Abstract
Objectives
The water-associated attributes of resin-dentin interfaces created by contemporary adhesives are important determinants of bond integrity and stability. In the present work, these attributes were estimated from the perspectives of causality, to examine the behavior of the first and most-recently launched versions of universal adhesives when applied in either the etch-and-rinse mode or the self-etch mode.
Methods
The immediate cause of interfacial permeability and the time-dependent cause of water sorption were investigated in conjunction with the intermediate effect of interface degradation and the more long-term effect of loss of mechanical strength, before and after thermomechanical cycling. The results were compared with control etch-and-rinse and self-etch adhesives.
Results
Although the introduction of this new class of universal adhesives has brought forth significant changes to the dental adhesion arena, including more application options, reduced bonding armamentarium and increased user friendliness, the water-associated attributes that are critical for making resin-dentin bonds more durable to environmental challenges and less susceptible to degradation have remained unchanged at large, when compared with benchmarks established by former classes of adhesives.
Conclusion
It appears that the current trend of adhesive development has brought forth significant changes but lacks the vigor that demarcates progress and technological sublimity.
Clinical significance
The advent of the user friendly universal adhesives has brought forth significant changes to the dental adhesion arena. However, the elements that are critical for making resin-dentin bonds more durable to environmental challenges and less susceptible to degradation have remained unchanged at large.
1
Introduction
The call for bonding synthetic materials to tooth structures has saturated the fabrics of contemporary restorative dentistry. Indeed, the boundary between the art of dental esthetic illusion and the practice of dental adhesion is becoming increasingly indiscriminate. As such, there is an ongoing trend among manufacturers to simplify single-bottle dentin bonding technology to satisfy clinicians’ demand for adhesive procedures that are faster, less technique-sensitive and more user-friendly . Recently introduced single-bottle adhesives also provide clinicians with the option of selecting the most optimal bonding mode, dictated by different clinical scenarios, for bonding different restorative materials to tooth structures. This clan of multi-mode universal adhesives is designed to bond to tooth structures via the etch-and-rinse mode, the self-etch mode or the selective-etch mode using the same bottle of adhesive solution . Some manufacturers also incorporate 10-methacryloyloxydecyl dihydrogen phosphate (10-MDP) into their adhesives as a self-etching resin monomer and as a primer for bonding zirconia-base ceramics .
A recent systematic review of universal adhesives surmised that acid-etching does not adversely affect the dentin bond strength of these adhesives . However, the pH values of the adhesive agents and the smear layer thickness of bonding substrates significantly affect the ability of using these adhesives in the self-etch mode to dissolve the smear layer and partially demineralize the underlying intact dentin . Residual water from the dentin surface and/or the adhesive solvent may compromise the performance of universal adhesives . The technique of universal adhesive application is paramount for predictable outcomes associated with these adhesives .
Introduction of the universal bonding concept, while appealing to clinicians, should not compromise adhesive bonding effectiveness and the durability of resin-dentin bonds . Ideally, establishing a stable and durable resin-dentin interface with excellent sustainability should be the fervor for the creation of the next generation of dentin adhesives, which may be interpreted as “low-maintenance” in consumer culture’s jargon . Scotchbond Universal (3 M ESPE, St. Paul, MN, USA) was the first 10-MDP containing universal adhesive introduced into the market. Clearfil Universal Bond Quick (Kuraray Noritake Dental Inc., Tokyo, Japan) represents the most recently-launched 10-MDP containing universal adhesive.
In the present study, the notion that the water-associated attributes that adversely affect dentin bonding , identified in previous generation of adhesives, have been resolved with the advent of universal adhesives was challenged by examining the ultrastructure and permeability characteristics of resin-dentin interfaces bonded in the etch-and-rinse mode and the self-etch mode. The potential degradability of adhesive interfaces by endogenous enzymes present in mineralized dentin, and the adhesive bond strengths to dentin were examined before and after thermomechanical cycling. The water sorption characteristics of the polymerized adhesive resins were also investigated. Although many universal adhesives are commercially available, it is impractical to examine them all in a single piece of work. Hence the water-associated attributes of the first and the most recently-launched universal adhesives were examined and compared with a commercially available etch-and-rinse adhesive (OptiBond Solo Plus, Kerr Corporation, Orange, CA, USA) and a commercially available self-etch adhesive (Clearfil SE Bond 2, Kuraray Noritake Dental Inc., Tokyo, Japan). The null hypotheses tested were: 1) adhesive etching mode has no consequence on the intrinsic water permeability of the resin-dentin interfaces; 2) thermomechanical cycling has no effect on the gelatinolytic activity of resin-dentin interfaces created by either the etch-and-rinse or the self-etch technique; 3) there is no difference in the microtensile bond strength of the four adhesives bonded to dentin before and after thermomechanical cycling; and 4) there is no difference among the four adhesive resin blends on water sorption.
2
Materials and methods
2.1
Crown segments
Two hundred and twenty-two extracted non-carious human third molars were collected based on a protocol approved by the Human Assurance Committee of the Augusta University, with informed consent obtained from the donating subjects with respect to the use of human tissues. The extracted teeth are stored in 0.9% (w/v) NaCl containing 0.02% sodium azide at 4 °C for no longer than one month. The roots of the teeth were removed perpendicular to the longitudinal axis of the tooth, at 3.5 mm below the cement-enamel junction (CEJ), using a water-cooled low-speed diamond saw (Isomet, Buehler Ltd, Lake Bluff, IL, USA). For each tooth, a second parallel cut was used to remove the occlusal enamel and superficial dentin, creating a flat bonding surface consisting of mid-coronal dentin. A standard smear layer was manually created by wet-sanding the occlusal surfaces of crown segments with 320-grit silicon carbide paper under water irrigation for 1 min.
2.2
Bonding procedures
The teeth were randomly assigned to six groups of twenty-nine teeth each according to the adhesive systems and bonding techniques. The compositions of the four adhesives are summarized in Table 1 . The two universal adhesives, Scotchbond Universal (SUB) and Clearfil Universal Bond Quick (CUBQ) were used in either the etch-and-rinse mode for the self-etch mode. OptiBond Solo Plus (OSP) was used in the etch-and-rinse mode only while Clearfil SE Bond 2 (CSEB) was used in the self-etch mode only. For the etch-and-rinse bonding mode, a 35% phosphoric acid etchant (Select Hv Etch, Bisco, Inc., Schaumburg, IL, USA) was used for etching all teeth for 15 s. A separate etchant was not used for bonding in the self-etch bonding mode. For both bonding modes, the adhesives were applied according to the manufacturer’s instructions ( Table 1 ). After light-curing of the adhesive, resin composite build-ups were constructed in four 1-mm thick increments using a hybrid resin composite (except for specimens designated for transmission electron microscopy, as reported in the corresponding section). Each increment was light-cured for 20 s using a light-emitting diode (440–480 nm range) curing unit with an output intensity of 1200 mW/cm 2 .
Adhesive | pH | Composition | Self-etch mode | Etch-and-rinse mode |
---|---|---|---|---|
Scotchbond Universal (3 M ESPE, St, Paul, MN, USA) | 2.7 | 10-MDP, dimethacrylates, HEMA, Vitrebond copolymer, filler, ethanol, water, initiator, silane | 1. Apply the adhesive to thoroughly wet all the tooth surfaces and rub it in for 20 s. | 1. Apply phosphoric acid etching gel, leave for 15 s, rinse and blot-dry with lint-free paper. |
2. Gently air-dry the adhesive for approximately 5 s for the solvent to evaporate. | 2. Apply adhesive as for the self-etch mode. | |||
3.Light-cure for 10 s. | ||||
Clearfil Universal Bond Quick (Kuraray Noritake Dental Inc., Tokyo, Japan) | 2.3 | 10-MDP, BisGMA, HEMA, hydrophilic amide resin monomers, colloidal silica, silane coupling agent, NaF, CQ, ethanol, water | 1. Apply generous amounts of adhesive to thoroughly wet all the tooth surfaces with a rubbing motion. | 1. Apply phosphoric acid etching gel, leave for 15 s, rinse and blot-dry with lint-free paper. |
2. Dry by blowing mild air for more than 5 s until the adhesive shows no movement. Use a vacuum aspirator to prevent the adhesive from scattering. | 2. Apply adhesive as for the self-etch mode. | |||
3. Light-cure for 10 s. | ||||
Clearfil SE Bond 2 (Kuraray Noritake Dental Inc., Tokyo, Japan) | Primer: pH 2.0; Bond: pH 2.4. | Primer: 10-MDP, HEMA, hydrophilic aliphatic dimethacrylate, CQ, water Bond: 10-MDP, BisGMA, HEMA, hydrophobic aliphatic dimethacrylate, CQ, initiators, accelerators, silanated colloidal silica | 1. Apply Primer to thoroughly wet all the tooth surfaces and leave it in for 20 s (no scrubbing is required). | – |
2. Gently air-dry the adhesive for approximately 5 s for the solvent to evaporate. 3. Apply Bond, and gently air-dry it to make a uniform bond film. | ||||
3. Light-cure for 10 s. | ||||
OptiBond Solo Plus (Kerr Corp., Orange, CA, USA) | 2.2 | Ethyl alcohol, alkyl dimethacrylate resins, barium aluminoborosilicate glass, SiO 2 , sodium hexafluorosilicate | – | 1. Apply phosphoric acid etching gel, leave for 15 s, rinse and blot-dry with lint-free paper. |
2. Apply adhesive to thoroughly wet all the tooth surfaces with applicator tip for 15 s, using light brushing motion. | ||||
3. Gently air-dry the adhesive for approximately 3 s for the solvent to evaporate. | ||||
4. Light-cure for 10 s. |
2.3
Transmission electron microscopy
Two teeth from each of the six groups were used for transmission electron microscopy (TEM) of the resin-dentin interface. After bonding with the respective adhesive, a 2-mm thick layer of microfilled resin composite was coupled to the polymerized adhesive to facilitate ultramicrotomy. Each bonded specimen was sectioned longitudinally through the center of the tooth to produce a 1-mm thick slab containing the resin-dentin interface. The specimens were fixed with Karnovsky’s fixative (2.5 wt% glutaraldehyde and 2% paraformaldehyde in 0.1 mol/L cacodylate buffer; pH, 7.3) for 8 h, and post-fixed in 1% osmium tetroxide for 1 h. The fixed specimens were dehydrated in an ascending ethanol series (50–100%), immersed in propylene oxide as a transition medium, and embedded in pure epoxy resin. Ninety nanometer-thick sections were prepared using an ultramicrotome. The sections were examined unstained using a JEM-1230 TEM (JEOL, Tokyo, Japan) at 110 kV.
2.4
Water permeability of bonded interfaces
Five teeth in each adhesive group were designated for permeability examination of the bonded interfaces. A pair of calipers was used to control the remaining dentin thickness of each tooth to 2.5 ± 0.1 mm from the deepest pulpal horn. A double fluorescence technique was employed to enable the adhesive and the water that permeated the resin-dentin interface to be identified simultaneously with confocal laser scanning microscopy (CLSM). Three drops of each adhesive system was mixed, respectively, with 1 μL of a yellow fluorescent dye (Alexa Fluor™ 532, excitation/emission: 532/553 nm; ThermoFisher Scientific, Waltham, MA, USA) to produce a homogeneous mixture. The fluorescent adhesives were kept in the dark until use. Each crown segment to be bonded was attached to a perforated Plexiglass block with cyanoacrylate blue. The assembly was connected via an 18-gauge stainless steel tube to a polyethylene tubing. The latter was attached to a column of a blue fluorescent dye-containing water (Alexa Fluor™ 405, excitation/emission: 401/421 nm; ThermoFisher Scientific) oriented 20 cm above the Plexiglass block to simulate the delivery of physiologic intrapulpal pressure ( Fig. 2 a). Intrapulpal pressure was applied to the prepared dentin surface during the adhesive procedures and resin composite build-up. The set-up was left in the dark for 4 h after completion of the bonding and restorative procedures to enable water to continue permeate the resin-dentin interface.
After completion of the water permeation protocol, each bond crown segment was removed from the Plexiglass block and sectioned longitudinally through the center of the tooth to retrieve 1-mm thick slab containing the water-perfused resin-dentin interface. Each slab was fixed to a glass slide with Loctite Super Glue (Henkel, Rocky Hill, CT) and serially polished with 600- and 1200-grit wet silicon carbide papers to obtain a ∼50 μm-thick section. Final polishing was achieved using 4000-grit wet silicon carbide paper (MicroCut S-P400; Buehler Ltd.) for 5 min to obtain a glossy surface for CLSM examination. Microscopy was performed by an independent observer who was unaware of the treatment applied to the crown segments. Examination was performed using a two-photon CLSM (LSM 710 NLO, Carl Zeiss, Oberkochen, Germany). Three 85 μm thick optical section series were acquired per slab from different focal planes (N = 15 images). One image was taken from the center of each slab, and the other two images were taken from each side where the remaining dentin thickness was the thinnest from the dentin surface. The images were stacked and processed with the ZEN 2010 software (Carl Zeiss). Blue fluorescence within and above the hybrid layer was quantified using Image J (National Institutes of Health, Bethesda, MD) to represent the relative permeability of the respective resin-dentin interface.
2.5
In-situ zymography
Although pre-forms of dentin matrix-bound endogenous proteases are activated during demineralization of the collagen fibrils and adhesive application, degradation of the denuded collagen fibrils within hybrid layers requires specimen aging for 9–12 months before visible evidence of collagen degradation could be identified by TEM because of the highly cross-linked nature of dentin collagen . In-situ zymography is a laboratory technique that enables rapid localization of matrix metalloproteinases (MMP) activity in histological sections . The technique utilizes gelatin conjugated to quenched fluorescein as the substrate for identifying the location and activities of activated endogenous enzymes within the resin-dentin interfaces . Hence, in-situ zymography may be used as an expedited, quantifiable method for comparing the relative degradation potential of resin-dentin interfaces, without relying on actual degradation of the resin-sparse, water-rich denuded collagen fibrils. In the present work, a double fluorescence technique was employed to enable the locations of the dentin adhesive and the activated endogenous enzymes to be identified simultaneously with CLSM ( Fig. 3 a). For the preparation of fluorescent adhesives, one grain of tetramethylrhodamine B isothiocyanate (excitation/emission: 540/625 nm; MilliporeSigma, St. Louis, MO, USA) was dissolved in 3 drops of each adhesive.
Ten teeth in each adhesive group were designated for in-situ zymography of the resin-dentin interface. The dyed adhesives were used for dentin bonding, as previous described. The ten bonded teeth were divided into two subgroups. Five teeth were stored in deionized water at 37 °C for 24 h. The other five teeth were subjected to thermomechanical challenge, using 10,000 thermal cycles (10 °C for one min, 25 °C for one min and 55 °C for one min) and 240,000 mechanical cycles, corresponding to one year of intraoral functioning . Simulated aging was performed in a thermomechanical wear system (Model E R -37000; Erios, São Paulo, SP, Brazil).
A 1-mm thick slab containing the resin-dentin interface was retrieved from the center of each bonded crown segment. The slab was affixed to a glass slide and polished to ∼50 μm-thick, as described in the previous section. In-situ zymography was performed with the EnzChek™ Gelatinase/Collagenase Assay Kit (E-12055, Molecular Probes, Eugene, OR, USA). Briefly, 50 μL of the quenched fluorescein-conjugated gelatin mixture was placed on top of each slab and protected with a cover slip. Slides were light-protected and incubated in a 100% relative humidity chamber at 37 °C for 48 h. Hydrolysis of the quenched fluorescein-conjugated gelatin releases fluorescein (excitation/emission: 458/540 nm). Microscopy was performed by an independent observer who was unaware of the treatment applied to the crown segments. Green fluorescence was imaged together with the red fluorescence released by the adhesive using different channels of the two-photon CLSM. For each of the 5 slabs in each subgroup (with or without thermomechanical cycling), three image series were taken for gelatinolytic activity evaluation (N = 15). One series was taken from the center of each slab, and the other two series were taken from each side where the remaining dentin thickness was the thinnest from the dentin surface. For each area, 20 optical sections (350 nm thick) were acquired from different focal planes. Fluorescence intensity emitted by degraded fluorescent peptides was quantified from the stacked image of each series using the Image J software. Gelatinolytic activity was expressed as the percentage of the resin-dentin interface that exhibited green fluorescence.
2.6
Microtensile bond strength
Twenty teeth in each adhesive group were designated for bond strength testing, using the non-trimmed version of the microtensile bond testing method . After bonding with the undyed adhesives and restoration with hybrid resin composite, 10 teeth per group were stored in deionized water at 37 °C for 24 h and 10 teeth were thermomechanically cycled in the manner described in the previous section. Each bonded crown segment were sectioned in the x and y directions across the resin-dentin interface to obtain beams with cross-sectional areas of approximately 0.9 mm × 0.9 mm. The four longest beams were obtained from the two central slabs of each bonded crown segment were used for bond strength testing. Each was secured with cyanoacrylate glue (Zapit; Dental Ventures of America, Corona, CA, USA) to a testing jig and stressed to failure under tension in a universal testing machine (Vitrodyne V1000; Liveco Inc., Burlington, VT, USA) at a cross-head speed of 1 mm/min. The tensile load at failure was recorded and divided by the measured cross-sectioned area of each beam to yield the tensile bond strength in megaPascals (MPa). The mean bond strength value derived from the 4 beams of each tooth was used to represent the microtensile bond strength of that particular tooth. Data analysis was subsequently performed using the tooth as the statistical unit (N = 10 teeth).
2.7
Water sorption
Six specimen disks (5 ± 0.1 mm diameter, 1 ± 0.1 mm thick) were fabricated for each adhesive group. The adhesives were dispensed into light-protected vials and placed in a vacuum chamber in the dark for 72 h to remove the adhesive solvent. The neat resin was dispensed into a Teflon split ring mold placed between two Mylar sheets. The solvent-free resin was first light-cured over the center of the disk for 40 s, and then over eight peripheral overlapping sections for 20 s each. After storage in a desiccator with silica gel at 37 °C for 24 h, the specimens were weighed in an electronic analytical balance with a reproducibility of 0.01 mg (Model XP 205PR; Mettler Toledo, Columbus, OH, USA) until a constant mass (M 1 ) was obtained (N = 6). The diameter and the thickness of the specimens were measured at four points using a pair of digital calipers for calculation of disk volume (V).
Each resin disk was stored separately in a Parafilm-sealed glass vial containing 20 mL of deionized water at room temperature for 24 days. During sorption, the specimens were weighted every 3 h during the first day, every 6 h during the second day, every 12 h during the third and fourth days and then daily thereafter. The disks were weighed until a constant mass was obtained (M 2 ) for the four adhesives at the end of the 24-day period. Percent mass gain or loss at equilibrium was calculated for each resin disk using the equation (M 2 -M 1 )/V*100% .
2.8
Statistical analyses
Interval data generated from the experiments were examined for their normality (Shapiro-Wilk test) and equal variance assumptions (modified Levene test) prior to the adoption of parametric statistical methods. Statistical significance for all analyses was pre-set set at α = 0.05.
Data for relative permeability of the resin-dentin interfaces were non-linearly transformed to satisfy the equal variance assumption prior to analysis with one-factor analysis of variance and post-hoc pairwise comparisons with the Holm-Šidák statistic. Data generated from in-situ zymography were analyzed with a two-factor analysis of variance design to examine the effects of “adhesive/application mode” and “thermomechanical cycling”, and the interaction of those two factors on percent gelatinolytic activity. The Holm-Šidák statistic was employed for post-hoc pairwise comparisons. The original data sets generated from microtensile testing did not conform to the requirements for the use of parametric statistical methods. Hence, the data were nonlinearly transformed prior to analysis with two-factor analysis of variance to examine the effects of “adhesive/application mode” and “thermomechanical cycling”, and the interaction of those two factors on dentin bond strength. The Holm-Šidák statistic was employed for post-hoc pairwise comparisons. Data of maximum water sorption in the four adhesive resin groups were analyzed with one-factor analysis of variance followed by Holm-Šidák pairwise comparisons. Because mass loss after water sorption was identified in two adhesive resin groups only, the maximum solubility data were analyzed with Student’s t -test.
2
Materials and methods
2.1
Crown segments
Two hundred and twenty-two extracted non-carious human third molars were collected based on a protocol approved by the Human Assurance Committee of the Augusta University, with informed consent obtained from the donating subjects with respect to the use of human tissues. The extracted teeth are stored in 0.9% (w/v) NaCl containing 0.02% sodium azide at 4 °C for no longer than one month. The roots of the teeth were removed perpendicular to the longitudinal axis of the tooth, at 3.5 mm below the cement-enamel junction (CEJ), using a water-cooled low-speed diamond saw (Isomet, Buehler Ltd, Lake Bluff, IL, USA). For each tooth, a second parallel cut was used to remove the occlusal enamel and superficial dentin, creating a flat bonding surface consisting of mid-coronal dentin. A standard smear layer was manually created by wet-sanding the occlusal surfaces of crown segments with 320-grit silicon carbide paper under water irrigation for 1 min.
2.2
Bonding procedures
The teeth were randomly assigned to six groups of twenty-nine teeth each according to the adhesive systems and bonding techniques. The compositions of the four adhesives are summarized in Table 1 . The two universal adhesives, Scotchbond Universal (SUB) and Clearfil Universal Bond Quick (CUBQ) were used in either the etch-and-rinse mode for the self-etch mode. OptiBond Solo Plus (OSP) was used in the etch-and-rinse mode only while Clearfil SE Bond 2 (CSEB) was used in the self-etch mode only. For the etch-and-rinse bonding mode, a 35% phosphoric acid etchant (Select Hv Etch, Bisco, Inc., Schaumburg, IL, USA) was used for etching all teeth for 15 s. A separate etchant was not used for bonding in the self-etch bonding mode. For both bonding modes, the adhesives were applied according to the manufacturer’s instructions ( Table 1 ). After light-curing of the adhesive, resin composite build-ups were constructed in four 1-mm thick increments using a hybrid resin composite (except for specimens designated for transmission electron microscopy, as reported in the corresponding section). Each increment was light-cured for 20 s using a light-emitting diode (440–480 nm range) curing unit with an output intensity of 1200 mW/cm 2 .
Adhesive | pH | Composition | Self-etch mode | Etch-and-rinse mode |
---|---|---|---|---|
Scotchbond Universal (3 M ESPE, St, Paul, MN, USA) | 2.7 | 10-MDP, dimethacrylates, HEMA, Vitrebond copolymer, filler, ethanol, water, initiator, silane | 1. Apply the adhesive to thoroughly wet all the tooth surfaces and rub it in for 20 s. | 1. Apply phosphoric acid etching gel, leave for 15 s, rinse and blot-dry with lint-free paper. |
2. Gently air-dry the adhesive for approximately 5 s for the solvent to evaporate. | 2. Apply adhesive as for the self-etch mode. | |||
3.Light-cure for 10 s. | ||||
Clearfil Universal Bond Quick (Kuraray Noritake Dental Inc., Tokyo, Japan) | 2.3 | 10-MDP, BisGMA, HEMA, hydrophilic amide resin monomers, colloidal silica, silane coupling agent, NaF, CQ, ethanol, water | 1. Apply generous amounts of adhesive to thoroughly wet all the tooth surfaces with a rubbing motion. | 1. Apply phosphoric acid etching gel, leave for 15 s, rinse and blot-dry with lint-free paper. |
2. Dry by blowing mild air for more than 5 s until the adhesive shows no movement. Use a vacuum aspirator to prevent the adhesive from scattering. | 2. Apply adhesive as for the self-etch mode. | |||
3. Light-cure for 10 s. | ||||
Clearfil SE Bond 2 (Kuraray Noritake Dental Inc., Tokyo, Japan) | Primer: pH 2.0; Bond: pH 2.4. | Primer: 10-MDP, HEMA, hydrophilic aliphatic dimethacrylate, CQ, water Bond: 10-MDP, BisGMA, HEMA, hydrophobic aliphatic dimethacrylate, CQ, initiators, accelerators, silanated colloidal silica | 1. Apply Primer to thoroughly wet all the tooth surfaces and leave it in for 20 s (no scrubbing is required). | – |
2. Gently air-dry the adhesive for approximately 5 s for the solvent to evaporate. 3. Apply Bond, and gently air-dry it to make a uniform bond film. | ||||
3. Light-cure for 10 s. | ||||
OptiBond Solo Plus (Kerr Corp., Orange, CA, USA) | 2.2 | Ethyl alcohol, alkyl dimethacrylate resins, barium aluminoborosilicate glass, SiO 2 , sodium hexafluorosilicate | – | 1. Apply phosphoric acid etching gel, leave for 15 s, rinse and blot-dry with lint-free paper. |
2. Apply adhesive to thoroughly wet all the tooth surfaces with applicator tip for 15 s, using light brushing motion. | ||||
3. Gently air-dry the adhesive for approximately 3 s for the solvent to evaporate. | ||||
4. Light-cure for 10 s. |
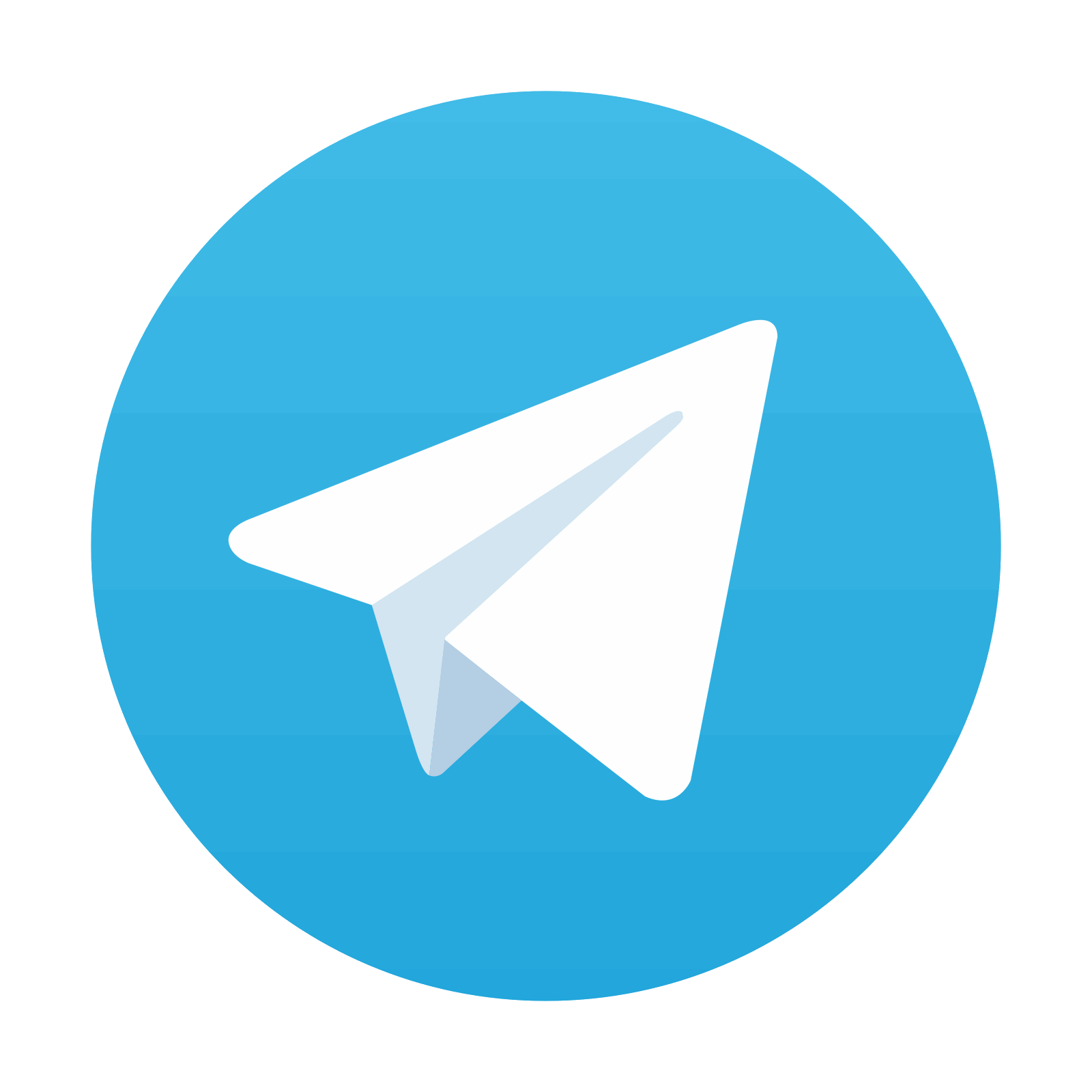
Stay updated, free dental videos. Join our Telegram channel

VIDEdental - Online dental courses
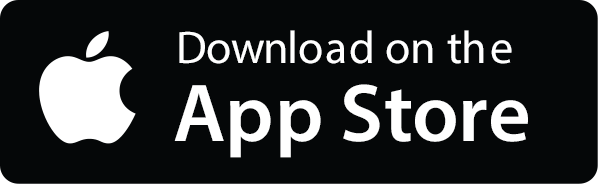
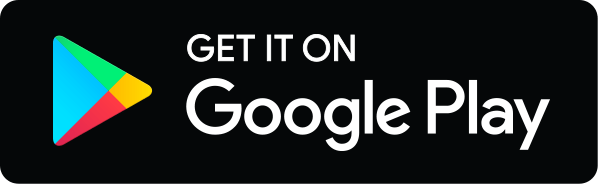
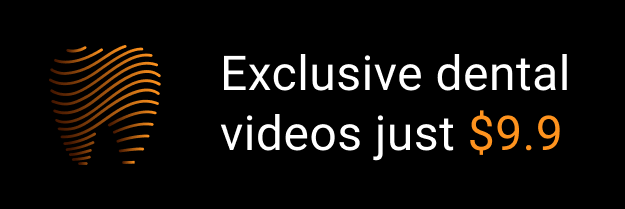