11
Unveiling and Managing Upper Airway Problems in the Orthodontic Patient
Mimi Yow1,2,3,4, Huiting Lynn Koh2,3,5, and Shaun Loh2,3,6
1 Department of Orthodontics, National Dental Centre Singapore
2 SingHealth Duke‐NUS Sleep Centre
3 Duke‐NUS Graduate Medical School
4 Faculty of Dentistry, National University of Singapore
5 Department of Otolaryngology, KK Women’s, and Children’s Hospital
6 Department of Otorhinolaryngology, Head and Neck Surgery, Singapore General Hospital
Obstructive sleep apnea (OSA) occurs when there is limitation of upper respiratory airflow due to partial or complete upper airway obstructions despite persistent respiratory effort. With reduced airflow, gaseous exchange is impaired and sleep is fragmented from recurrent arousals (Carberry et al., 2016). The afflicted individual is usually unaware of the problem. Snoring and sleep apnea are often reported by bed partners who witness the patient’s sleep‐breathing problems, or there may be suspicion of OSA from associated medical comorbidities of long‐term OSA; that is, metabolic, cardiovascular, neurological, ophthalmological, and urological disorders (Javaheri et al., 2017; Marrone and Bonsignore, 2020).
The spectrum of sleep‐disordered breathing
The signs and symptoms of what we now know as the sleep‐disordered breathing (SDB) spectrum were recognized centuries ago. They were described in literary works by William Shakespeare and Charles Dickens in the seventeenth and nineteenth centuries, respectively. In Shakespeare’s last play, The Tempest, Sebastian says to Antonio as they circle the sleeping bodies, “Thou dost snore distinctly; there is meaning in thy snores” (Shakespeare, 1987: Act II Scene 1). In his first novel, the Posthumous Papers of the Pickwick Club, Dickens (1837) described one of his characters, Joe, as “a wonderfully fat boy – habited as a serving lad, standing upright on a mat, with his eyes closed as if in sleep.” Obesity‐hypoventilation or Pickwickian syndrome was named with reference to Joe in the Pickwick Papers. Patients with this syndrome present with morbid obesity, hypersomnolence, periodic respiration, intermittent cyanosis, polycythemia, and heart failure (Burwell et al., 1956).
Snoring and OSA are events in a wide‐ranging SDB spectrum with variable severity and overlapping signs and symptoms that can occur in both adults and children (Schwab, 2003). There are different forms of SDB: primary or simple snoring without arousals or other symptoms is at the mildest end of the spectrum, while upper airway resistance syndrome (UARS) is more severe and associated with multiple respiratory effort‐related arousals (RERAs). Sleep hypopnea syndrome is characterized by snoring with symptoms increasing in severity, but with the absence of apnea, and this is followed by mild, moderate, and severe OSA, which progressively increases in severity along the continuum (Figure 11.1).

Figure 11.1 The spectrum of sleep‐disordered breathing: snoring; upper airway resistance syndrome (UARS); sleep hypopnea syndrome (SHS); obstructive sleep apnea (OSA); obesity hypoventilation syndrome (Pickwickian syndrome).
Decoding obstructive sleep apnea
A thorough examination of the patient with snoring and OSA is necessary in diagnosis. Coexistence of a wide range of other medical conditions such as cranio‐dentofacial anomalies, obesity, hypertension, cardiac dysfunction, chronic obstructive pulmonary disease, restrictive lung diseases, metabolic disease, muscular dystrophy, kyphoscoliosis, hypothyroidism, and pituitary tumors highlights the importance of interactive collaboration in overall management (Davila, 1995; Mathur and Douglas, 1995).
The gold standard test to confirm SDB is an attended overnight polysomnography (PSG). This is a hospital‐based test that records specific physiological parameters during sleep, which enables the diagnosis of OSA and other types of sleep disorders. The total number of respiratory events in an hour is expressed as the apnea–hypopnea index (AHI). AHI is derived from the sum of apneas and hypopneas in an hour of sleep.
Adult
An adult apneic event is defined as complete cessation of airflow, for 10 seconds or more, despite persistent respiratory effort. Hypopnea occurs when airflow reduces, by 30% or more, in a partially obstructed airway. Each episode of reduced airflow is significant if it lasts for at least 10 seconds with an arterial oxygen desaturation of 4% or more; or if there is a 50% or greater reduction in airflow for at least 10 seconds associated with an arousal due to hypercapnia. The criteria of apneas and hypopneas of 10 seconds’ duration are based on two lost breaths of adult normal respiration rate. Classification of mild OSA is 5–15 apnea and/or hypopnea events per hour; more than 15 and up to 30 events per hour is moderately severe OSA; and more than 30 events per hour is classified as severe OSA (Guilleminault et al., 1978; Berry et al., 2020).
Child
The polysomnographic criteria for defining pediatric OSA differ from those of adult OSA (Table 11.1). In children, the International Classification of Sleep Disorders, third edition (ICSD‐3) polysomnographic criteria for diagnosis of OSA are either (i) one or more obstructive events (obstructive or mixed apnea or obstructive hypopnea) per hour of sleep; or (ii) obstructive hypoventilation, as manifest by PaCO2 >50 mmHg for >25% of sleep time, together with snoring, paradoxical thoracoabdominal movement, or flattening of the nasal airway pressure waveform implying flow limitation. OSA consists of shorter apnea and hypopnea duration associated with pediatric respiration rates. An apneic event lasts for the duration of two lost breaths in children, about 6 seconds. Hypopnea in children refers to a drop in airflow by at least 30% for the duration of two breaths and associated with an arousal or 3% desaturation of arterial oxygen. An AHI of 1–4 events per hour indicates mild OSA, 5–9 events per hour is moderately severe OSA, and 10 or more events per hour is severe OSA in children (Iber et al., 2007).
Polysomnographic values of note are the AHI and the apnea index (AI) in grading the severity of OSA. Based on positive clinical findings, even if the AHI value is low, a diagnosis of OSA with an AHI of 1 is significant in children. Clinical information is as important as AHI and AI values in determining the clinical severity and morbidity of pediatric sleep‐disordered breathing (Marcus et al., 1992; Chan et al., 2004). Affected children demonstrate behavioral problems and learning difficulties, hyperactivity, inattention, aggression, and neurocognitive deficits with impact on memory, learning, and executive functions. A child who snores at least two nights per week or more is significantly at risk of negative behavioral outcomes and decline in cognitive functions. The frequency of snoring regardless of AHI scores was predictive of adverse outcomes and cognitive functions (Smith et al., 2017).
Table 11.1 Characteristics of children and adults with obstructive sleep apnea.
Parameters | Child | Adult |
---|---|---|
Peak age | 2–6 years | 30–60 years |
Sex ratio (female: male) | 1 : 1 | 1 : 2 |
Apnea–hypopnea index | ≥1 | ≥5 |
Estimated prevalence | ||
–General population | 1–3% | 2–4% |
–Habitual snorers | 9% | 10–40% |
–Morbidly obese | 37–55% | >50% |
Body mass index (BMI) | Low: failure to thrive <3rd percentile High: >95th percentile |
≥28 Asian; ≥30 Caucasian |
Common causes | Adenotonsillar hypertrophy Craniofacial anomalies |
Obesity Dentofacial anomalies |
Research findings suggest that neurocognitive deficits are partially reversible, but there may be a residual learning deficit representing a “learning debt” (Gozal and Pope, 2001; Larkin et al., 2005). Children with AHI ≥5 have raised levels of C‐reactive protein (CRP), which is known to increase the risk of cardiovascular disease. Children with OSA may also have autonomic dysfunction with increased sympathetic activity (Gozal and O’Brien, 2004). In addition, there is evidence of systemic inflammation in OSA patients (Li et al., 2008). The inflammatory mediator cysteinyl leukotriene (cys‐LT) has been found in exhaled breath condensates of children and is related to the severity of SDB (Goldhart et al., 2006).
In children, OSA is complex and cannot be excluded on the basis of history and physical examination (Gottlieb and Young, 2009). A diagnosis of OSA based on history and physical examination is inadequate (sensitivity 63% and specificity 60%). Home‐based three‐night pulse oximetry is a better screening tool. Test results with an oxygen desaturation index (ODI) >10 demonstrated a sensitivity of 93% and specificity of 75% for moderately severe to severe OSA (Chung et al., 2016; Galway et al., 2021). If there is high clinical suspicion, the patient should be referred to a sleep‐trained pediatrician or a multidisciplinary sleep team for possible causes and confirmation of the diagnosis. Studies conducted on the predictive value of screening tools using history, clinical signs and symptoms, and overnight pulse oximetry indicated there was no foolproof method for confirming OSA (Hoffstein and Szalai, 1993). Full‐night (type 1) polysomnography is still the gold standard test and is recommended for the diagnosis of OSA in both adults and children (Kushida et al., 2005).
Epidemiology
Prevalence
Snoring and OSA are common. Reported prevalence of snoring in adults ranges from 32% to 52.8%, and 16.7% to 30.6% in children from different countries (Ferreira et al., 2000; Teculescu et al., 2001). In another study, the global prevalence for adults with OSA based on data from 16 countries exceeded 50%, affecting 936 million people aged between 30 and 69 years of age. Global prevalence for those with moderate to severe OSA was 45% (Benjafield et al., 2019). In most children, primary snoring does not progress to OSA and resolves spontaneously except in about 11%. In a Caucasian group of 3–6‐year‐olds who were habitual snorers, 13% had OSA, whereas in an Asian population of 7‐year‐olds who were habitual snorers, 9% developed OSA after three years (Castronovo et al., 2003; Anuntaseree et al., 2005). Among morbidly obese children between the ages of 6 and 19 years, OSA prevalence increases to 55% (Chay et al., 2000). There is evidence linking OSA with cardiovascular and metabolic diseases, affecting not only children with OSA, but also children with primary or habitual snoring (Ali et al., 1993; Brunetti et al., 2001; Ferreira et al., 2000; Gozal and Pope, 2001; Chervin et al., 2002; Chng et al., 2004; Gottlieb et al., 2004).
Risk factors
Body mass index (BMI) is an independent factor that correlates with severe OSA. In the morbidly obese, the incidence of OSA increases by at least 12–30 times (Young et al., 1993; Kyzer and Charuzi, 1998; Resta et al., 2001). Weight gain and obesity are important factors in the development and progression of OSA in middle‐aged patients. The World Health Organization (WHO) recommends cutoff points to indicate obesity at BMI ≥30 kg/m2 for Caucasian adults and ≥28 kg/m2 for Asian adults; Asians have a higher body fat percentage at a lower BMI (WHO Expert Consultation, 2004). Other than age, sex, and obesity, general characteristics of OSA patients are low waist–hip ratio and high serum cholesterol concentration (Tishler et al., 2003). Different physical features characterize patients with OSA (Table 11.1).
Respiration: Effect of anatomy and sleep
Effect of anatomy on respiration
The upper airway tract comprises three components: the nose, the pharynx, and the larynx. The nose, portal to the upper airway, is divided into two openings by the nasal septum; there is an alternative route should one nostril be blocked. The nasal airway is the preferred route for resting ventilation and infants are obligatory nasal breathers (Hall et al., 2000). The structures maintaining the patency of the anterior nares are the alar, procerus, compressor, and dilator naris muscles. The anterior nasal valves are responsible for much of the nasal resistance in patients with normal nasal apertures. The nose contributes up to half of the total airway resistance in the normal awake patient (Ferris et al., 1964). The lateral nasal walls contain several protuberances, the nasal turbinates, which serve two functions: creation of air turbulence to slow down airflow to prolong air contact with the highly vascularized nasal mucosa for temperature and humidity control; and production of nasal secretions with antimicrobial actions on inhaled air before it is channeled into the lungs. With increased ventilatory drives to meet physiological demands, the nasal apertures widen, and nasal mucosa constricts to reduce airflow resistance. At an individual physiological threshold of ventilatory demand, a secondary route of oral breathing is established to bypass nasal resistance, thereby increasing the volume per breath.
Inspired air passes through the nasal airway to the pharynx via the bilateral choanae. The pharynx traverses three regions: the nasopharynx, which extends from the posterior nasal choanae to the hard palate; the oropharynx, which comprises the retropalatal and retroglossal segments – the former extends from the level of the hard palate to the caudal margin of the soft palate and tip of the uvula, and the latter from the soft palate margins to the base of the epiglottis; and the laryngopharynx, which is the segment from the base of the tongue to the larynx. Narrowing of the posterior nasal choanae occurs in patients with craniofacial abnormalities. Pharyngeal airway narrowing or collapse is common in the retropalatal and retroglossal aspects of the pharynx, with a majority of patients having more than one site of obstruction (Hudgel, 1988; Horner et al., 1989; Launois et al., 1993; Morrison et al., 1993; Suto et al., 1993; Trudo et al., 1998; Ciscar et al., 2001). Nasendoscopic studies describe the mechanics of pharyngeal airway narrowing as a function of sphincteric movement of the lateral pharyngeal walls together with backward movement of the anterior structures. Posterior pharyngeal wall movement is not implicated in airway narrowing (Weitzman et al., 1978) and the narrow parts of the pharyngeal lumen are potential choke points (Wellman et al., 2014).
The larynx connects the pharynx with the lower airway, the trachea. It is responsible for phonation as well as protection of the trachea. It is bounded superiorly by the epiglottis, inferiorly by the vocal cords, and laterally by the aryepiglottic folds. These structures work in synchrony to channel air into the anterior tract, the trachea, whereas food and liquids are directed into the posterior tract, the esophagus. The epiglottis acts as a valve to separate the trachea from the esophagus during deglutition and to maintain a patent airway during respiration. The posterior cricoarytenoid is the only abductor muscle that widens the glottic aperture during inspiration and expiration. The tongue and lingual tonsils, which stud the dorsum of the posterior third of the tongue, may hinder the function of the epiglottis if the lingual tissues are enlarged and encroach into the posterior airway space (Suzuki and Kirchner, 1969; Wyke, 1974).
Redundant mucosa in the arytenoid–aryepiglottic region has been associated with OSA. Vocal cord abnormalities are rarely implicated in symptomatic OSA; however, bilateral vocal cord paralysis has been reported to be associated with snoring. Rarely, congenital vallecula cysts occur between the base of the tongue and the epiglottis. When cysts are present in this region or in the larynx, they are sometimes associated with airway obstruction, stridor, pyoceles, or vocal cord paralysis (Griffith et al., 1993).
Many cephalometric studies show an association between craniofacial characteristics and OSA. These include hard and soft tissue features such as reduced mandibular body length (Miles et al., 1996), posteriorly positioned maxilla, and inferiorly positioned hyoid bone (deBerry‐Borowiecki et al., 1988; Bacon et al., 1990; Pracharktam et al., 1994; Lowe et al., 1995). Other distinguishing craniofacial features of OSA patients are reduced cranial base length, mandibular or maxillomandibular retrusion, increased lower face height, an elongated soft palate, a large tongue, and a smaller upper airway compared with normal individuals (Horner et al., 1989; Ciscar et al., 2001).
The oropharyngeal airway is usually narrow in individuals with OSA (Shepard and Thawley, 1989; Schwab et al., 1993, 2003). Reduced size of the posterior airway space and increased distance of the hyoid bone to the mandible are also associated with those with OSA. The two cephalometric parameters that are used as indicators of OSA are posterior airway space of <11 mm and a hyoid to mandible distance of >15.4 mm (Riley et al., 1983). Severe respiratory disturbance indices are associated with a posterior airway space of <5 mm at the base of the tongue and a distance of the hyoid bone to the lower border of the mandible of ≥24 mm (Partinen et al., 1988). There is a low probability of OSA if the airway cross‐sectional area is >110 mm2, but a high probability of severe OSA if the area measures <52 mm2. Most constrictions are located in the oropharynx (Lowe et al., 1986; Avrahami and Englender, 1995; Ogawa et al., 2007).
There are also differences in the soft tissue characteristics of OSA patients compared with those without OSA; besides a larger tongue, OSA patients have thicker and longer soft palates and parapharyngeal fat pads in the lateral pharyngeal walls (Schwab, 2003; Schwab et al., 2003).
Effects of sleep on respiration
The diaphragm is the main driver of ventilation during sleep. Pharyngeal airway patency is well maintained during wakefulness, but during sleep pharyngeal and genioglossal muscle activity is reduced, which predisposes to sleep‐dependent pharyngeal airway collapse (McNicholas, 2002; Jordan and White, 2008). Sleep adversely affects tonic and phasic skeletal muscle functions. There is evidence suggesting that in OSA, the intrinsic upper airway muscle function and central neural regulation of the upper airway dilator muscles and genioglossus may be impaired. Recurrent apnea during sleep induces intermittent hypoxia that alters respiratory muscle contraction, reducing muscle endurance with diminished pharyngeal electromyographic (EMG) responses to physiological changes (Bradford et al., 2005).
Sleep reduces the strength of muscular tone and, consequent to that, the negative pressures of inspiration may suck the tongue into the pharyngeal airway (Remmers et al., 1978). The mechanical properties of the upper airway that maintain tissue tone and stiffness to preserve airway patency are reliant on intrinsic physiological properties. In patients with OSA, there is reduced effectiveness of upper airway dilator muscle contractions and genioglossal reflex. The genioglossal negative pressure reflex declines with age, which may explain age‐related onset of OSA. Current evidence suggests a biomechanical basis for OSA in patient with anatomical and sleep‐positional predisposition to pharyngeal collapse (White et al., 1985; Sériès, 2002; Malhotra et al., 2004; Dempsey et al., 2010).
Mechanical influences on respiration
There are several mechanical influences on the airway diameter, causing it to be open, narrowed, or closed. These factors can be either static or dynamic. Static factors are surface adhesive forces, neck and jaw posture, tracheal tug, gravity, and sleep position. Dynamic factors comprise upstream resistance within the nasal airway and pharynx: the Bernoulli effect and dynamic compliance (Schwab et al., 2005).
Several studies have demonstrated a smaller upper airway width in patients with OSA compared with patients without OSA. The reduction in size of the upper airway has been attributed to the enlargement of the surrounding soft tissues: fat, muscles, and lymphoid tissues in the tongue, soft palate, and lateral and parapharyngeal walls. Reduced airway diameter affects airway resistance the most, as described by Poiseuille’s law: R = 8nl/pr 4; the resistance (R) and the length of the tube (l) are inversely related to its radius (r) and pressure (p). By halving the size of the tube, intraluminal resistance increases by 16 times (Shepard and Thawley, 1989; Schwab et al., 1993, 2003; Schwab, 2003).
Nasal obstruction has been implicated as a cause of OSA due to nasal resistance and mouth breathing. Nasal resistance, measured by posterior rhinomanometry, is reported to be higher in individuals with OSA. Nasal resistance during inspiration places the upper airway at a greater risk of collapse (Isono et al., 2004). During sleep, the genioglossal activity increases when an infant switches from oral to nasal breathing. There is evidence correlating increased genioglossal EMG activity with changes in airway resistance and carbon dioxide levels (Roberts et al., 1986).
Mouth breathing plays a role in pharyngeal airway obstruction by changed dynamics of the airway that reduces airway caliber and patency. With mouth opening, the mandible and its soft tissue attachments rotate down and back, restricting the retroglossal and hypopharyngeal airway. When the tongue is lowered, it detaches from the soft palate and becomes free moving. With gravity and poor tongue muscle tone, it falls downward and backward into the pharyngeal airway, causing partial or complete obstruction. Reduced surface adhesion forces of mucosal surfaces are also contributory to airway obstruction (Issa and Sullivan, 1984; Roberts et al., 1985).
The child with sleep‐disordered breathing
The clinical morbidities associated with obstructed upper airways were first reported in medical publications more than a century ago. In 1889, Ambroise Guye described “the impairment of cerebral functions by disorders of the nose.” The obstructive effect of enlarged adenoid masses, particularly during sleep, was observed to produce “aprosexia” – the inability to fix the attention on any abstract subject. With removal of the adenoid mass from the nasopharynx, headaches ceased and learning difficulties were prevented (Guye, 1889).
This observation was confirmed soon after by William Hill (1889), who was “much struck by the fact that operations on children, undertaken for the relief of deafness associated with adenoid growths, enlarged tonsils, and hypertrophic catarrhal conditions of the nose, have frequently resulted in such an immediate improvement in the mental acuteness of the patients as was altogether incommensurate with the often, immediate improvement in the sense of hearing.” He advised that the “stupid looking lazy child who frequently suffers from headache at school, breathes through his mouth instead of his nose, snores and is restless at night, and wakes up with a dry mouth in the morning, is well worthy of the solicitous attention of the medical officer.”
Similar to Hill’s and Guye’s observations and advice, the current evidence‐based management calls for screening of children for snoring and recommendation of adenotonsillectomy in children diagnosed with OSA (Marcus et al., 2012; Venekamp et al., 2015).
History, physical examination, and diagnosis
All children should be screened for snoring as part of well‐child visits to identify at‐risk children who will benefit from a thorough evaluation and confirmation of OSA by sleep‐trained physicians (Marcus et al, 2012). A child with a mild form of SDB without diagnosis and treatment could progress to a more severe condition in over two years (Li et al., 2010). Habitual snoring of more than two nights per week warrants evaluation (Marcus et al., 2012; Smith et al., 2017). Early detection leads to prevention‐driven management of chronic comorbidities and poor quality of life associated with established SDB (Guilleminault et al., 2019; Tan et al., 2020).
Habitual snoring, if present, should prompt for a more detailed history of other signs and symptoms. Symptoms are commonly classified into night symptoms and daytime symptoms. Night symptoms include unusual sleeping postures, restless sleep, labored sleep breathing, and observed apneas. Daytime symptoms include unrefreshed sleep, morning headaches, hyperactivity, attention deficit, learning difficulties, and behavioral problems. Hyperactivity is more likely to be present in the child with OSA than is excessive daytime sleepiness; the latter occurs more often in adults with OSA (Leach et al., 1992; Carroll, 1996). Selective screening of high‐risk children with craniofacial syndromes and craniofacial synostosis is effective in picking up children with OSA, particularly in those with complex craniosynostosis and fusion of at least two cranial sutures. Snoring is common in 77% of children with craniofacial syndromes and there is a 40% chance of developing OSA (Bannink et al., 2010). In a study of children with Apert, Crouzon, Muenke, Pfeiffer, and Saethre–Chotzen craniosynostotic syndromes, the critical clinical question that identified children with OSA was “Has the child difficulty with breathing during sleep?” A negative response excluded clinically significant OSA (high negative predictive value of 91% and sensitivity of 64%). Many pediatric sleep questionnaires have been developed to screen children for the presence of SDB, though none of them is widely accepted. An approach to screening children for SDB is the use of a simple instrument, a five‐item BEARS questionnaire (Tan et al., 2020). The questionnaire increases the likelihood of identifying sleep problems among preschoolers, school‐aged children, and adolescents in a primary care setting.
A good physical examination and identifying sites of airway obstruction form the basis for diagnosis and effective treatment of pediatric SDB. It is important to start with a general examination of the child presenting with SDB symptoms. The risks of OSA are higher in children with a family history of SDB, cranio/dentofacial structural anomalies, prematurity, neuromuscular disorders, Down syndrome, cleft lip and palate after pharyngoplasty, morbid obesity, asthma, and atopic disease (Brouillette et al., 1982; Mallory et al., 1989; Marcus et al., 1991; Silvestri et al., 1993; Guilleminault et al., 1995; Chng et al., 2004; Savini et al., 2019). There is a positive correlation between OSA and children with obesity and without adenotonsillar hypertrophy (Verhulst et al., 2008). Obesity in a child is defined as having a BMI that is above the 95th percentile in their age and sex cohort. It is a primary independent factor in increased respiratory disturbance index (RDI) in children. Children with BMI ≥180% of ideal body weight are deemed to be morbidly obese, and between 37% and 55% of such children have OSA (Brooks et al., 1998; Chay et al., 2000; Mei et al., 2002). Besides the body habitus of the child, a note should be made about the presence of retrognathia or micrognathia and craniofacial abnormalities like midface hypoplasia, narrow nasal aperture, and open mouth posture.
Adenotonsillar hypertrophy reduces pharyngeal airway caliber and it is the most common finding in otherwise well children with SDB. The adenoid size may be examined via the use of flexible nasendoscopy or lateral cephalometry. The region of greatest pharyngeal airway narrowing is the area where the adenoid and tonsil masses overlap (Arens et al., 2003). The Brodsky grading of enlarged adenoids and tonsils (Brodsky, 1989) is reliable and easy, with good interrater agreement (ICC 0.721 and Cronbach alpha 0.911; Kumar et al., 2014). The Scammon growth curves demonstrate accelerated growth of the lymphoid tissue from birth, reaching its maximum at 11–13 years. Total body lymphoid tissue of a 13‐year‐old may attain a mass of almost 200% greater than that of an adult. Craniofacial skeletal growth follows a different timing and growth trajectory from lymphoid tissue growth; the latter tails off between 14 and 20 years of age, while the cranial vault and upper face follow the neural pattern of growth, which peaks between 8 and 12 years of age, with growth ceasing between 12 and 16 years of age. Growth of the lower face and mandible follows the general adolescent growth spurt that peak between 14 and 18 years and slows down or stops after 20 years of age (Scammon, 1930). As a result, the skeletal “container” may be too small to accommodate enlarged lymphoid tissues due to mismatched timing of skeletal and soft tissue growth.
Another common area of airway obstruction is the nose. Examination of the nose is important to identify structural abnormalities such as the presence of a deviated nasal septum and inferior turbinate hypertrophy, often resulting from a history of allergic rhinitis. When the nose is obstructed, the secondary respiratory route or mouth breathing takes over, with postural and functional changes of the lip, tongue, and bucco‐facial musculature. Some children with mouth breathing from an obstructed nasal and/or nasopharyngeal airway develop specific dentofacial characteristics: long narrow face or “adenoid facies,” increased anterior lower face height, downward and backward rotation of the mandible, high‐vaulted narrow palate, lowered tongue posture, incompetent lips, excessive upper gingival show, anterior open bite with retrognathic mandible and/or retrognathic maxilla, and forward head posture (Guilleminault et al., 2016; Brożek‐Mądry et al., 2021). Research findings attribute the bidirectional ramifications of dentofacial and oral features to altered breathing and its effects on craniofacial growth and development (Vargervik et al., 1984; Huang and Guilleminault, 2013; Grippaudo et al., 2016).
Using history taking, questionnaires to elicit sleep‐related symptoms, and clinical assessment will fail to detect OSA for one in five children (Chay et al., 2000). The American Academy of Pediatrics recommends an overnight polysomnogram (PSG) to confirm the diagnosis of SDB or OSA. However, a study showed that only 10% of pediatric otolaryngologists would obtain a diagnostic PSG prior to surgical treatment (Mitchell et al., 2006). Reasons cited for not obtaining PSG include concerns about a child’s emotional distress over the testing, high cost, and lack of access to a sleep laboratory. The American Academy of Otolaryngology published a guideline advocating preoperative PSG in the subgroup of high‐risk children who are syndromic, have craniofacial abnormalities, obesity, neuromuscular disorders, sickle cell disease, and mucopolysaccharidoses. The role of PSG in this high‐risk group is to identify nonobstructive (central) apneas that will not benefit from surgery, provide diagnostic certainty in patients who have increased risk of surgery, ascertain the need for preoperative anesthetic assessment and postoperative monitoring, and document the baseline severity of SDB for comparison after surgery if persistent SDB is anticipated (Marcus et al., 2012).
Cephalometrics
In children with adenotonsillar hypertrophy, narrowing of the airway is usually at the retropalatal region where the soft palate, adenoid, and tonsils overlap (Isono et al., 1998; Fregosi et al., 2003; Figure 11.2). There is high sensitivity in identifying adenoid hypertrophy by using lateral cephalograms (Duan et al., 2019). Orthodontic two‐ or three‐dimensional (3D) diagnostic imaging by lateral cephalometry or ultra low dose‐low dose cone beam computed tomography (van Bunningen et al., 2022) are precise and able to show potential obstructions in the upper airway space that require further investigations:
- Percentage of nasopharynx occupied by the adenoid mass compared to percentage airway norm for a 6‐year‐old is more than 51% (Handelman and Osborne, 1976; Schulof, 1978).
- Reduced distance from the posterior nasal spine (PNS) to the nearest adenoid mass measured along the PNS‐basion; normal distance in a 6‐year‐old is approximately 15–21 mm (Linder‐Aronson, 1970; Schulof, 1978).
Figure 11.2 Significant parameters in a lateral cephalogram of a child with obstructive sleep apnea: Large adenoids and tonsils causing narrowing of the nasopharyngeal and oropharyngeal airway.
- Reduced distance from the PNS to the nearest adenoid mass measured along a line through the PNS perpendicular to the sella‐basion; normal measurement in a 6‐year‐old is approximately 15–16 mm (Schulof, 1978; Linder‐Aronson and Leighton, 1983).
- Reduced distance to the nearest adenoid mass from a point on the pterygoid vertical 5 mm above the PNS; the normal value is approximately 7 mm (Ricketts, 1954; Schulof, 1978).
Assessment of the upper airway by drug‐induced sleep endoscopy (DISE) or cine‐magnetic resonance imaging is used for dynamic characterization of structures at the obstructed site(s) for surgical treatment planning in children with persistent OSA (Li et al., 2023).
Orthodontic management
Growth modification of the maxilla and mandible
Rapid maxillary expansion (RME) with maxillary protraction increases the transverse width of the maxilla and enlarges the palatal and retropalatal areas. This improves the ratio of airway space relative to adenotonsillar size. Enlargement of the dental arches, transversely and antero‐posteriorly, increases the intraoral space for accommodating lingual and sublingual tissues by removing the constraints of narrow maxillary and mandibular arches (Viva et al., 1992; Defabjanis, 2003). Systematic review of prepubertal RME treatment showed increased widths of the nasal and maxilla lateral walls by 3.3 mm and 3.4 mm, respectively (Seif‐Eldin et al., 2019). Villa et al. (2007) reported that the majority of children (78.5%) treated with RME had significantly reduced AHI and 85.7% of the children switched from oral to nasal breathing. A Cochrane systematic review showed that treatment with orthodontic appliances in children with mild OSA could reduce AHI, nasal congestion, and habitual snoring. Although the evidence is equivocal, dentofacial growth modification can be considered as adjunctive therapy for OSA management in children with dentofacial anomalies (Carvalho et al., 2016; Figure 11.3).
Correction of antero‐posterior or maxillo‐mandibular deficiencies in children have been shown to improve SDB. Forward repositioning of the mandible with functional appliances enlarges the upper airway and improves respiratory function after six months. The AHI improved in more than 60% of patients (Villa et al., 2002). Results of studies on the effects of maxillary protraction on airway dimensions in children with Class III malocclusion are nonconclusive. In one study, significant favorable skeletal changes of the maxilla and mandible did not result in short‐term or long‐term changes in sagittal airway dimensions of the nasopharynx and oropharynx (Baccetti et al., 2010). Another study reported a favorable increase in airway dimensions with maxillary protraction. Maxillary protraction increased the antero‐posterior width of the upper airway and nasopharyngeal airway area (Oktay and Ulukaya, 2008). The increased airway size remained significant after four years. The oropharyngeal airway area, however, did not increase immediately after active treatment, although it increased significantly during a four‐year follow‐up period (Kaygisiz et al., 2009). The study suggested that increasing oropharyngeal airway caliber post active treatment may be an effect of accelerated growth.
A randomized control study of nonobese 4–10‐year‐old children with OSA (AHI ≥1) reported normalization of AHI in 50% of patients who used customized oral appliances to advance the mandible for six months. The use of oral appliances was associated with reduced tonsillar hypertrophy in about 67% of patients compared with 14% of children in the control group. About 74% of the patients tolerated the appliance treatment well (Villa et al., 2002).
A review of dentofacial orthopedic appliances showed them to be effective as nonsurgical alternatives in the management of childhood OSA in the short term. Longitudinal data are needed to confirm if orthodontic and dentofacial orthopedic treatment for children with OSA can be curative (Carvalho et al., 2007).
Treatment of children with sleep‐disordered breathing and the role of surgery
Sleep‐disordered breathing in children is a heterogeneous condition with overlapping phenotypes. As such, polysomnographic diagnosis and clinical findings are equally important in determining the treatment plan. Phenotypic features can be divided into anatomical and nonanatomical factors. Anatomical hard and soft tissues comprise craniofacial anomalies, nasal anatomy, adenotonsillar enlargements, and body habitus. The nonanatomical factors are arousal threshold, loop gain, and muscle reactivity (Carberry et al., 2018).
Practice guidelines from the American Academy of Pediatrics and American Academy of Otolaryngology–Head and Neck Surgery (AAO‐HNS; Trosman, 2013) both recommend adenotonsillectomy as primary therapy for OSA in healthy children older than 2 years. These healthy children have the commonest anatomical phenotype in pediatric SDB: adenotonsillar hypertrophy, possibly associated with a long face, narrow palate, and minor malocclusions. Enlarged tonsils, of Brodsky grade 2+ or larger associated with oxygen desaturation, are good predictors for the success of adenotonsillectomy (Heath et al., 2021). Removal of the tonsils in the group has a cure rate of up to 72%, as defined by AHI <1 (Lee et al., 2016). For children with mild OSA and parents who decline surgical treatment, conservative treatment of allergic rhinitis may be effective in some patients. Meta‐analysis of treatment with intranasal steroids showed a reduction of AHI by 1.1 events per hour (Liu et al., 2015), while there was a reduction of AHI by 4.2 events per hour when intranasal steroids were used in combination with oral montelukast medication (Liming et al., 2018).

Figure 11.3 Dentofacial orthopedic appliances to increase maxillary/nasal width and protract the mandible. (a) Hyrax rapid maxillary expander (RME) with upper twin block appliance (TBA). (b) Lower TBA with upper RME and upper TBA.
Another anatomical phenotype comprises children with congenital anatomical features that predispose them to SDB, such as micrognathia and the Pierre Robin sequence, maxillary hypoplasia, mid‐nasal stenosis, and craniofacial synostosis. The cornerstone in planning optimal treatment for this group is the ability to determine the specific causes and locations of upper airway narrowing and collapse. Without precision profiling of the phenotypes, the gold standard treatment is continuous positive airway pressure (CPAP) therapy to pneumatically splint the airway open. It is 90% effective, but limited by low compliance of 50% or more in nonadherence to treatment (Sawunyavisuth et al., 2021). A detrimental side effect from long‐term CPAP therapy is midface hypoplasia from the CPAP mask that retards maxillary growth (Ghadiri and Grunstein, 2020).
Surgical procedures of the hard and soft tissues are alternative options to establish patency of the upper airway when conservative management with positive airway pressure therapy fails. Adenotonsillectomy is considered to be the first‐line approach in managing OSA of otherwise well children who are 2 years and older with significant adenotonsillar hypertrophy and OSA. RME in growing children improved much more in the short term (under three years) for those with previous adenotonsillectomy or who have small tonsils. The cure rate was 25.6% in AHI reduction to below 1 event per hour and ODI increased to above 90% (Camacho et al., 2017). Mandibular distraction osteogenesis and maxillary protraction are other options to relieve upper airway constriction from disproportion in craniofacial growth (Garg et al., 2017). Early tracheostomy may also be considered for syndromic infants with an obstructed or unstable upper airway (Katz et al., 2012).
A third separate phenotype of children with SDB is best described as an “adult” phenotype, where the child is older with obesity in addition to the facial features of chronic nasal obstruction such as midface hypoplasia and open mouth posture. These children may have adenotonsillar hypertrophy, or the lymphoid tissues may have started regressing in size. Treatment of such children will have a large emphasis on weight management. Adenotonsillectomy success rates as defined by AHI <1 have been reported to be much lower, at 12–34% (Lee et al., 2016). Patients who have “failure” of adenotonsillectomy to cure OSA may be offered positive airway pressure therapy or site‐specific airway procedures, which target the nose, oropharynx, and/or hypopharynx.
The adult with sleep‐disordered breathing
History
Clinical assessment with a thorough history and examination is an important tool for evaluating adults with suspected SDB to identify those who need further evaluation and polysomnography. Symptoms associated with SDB can be classified into nocturnal and daytime symptoms.
Common nocturnal symptoms include persistent snoring, witnessed apneas, choking, nocturia, frequent awakenings, and restless sleep. The most commonly reported nocturnal symptom is snoring. Loud heavy snoring interspersed with periods of silence, which are apneic episodes, is associated with OSA. The history of snoring is best obtained from the bed partner, as most patients are unaware of their own snoring. Apart from snoring, it is important to ask about witnessed apneas or choking episodes. Patients who wake up two or more times per night to pass urine may have nocturia, a common symptom in OSA, reported to occur in up to 84% of OSA patients (Miyauchi et al., 2015). The occurrence of nocturia associated with OSA is postulated to be due to increased secretion of atrial natriuretic peptide, which increases urine production, coupled with increased abdominal pressure transmitted to the bladder due to ongoing inspiratory effort against an obstructed upper airway. Patients and/or bed partners sometimes report loud snorts, vocalization, or body movements associated with brief awakenings, which usually occur during the termination of apneic episodes.
Common daytime symptoms include excessive daytime sleepiness (EDS), dry mouth in the morning, morning headaches, impaired neurocognitive function, and mood disorders. EDS in individuals with OSA results from respiratory arousal‐related sleep fragmentation and poor‐quality sleep. The perception of EDS is subjective and can be hard to quantify. Screening for EDS in adults is easily accomplished with the Epworth Sleepiness Scale (ESS), an eight‐item questionnaire where patients score their likelihood of falling asleep in various scenarios, such as monotonous soporific situations or during driving. A summative ESS score of >10 predicts a possibility of hypersomnolence (Johns, 1991, 1992). However, EDS can also be a result of insufficient sleep and it is therefore important to enquire about the patient’s sleep and wake times.
Approximately 12–18% of patients complain of morning headaches that are usually described as dull and generalized (Russell et al., 2014). The exact mechanism is unclear, but is thought to be due to nocturnal oxygen desaturation and hypercapnia that results in cerebral vessel dilation and increased intracranial pressure. Neurocognitive impairments such as reduced vigilance, impairment in short‐ and long‐term memory, as well as reduced executive functioning are commonly associated with OSA. EDS and its consequent impact on quality of life and daytime functioning result in mood disturbances in some patients. Depression is the most common mood symptom among other mood disorders like anxiety, irritability, and emotional lability. Individuals with OSA have a constellation of medical comorbidities (Bonsignore et al., 2019) and the comorbidity burden increases with OSA severity (Tveit et al., 2018).
Physical examination
A comprehensive physical examination of the OSA patient includes body habitus, craniofacial and dental characteristics, as well as upper airway soft tissue factors that influence the size and collapsibility of the upper airway
Measurement of height and weight and calculation of the BMI is an important part of examination, as obesity is frequently associated as an independent risk factor for OSA (Jehan et al., 2017). Increased circumference and the degree of fat deposition around the neck have been shown to be a reliable indicator for OSA. Prior studies have shown that neck circumference measurements of ≥43 cm for men and ≥37 cm for women are associated with increased risk of OSA, and further investigation of these patients should be performed (Katz et al., 1990; Kushida et al., 1997).
Mandibular deficiency is the most common craniofacial deformity associated with OSA. Retroposition of the mandible results in posterior displacement of the tongue base and a narrow retroglossal airway. These patients with mandibular deficiency generally fall into several growth patterns: primary mandibular deficiency, long face growth pattern, or maxillomandibular deficiency. Craniofacial assessment should be done with the patient’s head positioned in the natural head posture to determine the skeletal class. Examination of the mandible should encompass assessment of both antero‐posterior and transverse dimensions considering the transverse width, chin–throat length, and chin‐to‐neck angle. A small or sunken midface and narrow high‐arched palate are suggestive of maxillary deficiency in different planes of space, which has an impact on the size of the piriform aperture that forms the bony boundaries of the nasal passage. There can be linea alba or white lines in the buccal mucosa, torus mandibularis, buccal dentoalveolar exostosis, attrition, structural damage to teeth and dental restorations, hypersensitive teeth, masticatory muscle pain, temporomandibular disorders, and headaches stemming from sleep bruxism (SB; Palm et al., 2014; Lee et al., 2021). Co‐occurrence of SB and OSA is common and the former occurs during arousals from apneic episodes. There is an increased risk of OSA (odds ratio 1.8) in patients with SB (Balasubramaniam et al., 2014) and screening for OSA is recommended (Puvanendran et al., 2020; Kuang et al., 2022).
Oropharyngeal findings typically seen in OSA include macroglossia, erythema and/or telangiectasia of the uvula, long and redundant soft palate, and tonsillar hypertrophy. Macroglossia is often associated with the presence of scalloping along the lateral border of the tongue due to pressure against adjacent teeth. Erythema and telangiectasia of the uvula result from vibratory trauma associated with chronic snoring. Snoring‐associated intermittent hypoxia and vibratory trauma are potentially damaging, with progressive neuropathy and myopathy of the soft palate and pharyngeal dilators (Saboisky et al., 2012; Patel et al., 2018; Shah and Stål, 2022). The forces that result from repeated airway obstruction can cause stretching of the soft palate, creating redundancy and webbing of the soft palate and tonsillar pillars. Tonsillar hypertrophy is graded on a scale from 0 to 4, depending on the relation of tonsil size to the posterior tonsillar pillars: Grade 0: absence of tonsils; Grade 1: tonsils within pillars; Grade 2: tonsils extending to the posterior pillars; Grade 3: tonsils extending past the posterior pillars; Grade 4: tonsils meeting in midline. The Friedman tongue position (FTP) is a simple means to approximate obstruction at the hypopharyngeal level. FTP is the evaluation of the tongue’s position relative to the tonsils, uvula, soft palate, and hard palate and is done with the tongue in the neutral, unprotruded position. Depending on the soft palate structures visualized, patients are scored as FTP I–IV, whereby patients with a high FTP score have a higher likelihood of hypopharyngeal obstruction (Friedman et al., 2017).
Awake nasendoscopic examination of the upper airway underpins evaluation of any patient with OSA (Figures 11.4–11.9). Nasal findings that are common in patients with OSA can be structural or mucosal causes that contribute to nasal obstruction. Structural causes include nasal septal deviation, inferior turbinate hypertrophy, middle turbinate concha bullosa, and collapse of the internal or external nasal valves.
Common causes of nasal mucosa inflammation include allergic rhinitis and sinusitis. Chronic mucosal inflammation leads to the formation of nasal polyps in some patients, contributing to nasal obstruction. Adenoid hypertrophy, particularly in the pediatric population, also contributes to nasal obstruction in the nasopharynx. Nasendoscopy allows direct visualization of retropalatal (velum) and retroglossal cross‐sectional dimensions, lingual tonsils, epiglottis, and the vocal cords. Lingual tonsil hypertrophy is a common cause of retroglossal obstruction. The degree of lingual tonsil hypertrophy is graded on a scale of 0 to 4 depending on the estimated vertical thickness of the lingual tonsil visualized during nasendoscopy (Friedman et al., 2017). The shape and position of the epiglottis are also important factors in airway evaluation, whereby a tubular, redundant, or retropositioned epiglottis can predispose to obstruction.

Figure 11.4 Nasoendoscopic view of normal patent nasal airway.

Figure 11.5 Nasoendoscopic view of patient with nasal septal deviation to the right with blocked right nasal cavity and patent left nasal cavity.

Figure 11.6 Nasoendoscopic view of patient with allergic rhinitis.

Figure 11.7 Nasoendoscopic views of the retropalatal airway.

Figure 11.8 Nasoendoscopic view of the retroglossal airway. (a) No lingual tonsil hypertrophy. Laryngeal inlet visualized. (b) Lingual tonsil hypertrophy causing posterior displacement of the epiglottis. The laryngeal inlet cannot be visualized.

Figure 11.9 Nasoendoscopic view of the retropalatal airway (a) before and (b) after uvulopalatopharyngoplasty. Note the lateral and antero‐posterior widening as the palate is suspended forward.
Cephalometrics and imaging
Lateral cephalometry is routinely used in treatment planning of orthodontic patients presenting for treatment of malocclusions. Cephalometric studies of upright as well as supine patients reveal structural differences between apneic and nonapneic subjects. Cephalometric features associated with OSA are brachycephalic craniofacial configuration, short antero‐posterior cranial base length, reduced nasion‐sella‐basion angle, inferiorly positioned hyoid, retrognathic maxilla, retrognathic and micrognathic mandible, smaller than normal values for SNA and/or SNB, increased Frankfort mandibular plane angle or maxillary‐mandibular plane angle (anterior divergent face), and increased anterior lower face height.
Soft tissue features associated with OSA are narrow retropalatal and retroglossal airway spaces, thicker and longer soft palate, reduced angle between the uvula tip and posterior nasal spine, and a greater tongue mass (Miles et al., 1996; Ingman et al., 2004; Hsu et al., 2005). Snoring children and children with OSA demonstrated mildly increased ANB angle due to reduced SNB angle that were statistically significant compared to controls, but they were not clinically significant; however, airway width reduction due to adenoid enlargement was significant (Katyal et al., 2013).
Airway imaging using CBCT and magnetic resonance imaging improved diagnostic capabilities by providing accurate visualization of the airway and enabling volumetric airway measurements. The mean total airway volume, from the anterior nasal cavity to the epiglottic level, is found to be significantly smaller in patients with retrognathic mandibles and significantly correlated with antero‐posterior and vertical cephalometric variables, notably the ANB angle and anterior face height (Kim et al., 2010). The availability of CBCT acquisition of accurate 3D information provided the key to understanding the biomechanical role of the craniofacial structures in the etiology of OSA (Strauss and Burgoyne, 2008; White and Pharoah, 2008), although it is not needed for clinical decision making.
Orthodontic management
Given the complexity of multiple and overlapping etiologies, interdisciplinary team diagnosis and management of the patient with OSA involving different specialists and coordination of treatment are essential. The sleep team comprising some or all of the following members depends on the needs of the patient: endocrinologist, general dentist, neurologist, nutritionist, oral and maxillofacial surgeon, orthodontist, otolaryngologist, prosthodontist, psychiatrist, psychologist, and respiratory physician.
Treatment entails both general and specific measures to manage the varied causes and sequelae of OSA. Treatment is important in managing the patient’s general health indicators: weight, cardiovascular health, and respiratory and metabolic functions. Specific treatment decisions are based on the appropriate treatment modality for the probable cause or causes of airway obstruction during sleep. Orthodontic treatment planning takes into consideration breathing, tongue and soft palate, tonsils, and locations of potential choke points in the pharyngeal airway. Where appropriate, the orthodontic treatment plan should enlarge narrow dental arches to acceptable limits rather than extract teeth to manage crowding. Retraction of the upper and lower incisors in managing bimaxillary proclination of incisors or compensation for a Class II malocclusion with a retrusive mandible may reposition the tongue postero‐inferiorly, thereby posing a risk of compromising an existing narrow oropharyngeal airway.
A combination of clinical, craniofacial, and polysomnographic variables is used to design effective prescriptions for oral appliance treatment. Positive predictors of successful outcomes with the use of oral appliances in OSA in patients with mild to moderate OSA are AHI ≥5 to ≤30 events per hour (Schmidt‐Nowara et al., 1995; Yow, 2009; Ng and Yow, 2020), posture‐dependent or supine‐related OSA (Marklund et al., 1998), retrognathic mandible, single‐site obstruction at the retroglossal region, and an oropharyngeal lumen that is narrowed antero‐posteriorly but not laterally. Negative predictors are severe OSA with AHI >30 events per hour; BMI >30 kg/m2; multiple‐level obstructions; nonpositional‐dependent OSA; inability to protrude the mandible >5 mm; and Class III malocclusion with a large mandible (Johnston et al., 2002).
In adult patients with mild to moderate OSA, AHI ≥5 to ≤30, intraoral appliances for mandibular advancement, similar in design to functional appliances for dentofacial growth in children, have been shown to be effective in managing OSA. However, mandibular advancement splints are not recommended as first‐line treatment as they are not as efficacious as CPAP, the latter being the gold standard in treating OSA (Barnes et al., 2004; Hoekema et al., 2004; Lim et al., 2006).
Oral appliance treatment in adult OSA increases the posterior airway space by enlarging the lumen of the pharyngeal airway with increased muscular activity of the pharynx and tongue. Studies of users of mandibular advancement splints using 3D imaging and endoscopy have shown increased cross‐sectional dimensions at the multiple levels of the pharynx (Liu et al., 2000). Anterior re‐positioning of the mandible displaces the soft tissues, most importantly, the genioglossus, away from the oropharynx. The upper airway muscle activity is increased through stretching the palatoglossus and palatopharyngeus (Lowe et al., 1990).
Different studies use different indicators to denote success in OSA management. Some use AHI reduction by 50% as a success criterion, while others maintain the polysomnographic yardstick of the norm as AHI ≤5 events per hour as the criterion of cure. Even though there is computerized tomographic evidence of enlarged airway measurements due to mandibular advancement appliances (Gale et al., 2000), follow‐up polysomnography studies of the patient sleeping with the mandibular advancement splint are necessary to confirm the effectiveness in managing SDB (Kushida et al., 2005).
There are many types and designs of appliances used in SDB. The two main ones are the mandibular advancement and tongue‐retainer devices. The mandibular advancement device is the OSA appliance that is most widely used. Efficacy and outcomes of treatment with oral appliances with an anterior tongue‐retention bulb, with or without mandibular advancement, are not well reported in the literature. The magnitude of mandibular advancement is an important factor in the reduction of AHI. A monobloc or a one‐piece intraoral appliance works well in patients who can manage good mandible protrusion of at least 5 mm. For the purposes of comfort and titratability, the initial mandibular advancement can be 75% of the maximum protrusion. The desired mandibular position is achieved by using two‐piece mandibular advancement splints with telescoping connectors, similar to the Herbst appliance or the twin‐block appliance with anteroposterior screws for gradual titration of mandibular advancement. Oligodontia, with fewer than 10 teeth per jaw, is usually a contraindication to prescribing an oral appliance. There are exceptions, however, and mandibular advancement appliances can sometimes be successfully retained in edentulous patients with good dentoalveolar ridges.
Tongue‐stabilizing devices are monobloc appliances, with or without built‐in mandibular advancement. The splint incorporates an anterior bulb, in front of the upper and lower incisors, for accommodating the tongue in a forward position. The tongue‐stabilizing bulb is made from a soft polyvinyl material, which when squeezed produces negative pressure that draws the tongue forward into the retaining bulb. The best predictor of success of the tongue‐stabilizing appliance is the presence of a single‐site airway obstruction in supine‐dependent OSA (Cartwright, 1985). Studies have shown no greater efficacy of the tongue‐stabilizing device in reduction of the AHI compared to the mandibular advancement splint. In a randomized control trial, more than 90% of patients preferred to use the mandibular advancement appliance without the tongue bulb (Deane et al., 2009). Patients also preferred mandibular advancement splints to CPAP machines, even though the oral appliance is less efficacious than the CPAP device. The reasons include the effectiveness of the mandibular advancement appliance in managing mild to moderate OSA with fewer side effects and greater patient comfort (Ferguson et al., 1996). Furthermore, the oral appliance is small, which makes it easy to carry on journeys, and it does not need to be powered by electricity. The added incentive with an intraoral device is the lack of external visibility and the fact that it does not generate noise. There are none of the claustrophobic and cumbersome straps and tubes of the CPAP machine to deal with.
Oral appliance treatment does have its drawbacks. Soreness of the teeth, occlusion changes, and facial muscle and temporomandibular joint pain are commonly reported in the first few hours of waking. Long‐term side effects occur after two or more years of oral appliance wear, and these include dental and skeletal changes. They present as reduction of overbite and overjet, proclination and retroclination of the lower incisors and upper incisors, respectively, and about 26% of patients experience irreversible changes in their occlusion. Oral appliances with greater advancements of the mandible have a greater impact on the skeletal system; there is downward and forward positioning of the mandible with increased anterior lower face height (Clark et al., 2000). In a report on the practice parameters of snoring and OSA, the American Academy of Sleep Medicine recommends fitting and follow‐up of oral appliances by trained dental practitioners in dental sleep medicine. Follow‐up overnight polysomnography is necessary to establish efficacy of the oral appliance with long‐term monitoring of OSA signs and symptoms, dental and periodontal health, as well as the occlusion (Kushida et al., 2006).
Surgical management
Even though CPAP therapy remains the gold standard for management of OSA, adherence to treatment remains a challenge. Up to 83% of patients are unable to use the CPAP device for more than four hours per night (Weaver et al., 2008). As discussed in the previous section, not all patients are suitable for nor respond fully to oral devices. In these cases, surgery is an option.
Surgical procedures for OSA can be broadly divided into site‐specific airway procedures that target the nose, oropharynx, and/or hypopharynx versus global airway procedures that seek to address the entire length of the upper airway, such as maxillo‐mandibular advancement (MMA) or bariatric surgery. The surgical management strategy of OSA can be device based, whereby surgery is performed to complement and facilitate device use (CPAP or oral appliance), or for surgical cure. The decision on which surgical management strategy to adopt is dependent on the following factors: the patient’s willingness to continue using CPAP/oral appliance; identification of factors that contribute to poor device comfort and compliance; and the physician’s overall assessment of the key contributing factors to the patient’s OSA.
A device‐based strategy can be adopted in patients who have poor compliance with CPAP or an oral device due to nasal obstruction. In a study that evaluated various physical, psychological, device‐related, and socioeconomic factors that influenced CPAP adherence, nasal obstruction and CPAP pressure levels were found to be key contributing factors to poor adherence (Archbold and Parthasarathy, 2009). Nasal surgeries like septoplasty, inferior turbinoplasty, concha bullosa excision, nasal valve suspension, endoscopic sinus surgery, and polypectomy can improve nasal airway patency. A meta‐analysis evaluating the effect of nasal surgery on CPAP device use and therapeutic treatment pressures reported reduction of CPAP pressures from 11.6 ± 2.2 to 9.5 ± 2.0 cmH2O after nasal surgery. In addition, 89.1% of patients who were not using CPAP prior to nasal surgery accepted, adhered to, or tolerated CPAP after surgery and device usage increased from a mean of 3.0 ± 3.1 to 5.5 ± 2.0 hours (Camacho et al., 2015). Tonsillectomy and/or adenoidectomy are performed in patients with severe adenotonsillar hypertrophy, with the same overall aim of improving upper airway patency and reducing CPAP pressure requirements. Partial epiglottidectomy is performed in patients with a floppy closing door epiglottis who experience difficulty with CPAP due to displacement of the epiglottis against the laryngeal inlet by airway pressure.
Multilevel soft tissue surgery to address all obstructions in the upper airway is required when the aim is device‐free surgical cure. The overall success rate of multilevel soft tissue surgery has been reported to be between 61.6% and 66.4% (Lin et al., 2017). DISE allows examination of the dynamic status of the upper airway during sleep to determine the sites and pattern of airway obstruction. With precise identification of the sites and types of obstruction, surgery can be tailored to address them such that surgical success rates are maximized (Lee et al., 2012; De Vito et al., 2018).
In adult patients with mid‐palatal suture maturation, surgically assisted palatal expansion (SARPE) and miniscrew‐assisted rapid palatal expansion (MARPE) are recently introduced options in improving nasal function by widening the maxilla. Increased upper airway volume by palatal expansion is well established (Calvo‐Henriquez et al., 2021). The change in anatomy reduces airflow speed and negative pressure effects on the pharyngeal airway. This correlates with reductions in AHI and ODI (Iwasaki et al., 2020). The evidence for long‐term efficacy is yet to be established.
MMA surgery is a strategy to establish multilevel upper airway patency (Yu et al., 2020; Figure 11.10). A meta‐analysis of 518 patients from 45 studies showed MMA surgery to be effective in treating OSA. Surgical success criteria were reductions in AHI ≥50% with fewer than 10 events per hour. Post‐MMA AHI ≤5 indicated cure of OSA for the patient. Surgical success in managing the severity and the cure rate were 85.5% and 38.5%, respectively. The strongest predictive factor for a surgical cure was AHI ≤60 (Zaghi et al., 2016). Using dynamic assessment with DISE, the anatomical structures of the upper airway that stabilized after successful MMA interventions were the lateral pharyngeal wall, soft palate, and tongue base. Individuals with the largest improvements in respiratory parameters of AHI and ODI had the greatest reduction in lateral pharyngeal collapsibility (Liu et al., 2015).

Figure 11.10 (a) Lateral cephalogram before maxillo‐mandibular advancement (MMA) surgery. (b) Lateral cephalogram after MMA with counter‐clockwise rotation of the maxillo‐mandibular complex and multilevel enlargement of the upper airway
Conclusion
The causes of SDB are multifactorial. The etiologies and pathogenesis are neither well defined nor completely understood. Although the likely anatomical areas and obstructing structures in the nose and choke points at different levels of the pharynx are clinically identifiable, their roles in SDB may not always be one of direct cause and effect. There are nonanatomical contributors to the heterogeneous disorder to consider; that is, muscle reactivity, loop gain, and arousal threshold. Identification of the sleep phenotypic traits is necessary for a precise treatment approach.
In children, the important consideration in management is to rule out the common causes of nasal and/or nasopharyngeal obstructions. Although surgical management by tonsillectomy and adenoidectomy is the mainstay in treatment of childhood OSA, treatment with dentofacial orthopedic appliances can alleviate compromised upper airways in growing patients where the adenotonsillar size is incompatible with dentofacial structure for upper airway function. Without proper diagnosis and treatment, approximately one‐third of children with mild SDB will progress in two years or more to a more severe form of the condition. The rationale behind the approach to treatment is either to increase the volume of the hard tissue “container” to accommodate the enlarged soft tissue contents, or to remove the contents that have become too large for the container.
Orthodontic treatment protocols include management of dentofacial morphometric risk factors to alleviate OSA in the short term by widening the maxilla and protraction of retruded maxilla and mandible. Long‐term studies are needed to provide an evidence‐based cure for OSA. For some patients OSA is not curable, but treatment is possible to manage the severity and attendant medical comorbidities. Comprehensive interdisciplinary management is necessary to address all possible causes, structural as well as nonstructural.
Caution should be exercised in certain surgical procedures that are known to cause OSA, such as pharyngoplasty to improve speech in cleft lip and palate patients, and orthognathic setback procedures of the maxilla or mandible to manage dentofacial esthetics. There is evidence to substantiate that these surgeries can permanently reduce the caliber of the upper airway. It is recommended to screen all patients for SDB before subjecting them to procedures that can reduce airway patency.
In adults, the first‐line management strategy is to rule out nasal obstructions and the use of the gold standard therapy with CPAP to pneumatically splint the airway open. Unfortunately, the lack of adherence to CPAP therapy is high. Consequent to that, there is a plethora of other airway‐opening strategies with positional sleep devices, mandibular advancement appliances, tongue stabilizers, and surgical procedures. In severe cases when all types of treatment to establish a functionally patent upper airway are exhausted, the obstructed upper airway is bypassed with tracheostomy.
Patients with SDB may have associated excessive daytime sleepiness and associated medical comorbidities that adversely affect the cardiovascular and cerebrovascular systems as well as metabolic and neurocognitive functions. Evaluation by an interdisciplinary team trained in sleep medicine is vital for orthodontic or orthognathic patients presenting with SDB for treatment. Identification of patients at risk of SDB before orthodontic and orthognathic treatment prevents compromising of a narrow upper airway that worsens a collapsible upper airway. Multidisciplinary collaboration adds to the decision‐making process in treatment planning. The treatment plan to manage dentofacial esthetics and occlusion should include the maintenance of or improvement to the patency of the upper airway. The patient with SDB and medical comorbidities requires multiple treatment considerations from the cardiologist, endocrinologist, neurologist, orthodontist, oral and maxillofacial surgeon, otorhinolaryngologist, pediatrician (for a child), psychiatrist, psychologist, and respiratory medicine physician. Orthodontic treatment in patients with SDB, dentofacial deformities, and malocclusion should be done in close collaboration with a sleep medicine team.
A collaborative interdisciplinary approach is essential to optimize the care of patients with SDB. The challenge for the orthodontist or dentist is in recognizing patients who are at risk of a collapsible upper airway to initiate early investigations and interventions. Orthodontic patients can be easily screened using a combination of family history, sleep history, clinical assessment, and imaging (Boxes 11.1 and 11.2; Figures 11.11 and 11.12).

VIDEdental - Online dental courses
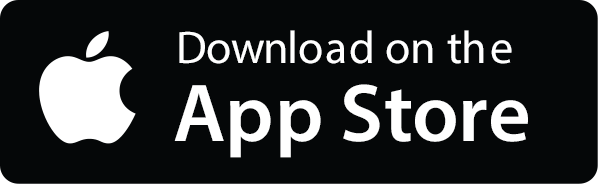
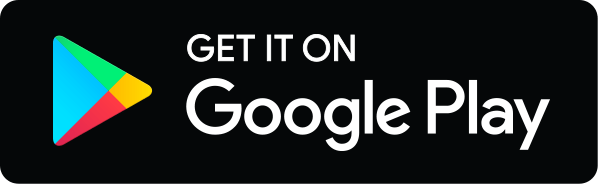