Abstract
The aim of this study was to compare the use of beta-tricalcium phosphate (β-TCP) (chronOS) with autogenous bone grafts alone in maxillary sinus elevation surgery. The test samples were β-TCP alone, β-TCP mixed with autogenous bone grafts (1:1), and autogenous bone grafts alone. Twelve maxillary sinuses were grafted with β-TCP (group 1), nine with β-TCP + autogenous bone graft (group 2), and 12 with autogenous bone graft (group 3). After 6 months, biopsies were obtained concurrent to the placement of dental implants; these were subjected to histomorphometric analysis and immunohistochemical analysis for runt-related transcription factor 2 (RUNX2) and vascular endothelial growth factor (VEGF). The average bone formation in group 1 was 46.3 ± 11.6% in the pristine bone region, 47.6 ± 9.9% in the intermediate region, and 44.8 ± 22.1% in the apical region; in group 2, values were 35.0 ± 15.8%, 32.5 ± 13.7%, and 32.8 ± 16.0%, respectively; in group 3, values were 43.1 ± 16.0%, 31.0 ± 13.0%, and 46.1 ± 16.3%, respectively. Immunostaining of samples in group 2 showed high cellular activity and immature bone; this differed from groups 1 and 3, in which mature bone was demonstrated. Thus, this study showed that β-TCP presents the same behaviour as autogenous bone graft, which makes it a good bone substitute.
The use of dental implants to restore the occlusion is one of the most frequently performed treatments in oral surgery today. However, in some cases, bone resorption prevents implant placement owing to the lack of bone height and thickness. In the posterior maxillary bone, sinus pneumatization can also restrict dental implant placement. The surgical procedure involving elevation of the Schneiderian membrane and the placement of bone substitutes, which have been researched extensively, enables the bone height to be re-established.
The ideal bone graft should provide a three-dimensional (3D) matrix to support the necessary cells that enable bone healing and resorption. Among the existing biomaterials (allogeneic bone graft, xenograft, and alloplastic materials), the autogenous bone graft is the only one that is able to initiate biological events culminating in osteogenesis, serving as an osteoinductor and osteoconductor. Furthermore, the autogenous bone graft contains osteoblastic cells, undifferentiated mesenchymal cells, osteoclasts, and growth factors.
For intraoral procedures, the best location for autogenous bone graft harvesting is the oral cavity; however, the amount of bone graft harvested may be insufficient for requirements. Biomaterials thus represent promising substitutes for the autogenous bone graft in maxillary bone augmentation. However, such biomaterials have to be biocompatible, non-antigenic, degradable, promote vascular growth, and stimulate osteogenesis.
One of the bone substitutes most used in dental surgical procedures and maxillary bone augmentation is beta-tricalcium phosphate (β-TCP), which has shown good reported outcomes. It is an osteoconductive material with interconnected pores that create a capillary force to draw cells and nutrients into the material; moreover, β-TCP is resorbed quickly, followed by new bone formation. Some authors believe that the use of biomaterials alone cannot provide the ideal structural resistance in the bone, thus compromising osseointegration in the long term. For this reason, some have proposed mixing the autogenous bone graft with osteoinductive factors to achieve better bone repair in addition to more volume.
The volumetric changes achieved with β-TCP alone, β-TCP mixed with autogenous bone graft in a 1:1 ratio, and autogenous bone graft alone after 6 months of bone healing have been demonstrated previously. In that study, a −38.3% bone volume change was reported for β-TCP, −43.8% for β-TCP + autogenous bone graft, and −45.7% for autogenous bone graft alone. The similarity of β-TCP and autogenous bone in relation to the maintenance of bone graft volume, suggested that β-TCP could be used safely for maxillary sinus augmentation. However, data for bone healing from histomorphometric and immunohistochemical assessments are still required.
Bone healing requires a suitable environment to promote vascular formation in order to supply nutrients and oxygen to the graft. Vascular endothelial growth factor (VEGF) is one of the most important vascular growth factors, because it promotes the migration and proliferation of endothelial cells. Initially, the regulation of bone healing occurs by osteoblastic differentiation, which is mediated by runt-related transcription factor 2 (RUNX2). RUNX2 transcription is regulated by bone morphogenetic proteins and fibroblastic growth factors in the early stages of osteoblast differentiation. The action of RUNX2 is mediated by pluripotent mesenchymal cells until the final stage of osteoblast differentiation, at which time the process is inhibited.
The aim of this study was to perform a histological evaluation of the healing process following the use of β-TCP (chronOS; DePuy Synthes, Paoli, CA, USA). The effects of β-TCP in combination or not with autogenous bone graft (1:1) were compared with the effects of autogenous bone graft alone at 6 months after maxillary sinus augmentation by quantifying the amounts of RUNX2 and VEGF.
The hypotheses of this study were (1) null hypothesis (H0): β-TCP does not present more new bone formation than autogenous bone graft alone; (2) alternate hypothesis (H1): β-TCP added to autogenous bone graft 1:1 presents more new bone formation than β-TCP alone.
Materials and methods
This prospective clinical study was performed at the Araçatuba Dental School – UNESP in São Paulo, Brazil, from March 2012 to November 2013, and was approved by the necessary ethics committee. Patients were included in the study if they presented edentulous posterior maxillary bone regions and a bone height of less than 5 mm, which necessitated bone augmentation for dental implant placement. Patients were excluded if they presented uncontrolled systemic problems or local problems, such as uncontrolled periodontitis or a sinus pathology. Smokers and patients who had received radiation treatment in the head and neck region were also excluded. Maxillary and mandibular cone beam computed tomography (CBCT) was performed prior to recruitment in order to evaluate the maxillary sinus and the bone height remaining in the maxillary floor, as well as the retromandibular and mandibular symphysis regions, to determine the volume of the mandible from where the bone grafts would be harvested. Based on these parameters, 22 patients requiring the augmentation of 36 maxillary sinuses were recruited.
Three groups were created for this study: group 1 comprised 12 maxillary sinuses augmented with β-TCP; group 2 comprised 12 maxillary sinuses augmented with β-TCP and autogenous bone graft (1:1); group 3 comprised 12 maxillary sinuses augmented with autogenous bone graft alone. There was no association between the side of the maxillary sinus and the graft material used. Randomization was performed by drawing lots to decide which sites would be grafted with each material. This was done by a clinical assistant, who was not involved in the surgeries or in the data evaluation. Two patients did not return for follow-up; thus the final analysis included only nine sinuses for group 2 and 11 for group 1.
Surgical procedures
All surgical procedures were performed under local anaesthesia using 2% lidocaine with 1:100,000 epinephrine (Nova DFL, Taquara, RJ, Brazil).
Harvesting bone grafts from mandibular bone
The autogenous bone graft was removed from the mandibular symphysis as follows: An incision was made in the inferior labial mucosa extending from the canine tooth to the mental muscle. The blade was then tilted towards the bone to incise the muscle and periosteum. The flap was elevated with a Molt No. 9 elevator (Golgran, São Caetano do Sul, SP, Brazil), and the mandibular symphysis bone was exposed and osteotomized with a 701 drill (KG Sorensen, Cotia, SP, Brazil) under constant irrigation with 0.9% saline solution (Darrow, Rio de Janeiro, RJ, Brazil). The retromolar bone graft site was approached through an intraoral incision, similar to a mandibular sagittal osteotomy, and osteotomized with a 701 drill; the graft was harvested with a chisel. The excised bone block was pulverized with a bone crusher (Neodent, Curitiba, PR, Brazil).
Maxillary sinus augmentation
The maxillary sinus was augmented according to the procedure of Boyne and James. During the first week, all patients were administered 500 mg paracetamol (EMS, São Paulo, SP, Brazil) four times per day to reduce pain and 500 mg amoxicillin (EMS, São Paulo, SP, Brazil) three times per day as antimicrobial prophylaxis to reduce the chance of infection.
Histomorphometric analysis
Biopsy samples were collected at the time of dental implant placement with a 3.0 × 15-mm trephine bur (MK Life, Porto Alegre, RS, Brazil) and stored in 10% formalin solution (pH 7) for 48 h. The samples were stored and maintained in the same apical orientation. They were then washed in running water for 24 h and decalcified in ethylenediaminetetraacetic acid (EDTA) solution for 4 weeks. The solution was changed weekly. Next, samples were embedded in paraffin maintaining the apical orientation, sliced to a thickness of 5 μm, placed on slides, and stained with haematoxylin and eosin. The biopsies were evaluated by light microscopy and the images were captured using an attached digital camera (JVC TK1270 colour video camera) at ×12.5 magnification. Three regions were identified for each biopsy specimen: pristine bone (2 mm above the upper side of the maxillary sinus floor), intermediate region, and apical region (2 mm below the Schneiderian membrane). The maxillary sinus floor was considered the most cortical bone in the lower part of the sample following the bone height determination by computed tomography ( Fig. 1 ). New bone and connective tissue formation was analyzed by histomorphometry using a Merz grid added to the images in PowerPoint for Mac (Microsoft, Redmond, WA, USA). Masson’s trichrome staining was used to show the initial stage of bone formation.

Immunohistochemical analysis
Primary polyclonal goat antibodies raised against human VEGF or RUNX2 were used in the immunohistochemical assays (Santa Cruz Biotechnology, Santa Cruz, CA, USA; catalogue numbers SC1881 and SC8566, respectively). A biotinylated donkey anti-goat secondary antibody (Jackson ImmunoResearch Laboratories, West Grove, PA, USA) coupled to avidin (Vector Laboratories, Burlingame, CA, USA) was used for signal amplification. The binding reaction was detected with diaminobenzidine (Sigma-Aldrich, St Louis, MO, USA), a chromogenic substrate for avidin. The biopsies were divided into the same three regions as used in the histomorphometric evaluation. The data analyses were performed using a single-evaluator semi-quantitative approach, with a score of ‘0’ indicating the absence of staining and scores of ‘1’, ‘2’, and ‘3’ indicating low, moderate, and intense staining, respectively.
Statistical analysis
The Shapiro–Wilk test was performed to determine whether the samples had a normal distribution. Comparisons between groups were performed using analysis of variance (ANOVA) followed by Tukey’s multiple comparison test (SigmaPlot 12.3; Systat Software, San José, CA, USA). An a priori P -value of <0.05 was used for all tests.
Results
Histomorphometry
The average bone height in the maxillary sinus floor was 3.2 ± 1.5 mm in group 1, 2.6 ± 1.6 mm in group 2, and 2.6 ± 1.3 mm in group 3.
The average new bone formation for group 1 was 46.3 ± 11.6% in the pristine bone region, 47.6 ± 9.9% in the intermediate region, and 44.8 ± 22.1% in the apical region ( Table 1 ). The newly formed bone presented a few woven bone areas and well cellularized connective tissue in all regions studied ( Fig. 2 ). The biopsies of this group showed mature bone and connective tissue that were cellularized and vascularized ( Fig. 3 ).
Region | Group 1 (%) A | Group 2 (%) B | Group 3 (%) AB |
---|---|---|---|
Pristine bone | 46.3 ± 11.6 a | 35.0 ± 15.8 b | 43.1 ± 16.0 c |
Intermediate | 47.6 ± 9.9 a | 32.5 ± 13.7 b | 31.0 ± 13.0 c |
Apical | 44.8 ± 22.1 a | 32.8 ± 16.0 b | 46.1 ± 16.3 c |
For group 2, the rate of new bone formation was 35.0 ± 15.8% in the pristine bone region, 32.5 ± 13.7% in the intermediate region, and 32.8 ± 16.0% in the apical region ( Table 1 ). With regard to the histological features, this group presented immature bone formation with woven bone areas and typical trabecular bone of type IV ( Figs. 4 and 5 ).
In group 3, the rate of new bone formation was 43.1 ± 16.0%, 31.0 ± 13.0%, and 46.1 ± 16.3% in the pristine bone region, intermediate region, and apical region, respectively. The bone formed in this group was mature, with osteoblastic cells on the periphery and well cellularized connective tissue ( Fig. 6 ).
No statistically significant difference in bone formation was observed among the three regions within each group ( P > 0.05). However, there was a statistically significant difference in bone formation between groups 1 and 2 ( P = 0.03) ( Table 1 ; Fig. 7 ).
For group 1, the amount of connective tissue was 48.8 ± 5.4% in the pristine bone region, 52.3 ± 9.9% in the intermediate region, and 55.1 ± 22.1% in the apical region ( P > 0.05). For group 2, the amounts were 56.8 ± 9.9% in the pristine bone region, 60.1 ± 13.9% in the intermediate region, and 55.5 ± 9.2% in the apical region ( P > 0.05). The amount of connective tissue found in group 3 was 55.1 ± 13.6% in the pristine bone region, 65.0 ± 10.2% in the intermediate region, and 50.2 ± 14.5% in the apical region ( P > 0.05) ( Table 2 ).
Region | Group 1 (%) A | Group 2 (%) A | Group 3 (%) A |
---|---|---|---|
Pristine bone | 48.8 ± 5.4 a | 56.8 ± 9.9 b | 55.1 ± 13.6 c |
Intermediate | 52.3 ± 9.9 a | 60.1 ± 13.9 b | 65.0 ± 10.2 c |
Apical | 55.1 ± 22.1 a | 55.5 ± 9.2 b | 50.2 ± 14.5 c |
Immunochemistry
A single evaluator assigned the scores for the two proteins probed in the three groups. In group 1, the intensity was low (‘1’) for preosteoblastic cells immunostained for RUNX2 in connective tissue in the pristine bone, intermediate, and apical regions ( Fig. 8 ). For group 2, immunostaining for RUNX2 was moderate (‘2’) in all regions, with cells presenting an osteoblastic phenotype next to the periphery bone and in the connective tissue ( Fig. 9 ). In group 3, a low (‘1’) level of RUNX2-positive staining was observed in all regions ( Fig. 10 ) ( Table 3 ).
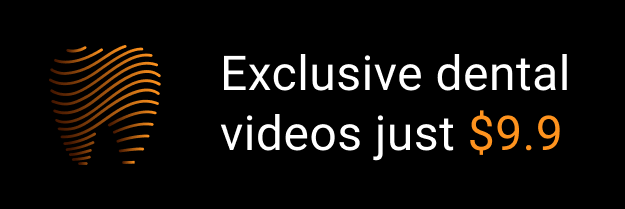