Abstract
Objectives
The purpose of this study was to compare the water sorption/solubility, percent conversion and microtensile bond strength of three single-step self-etching adhesives with those of a two-step self-etching primer adhesive system.
Methods
Solvent evaporation from the adhesives was determined gravimetrically. After removal of volatile solvents, the resins were cast into disks and polymerized. One-half of the disks were incubated in water while the other half were incubated in hexadecane. Repeated measurements of water sorption were made for 10 days followed by drying for 2.5 days to a constant weight. Percent conversion was done using FTIR spectroscopy. Microtensile bond strengths were measured 24 h after bonding.
Results
All of the adhesives lost 20–30% of their weight after 4 min of forced air except for Fluorobond II which lost no weight. All resins stored in water exhibited a time-dependent increase in water sorption and solubility. The resins stored in hexadecane showed very low sorption and solubility. Water sorption was highest for Absolute 2 (20.7%), intermediate for Fluorobond Shake One (10.2%) and lowest for Clearfil 3 S (8.9%) and Fluorobond II (7.5%). Percent conversions ranged from a low of 68.3% for Absolute 2 to a high of 87.4% for Clearfil 3 S. The two-step self-etching primer adhesive (Fluorobond II) gave the lowest water sorption and lowest solubility of any of the tested adhesives. SEM observations of resin disks incubated in hexadecane looked similar to unincubated controls. Incubating resin disks in artificial saliva covered the surfaces of the resins with mineral crystallites.
Significance
Single bottle self-etching adhesives show higher water sorption/solubilities than two-step self-etching adhesives. The former products would not be expected to function as well as the latter products.
1
Introduction
In 2003, Tay and Pashley asked the question “have dentin adhesives become too hydrophilic?” It has become clear that water left on acid-etched dentin surfaces in the wet bonding technique is necessary to keep collagen fibrils collapsing , but can water also causes phase changes when dimethacrylates encounter too much water . Even in self-etching adhesives that are applied to dry smear layer-covered dentin, 10–30 wt% water is added to the hydrophilic formulations to ionize the carboxylic or phosphate methacrylates and to solubilize calcium and phosphate ions liberated from the action of self-etching adhesives with dentin. If this water is not evaporated, it will dilute the comonomers, and may interfere with bonding and lower the mechanical properties of the resin . Manufacturers seem to concentrate on how rapidly their materials can create a bond rather than determining the optimum application time and evaporation time for maximum bond strength. It has been reported that reduction in mechanical strength and modulus of elasticity are associated with increasing hydrophilicity of the copolymer blends after water storage. This could explain why resin–dentin bonds made with hydrophilic resin monomers significantly degrade over time in vitro and in vivo .
Water sorption within polymer matrices created by contemporary hydrophilic dentin adhesives is not always uniform. Using ammoniacal silver nitrate to trace the distribution of absorbed water, Tay et al. have shown both uniform and non-uniform water uptake into commercial adhesive resins . Uniform absorption was seen as isolated individual silver grains, while the non-uniform water uptake resulted in the formation of liner, branched, water-filled channels . When dentin bonded with adhesive resins was stored in water for 12 months, the distribution of absorbed water changed dramatically . Clearly, water sorption by dental resins and the hybrid layer is more complex than expected .
All-in-one adhesives and self-etching primers are intrinsically hydrophilic owing to the presence of acidic, highly polar functional groups substituted on methacrylates, and the presence of water and ethanol solvents. They rapidly absorb water, which results in polymer swelling, plasticizing and weakening of the polymer network . Water absorption into polymers is assumed to be directly related to the hydrophilicity of the polymers .
The water sorption and solubility of newly developed adhesives needs to be fully investigated. The null hypothesis tested was that none of the adhesive resins tested will have different water sorption/solubility values.
2
Materials and methods
2.1
Evaporation of dentin bonding resin components by storage in room temperature and by air-drying
Forty microliters of unpolymerized adhesive were placed on a tared Teflon slide. For two-step adhesive, FB II, equal volumes of primer and adhesive were rapidly (5 s) mixed together and placed on a tared slide. The Teflon slide was weighted before and immediately after depositing the drop of bonding resin, enabling the weight of the bonding resin (A) from the difference of the two weights to be calculated (0-min storage time). To allow the volatile dentin bonding components to spontaneously evaporate, the plastic-plate with the unpolymerized adhesive mixture was stored in the dark for 60 min at room temperature (25 °C). When an air-stream was used to evaporate solvents, a 3-way air-water syringe was clamped 15 cm from the adhesive and the control button depressed half-way to produce an air flow of 20 L/min for 30 min in the dark. Five specimens were prepared per adhesive. The weight of plastic-plate with the bonding resin was successively remeasured every minute for the first 5 min, every 5 min from 10 to 30 min and every 10 min for the rest of the time. The weight of residual adhesives at each time (B) was determined by subtracting the weight of the plastic-plate.
The degree of spontaneous evaporation of volatile solvents ( Ep in wt%) at room temperature was followed for 60 min. The rate of evaporation of volatile solvents in response to an air-stream was calculated as follows:
with A the initial weight of the adhesive mixture and B the weight of the mixture after each storage time.
2.2
Water sorption/solubility
After the time required for spontaneous or air-stream-induced solvent evaporation was known, the commercial comonomers/solvent mixtures were placed in a circular well made in Teflon to form disks 10.0 ± 0.1 mm in diameter and 1.0 ± 0.02 mm thick. While in the dark, the Teflon well containing the solvated comonomers was exposed to the same air-stream used to evaporate the solvents in the previous section, for 30 min to insure that all volatile solvents had been removed prior to light curing. The surface of the comonomers was covered with a glass cover slip to exclude atmospheric oxygen, forming a flat surface, and the resin was light-cured for 30 s using a dental curing light (Morita, Kyoto, Japan) operated at 600 mW/cm 2 , with the tip held 1 mm from cover slip. After removing the disk from the mold, a similar light exposure was applied to the lower disk surface. Specimen dimensions to the nearest 0.01 mm were measured using a digital micrometer. Ten resin disks were made for each of the commercial resins. They were dry polished to a thickness of 0.50 ± 0.02 mm.
Water sorption was measured following the method outlined in ISO 4049 (12-1998). However, disk diameters were 10 mm and 0.5 mm in thickness, to match the dimensions of specimen molds. Immediately after polymerization, the specimens were placed in desiccators and transferred to a pre-conditioning oven at 37 °C. The specimens were repeatedly weighed after 24 h intervals until a constant mass (m 1 ) was obtained (i.e. variation was less than 0.2 mg in any 24 h period). Thickness and diameter of the specimens were measured using a digital caliper, rounded to the nearest 0.01 mm, and these measurements were used to calculate the volume ( V ) of each specimen (in mm 3 ). The resin disks were then individually placed in sealed glass vials containing 10 mL of distilled water (pH 7.2) at 37 °C. After fixed time intervals of 1, 2, 3, 4, 5, 6, 7 and 10 days of storage, the vials were removed from the oven and left at room temperature for 30 min. The specimens were washed in running water, gently wiped with a soft absorbent paper, weighed in an analytical balance ( m 2 ) and returned to the vials containing 10 mL of fresh distilled water. Following the 10 days of storage, the specimens were dried inside a desiccator containing fresh silica gel and weighed daily for 2.5 days until a constant mass ( m 3 ) was obtained (as previously described). The initial mass determined after the first desiccation process ( m 1 ) was used to calculate the change in mass after each fixed time interval, during the 10 days of storage in water calculated as the difference in dry mass before immersion and after reaching the water sorption plateau, following drying in a sealed chamber filled with anhydrous calcium sulfate . Disk volume was determined by measuring diameter and thickness before and after water exposure. Dry weight measurements were followed daily for 10 days. The values (%) for water sorption (WS) and solubility (SL) were calculated as <SPAN role=presentation tabIndex=0 id=MathJax-Element-2-Frame class=MathJax style="POSITION: relative" data-mathml='WS=M2−M3/V,SL=M1−M3/V,’>WS=M2−M3/V,SL=M1−M3/V,WS=M2−M3/V,SL=M1−M3/V, W S = M 2 − M 3 / V , S L = M 1 − M 3 / V , where M 1 is the initial dry constant mass (mg) before water immersion; M 2 is the mass (mg) after water immersion; M 3 is the mass (mg) after drying specimens that had reached their maximum water sorption and V is the specimens volume in mm 3 . Net water uptake was calculated as the sum of water sorption and solubility .
Resin disks were also immersed in hexadecane as an example of a water-free pure oil with a viscosity similar to water. At each time period, resin disks were removed from the hexadecane and blotted dry of excess hexadecane before weighing. These specimens were run in parallel with the water immersed specimens as controls.
2.3
Monomer conversion
To ascertain that the increase in water sorption of the more hydrophilic resin blends was not caused by their lower extent of cure, the monomer conversion of the commercial resins was measured using infrared spectroscopy, according to the method of Rueggeberg et al. . Briefly, 25 μL of solvated blends were placed in a small tared vessel on an analytical balance. After obtaining an initial weight, the vessel was removed from the balance and evaporated with an air-stream (0.5 L/s) for 10 min or until there was no further weight loss. Then 5 μL of the solvent-free blend was placed in a 6-mm hole was punched in a piece of single side Scotchtape, that was placed directly over the 2 × 2 mm diamond crystal of a horizontal attenuated total reflectance unit (Golden Gate-SPECAC, Inc., Woodstock, GA). As the tape was 50 ± 1 μm thick, the hole provided a convenient “well” into which was placed 5 μL of each evaporated resin blends. The fluid was covered with a thin Mylar film which, in turn, was covered by a glass slide. The resin was cured as described above, using a 120-s exposure. Multiple scans were made before and after light exposure at 2 cm −1 resolution between 1680 and 1550 cm −1 at a rate of one scan/s for 305 s, using a Fourier Transform Infrared spectrophotometer (IR Prestige-21, Shimazu, Tokyo, Japan). The degree of conversion was calculated using changes in the molar ratios (represented as peak absorbance height) of aliphatic (1636 cm −1 )/aromatic (1608 cm −1 ) carbon double bonds on the cured ( C ) and uncured ( U ) states. Conversion was calculated by using the following equation:
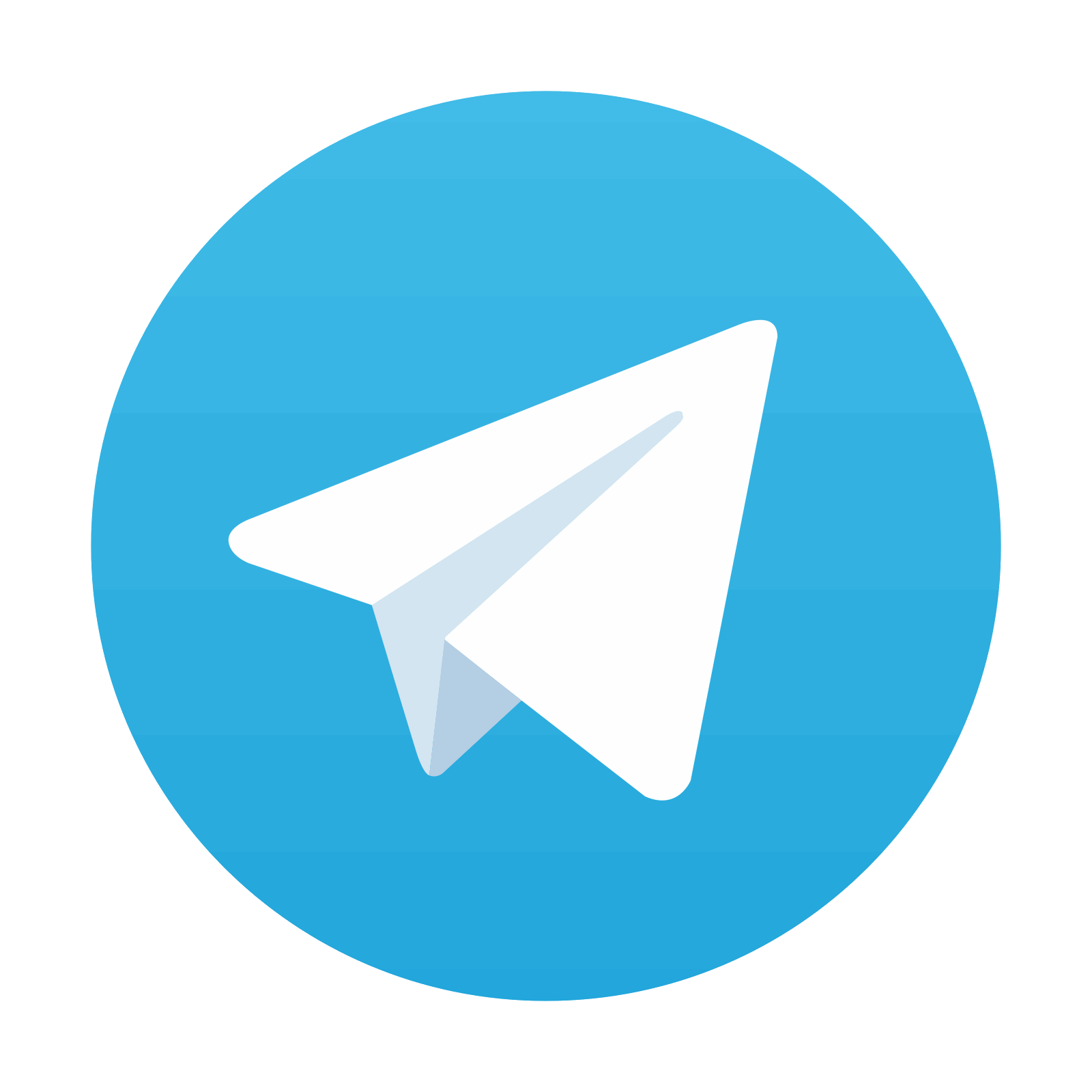
Stay updated, free dental videos. Join our Telegram channel

VIDEdental - Online dental courses
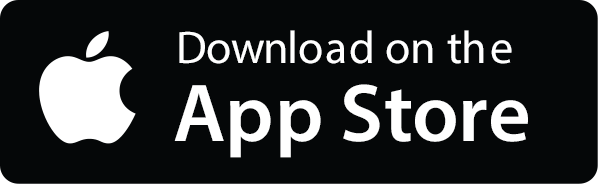
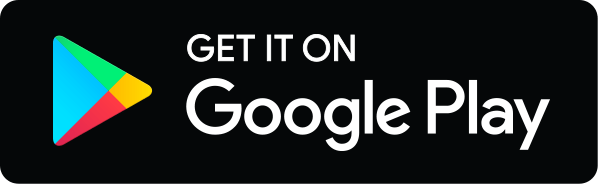