bodily tooth movement, with uniform distribution of the force in the periodontal ligament. Anchorage was ensured by the use of a 50 g superelastic coil spring attached to the skeletal anchorage device. (e, f) The same biomechanical system was used to achieve the final displacement, and at each appointment the appliance was adjusted to maintain the force‐to‐moment ratio for continuation of the translation movement.
Source: Melsen and Garbo (2004) / with permission from Quintessence Publishing Co., Inc.
Following resorption by the osteoclasts, the lacuna is deepened by mononuclear phagocyte cells (Eriksen et al., 1984). At the end of this process the reversal phase is initiated, and further preosteoblasts migrate into the resorbed cavity and differentiate into osteoblasts. The interface between the resorbed old bone and the new‐formed bone is called the cement line, or reversal line, and these osteoblasts now start to form bone. The precursor preosteoblasts are probably attracted by bone‐derived growth factors (such as transforming growth factor‐beta, TGF‐beta) and are derived from marrow stromal cells. Through a positive feedback mechanism the preosteoblasts also secrete growth factors, osteopontin, osteocalcin, and other proteins, such as insulin growth factor‐1 (IGF‐1), interleukin (IL)‐6, bone morphogenetic proteins (BMPs), and fibroblast growth factors (FGFs), all of which enhance the osteoblast’s activities. Fibroblasts also migrate toward the cement line and secrete thin collagen fibrils around which the osteoblasts start synthesizing osteoid. The osteoid is nonmineralized bone matrix that cannot be resorbed by osteoclasts. The mineralization process starts when the osteoid thickness reaches about 6 μm. After about four months the resorption cavity is filled with new bone, although not completely, with closely packed mineral crystals and subsequent increase in bone density.
It is essential for the orthodontist to understand the role of remodeling following the application of an orthodontic load. Bone formation has been observed not only in the periodontal ligament (PDL) tension side, but also in the direction of the force (Mohri et al., 1991; King et al., 1992); bone resorption has also been observed at this site (Mohri et al., 1991; Zaffe and Verna, 1995; Melsen, 1999; Figure 9.5). The presence of markers of both resorption and formation adjacent to the alveolar wall reflects the coupled activity of osteoclasts and osteoblasts that characterizes what orthopedists describe as bone remodeling. Synchronized initiation of numerous BMUs results in bone turnover on both the pressure and tension sides in the PDL (Tanne et al., 1990). The RANKL–RANK mechanism has been shown to be active in the PDL and is strengthened by a mechanical load (Ogasawara et al., 2004). Tooth movement can be accelerated by local delivery of RANKL (Kanzaki et al., 2006), whereas the delivery of OPG decreases the rate of tooth movement (Oshiro et al., 2002; Kanzaki et al., 2004). A lack of OPG has been found to be associated with a significantly higher level of bone resorption in the compression area, whereas the upregulation of RANKL induced by orthodontic forces depends on the presence of prostaglandin (PG) E2, which is released by osteoblasts and osteocytes (Kanzaki et al., 2002; Yamaguchi, 2009).
It is clear, therefore, that application of a mechanical load initiates remodeling as well as bone modeling. The variation in the quality of remodeling between patients may explain the individual variations in tissue response to orthodontic treatment.
Clinical implications of bone remodeling
Cortical anchorage was defined by Ricketts (1998) as movement of the roots toward the cortex of the alveolar bone to resist the active force of the appliance. The biological principle behind cortical anchorage is utilization of the differential turnover rates of cortical and trabecular bone, the former being 5–10 times slower than the latter (Steineche and Hauge, 2003). Teeth closer to the cortical bone need more time to be moved, but at a later stage anchorage loss will occur. Absolute anchorage can, however, only be achieved with ankylosed teeth or skeletal anchorage devices (Melsen and Costa, 2000; Melsen and Verna, 2000).
The biological reaction to an orthodontic force system interacts with the already occurring physiological bone turnover. Our knowledge of the turnover rate of bone in the alveolar process is, however, slight. The sole human histomorphometric study comparing static cortical remodeling in the mandible with that of the iliac crest, which is the most frequent site for bone biopsies, showed that the levels of remodeling parameters were lower in the basal part of the mandible than in bone samples harvested from load‐bearing areas, confirming that remodeling is maintained by function (Verna et al., 1999a). Recently, the site specificity of bone remodeling has been confirmed by Deguchi et al. (2008), who demonstrated faster bone remodeling and faster tooth movement in a dog’s maxilla than in the mandible. This is due not only to a different ratio between cortical and trabecular bone in the two anatomical sites, but also to differences in remodeling rates. At the clinical level, it is advisable to start the orthodontic treatment in the lower jaw to avoid unnecessary prolongation of the treatment time.

Figure 9.5 Alveolar bone on the buccal side of a rat’s tooth after 14 days of orthodontic treatment. On the side facing the periodontal ligament (PDL; arrows), there is staining for alkaline phosphatase (dark blue, right panel) and for acid phosphatase (red, left panel), confirming bone remodeling activities.
Source: Verna and Melsen [2002] / with permission from Libra Ortodonzia.
The rate of tooth movement is influenced by the rate of bone remodeling. Any substance able to interfere with bone remodeling may, in principle, influence the rate of tooth movement. Different substances have been tried experimentally and clinically to increase/decrease the rate of tooth movement, but their use is not recommended in the routine clinical setting. On the other hand, as clinicians we need to know whether substances that may interfere with orthodontic treatment are being taken by our patients. Nonsteroidal anti‐inflammatory drugs, vitamin D, estrogen, and doxycycline are known to interfere with bone remodeling and to decrease the tooth movement rate (Krishnan and Davidovitch, 2006; Bartzela et al., 2009). However, acetaminophen (paracetamol) is an analgesic that does not interfere with tooth movement (Arias and Marquez‐Orozco, 2006). Patients should therefore be instructed preferably to take acetaminophen for relief of pain, so that overall treatment time remains unaffected.
Corticosteroid treatment has an inhibitory effect on bone turnover that subsequently leads to secondary hyperparathyroidism. The effect on tooth movement will therefore depend on the duration of the drug treatment, and is enhanced in patients undergoing long‐term corticosteroid treatment (Kalia et al., 2004). Where treatment is short, the rate of tooth movement may not be affected, but at a tissue level the remodeling process seems to be delayed. In clinical terms, if a patient is under active orthodontic treatment and has to undergo short‐term steroid treatment, such as hay fever therapy, minimal adjustments should be made to the appliance during this period and the interval between appointments may be longer. In treatments of longer duration (about 12 months), the tooth movement rate is increased and the appliance should be checked as usual or more frequently. The effect of steroid treatment on root resorption has also been investigated, with higher rates of root resorption reported in rats administered steroids for a short amount of time (Verna et al., 2006). This is not surprising, since the slower turnover rate will lead to relatively larger amounts of bone covered by osteoid. Since osteoid cannot be resorbed by osteoclasts, the clastic activities are diverted toward the mineralized root surfaces. Once again, in such situations activation of the appliance will not help. On the contrary, less frequent activation will protect against further root damage. In patients with a history of allergy who are undergoing steroid treatment for a short time, it is advisable to postpone treatment until the patient has completed his or her pharmacological treatment and to follow the earlier suggestions in case of a sudden need for steroids during orthodontic treatment. The effect of corticosteroid treatment on tooth movement is also dose dependent, since high dosages induce osteoporosis, which means there is less bone available for resorption, and therefore the rate of tooth movement increases but with a higher degree of relapse (Ashcraft et al., 1992).
Tooth movement can also be accelerated by local mechanisms that increase the remodeling rate without the use of drugs, such as the regional acceleratory phenomenon (RAP) that occurs after fracture healing, as described by Frost (1994; Roblee et al., 2009). RAP is characterized by local increased resorption and formation activities with less mineralized bone formation, which is a sign of quick repair after an injury. RAP normally occurs following orthodontic tooth movement (Verna et al., 1999b) and its intensity is related to the intensity of the damage. Cortical perforations and corticotomies (with or without surgical flap) performed as part of the orthodontic treatment increase the tooth movement rate approximately twofold (Sanjideh et al., 2010) by enhancing the repair process locally, for example in tooth movement immediately following extraction.
In animal experiments the tissue reaction occurring during corticotomy‐assisted tooth movement is characterized by transient bone resorption, followed by the deposition of fibrous tissue after 21 days and by bone after 60 days (Wang et al., 2009). After corticision (a transmucosal corticotomy technique) less hyalinization and more rapid removal of hyalinized tissue has been observed, together with extensive areas of resorption followed by a 3.5‐fold higher accumulated mean apposition area of new bone than the control side (Kim et al., 2009). When cortical perforation is combined with alveolar augmentation, the original alveolar volume and any existing osseous dehiscences may no longer be a limitation for orthodontic tooth movement (Wilcko et al., 2009
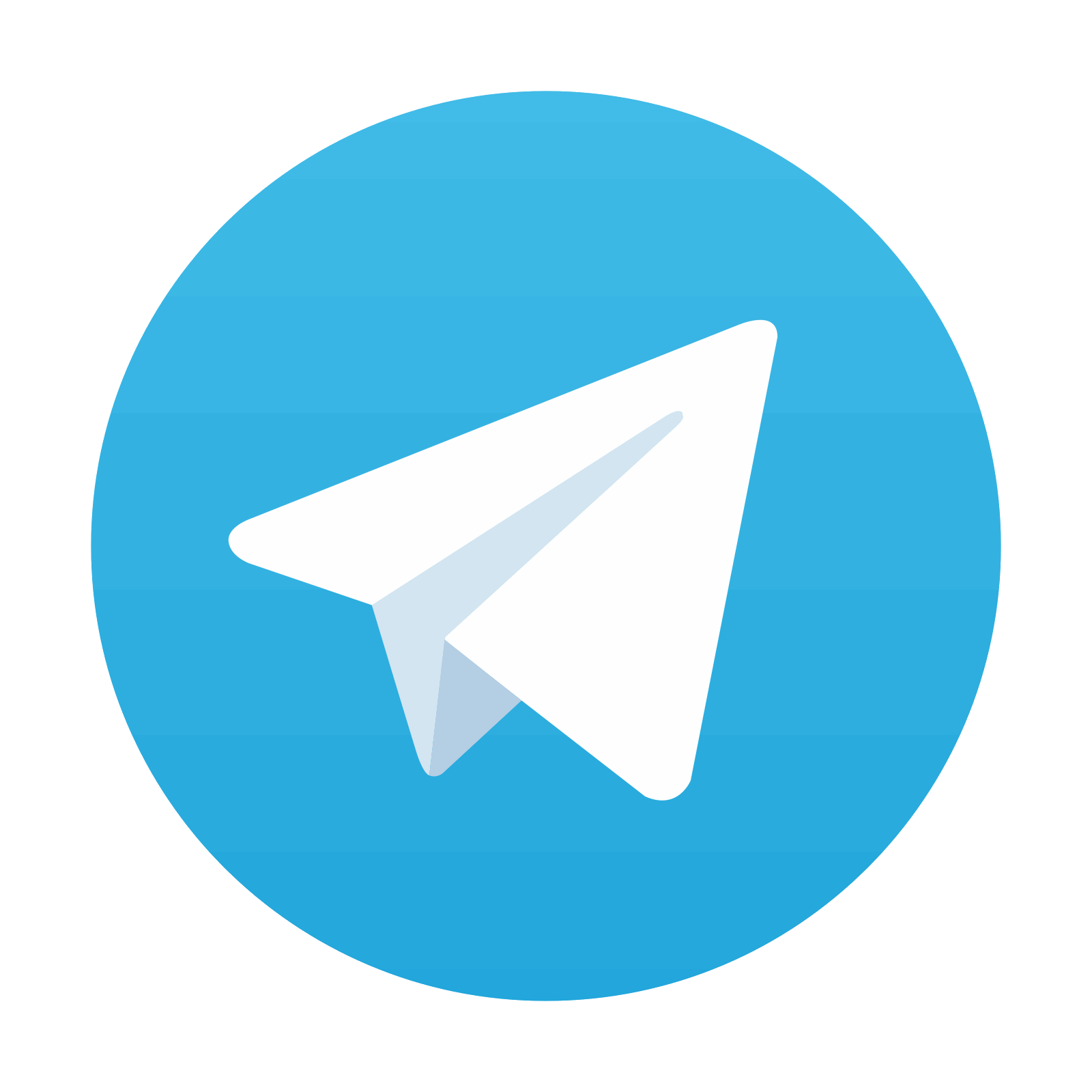
Stay updated, free dental videos. Join our Telegram channel

VIDEdental - Online dental courses
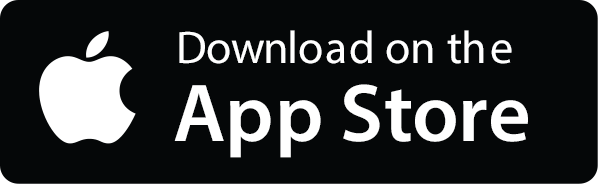
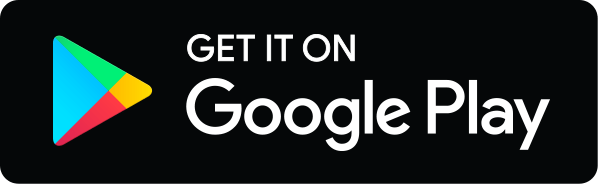