Abstract
Objectives
Poor interfacial adhesion between the fibers and resin matrix in the ultra high molecular weight polyethylene (UHMWPE) fiber reinforced composites (FRCs) is the main drawback of the composites. This study aims to evaluate the effect of corona and silane surface treatment of the fibers on the mechanical properties of the UHMWPE FRCs.
Methods
UHMWPE fibers were exposed to corona discharges for different periods of time (0 s, 5 s, 7 s). The surface characteristics of the UHMWPE fibers were investigated by attenuated total reflectance Fourier transformed infrared spectroscopy (ATR-FTIR), atomic force microscopy (AFM), scanning electron microscopy (SEM) and nanoindentation technique. The flexural strength and flexural modulus of the FRCs made of the treated fibers were determined on 2 mm × 2 mm × 25 mm specimens. The fracture toughness (the critical stress intensity factor, K IC ) of the composites was also evaluated using a three-point single edge notch beam (SENB) bending technique. Statistical analysis of the data was performed with ANOVA and the Tukey’s post-hoc test. The fiber-resin interface and the fracture surface were investigated using SEM.
Results
The change in the surface mechanical properties and chemistry of the corona treated UHMWPE fibers were monitored. The fibers exposed to corona for 5 s showed higher surface nanohardness. In the FRCs, the specimens reinforced with 5 s corona treated silanized fibers showed higher mechanical properties (flexural modulus, flexural strength, and fracture toughness), SEM images revealed a better adhesion between the resin and fibers after 5 s fiber corona treatment and silanization.
Significance
Corona and silane treatment of UHMWPE fibers provide dental FRCs with improved mechanical properties.
1
Introduction
Development of fiber-reinforced composites (FRCs) have offered dental researchers the possibility of making resin-bonded, mechanically and esthetically good, and metal-free restorations for teeth replacement . Variety of dental applications, such as bridges, splints, posts, space-maintainers, orthodontic retainers, denture bases, clasps and connectors, and implant prostheses made of FRCs have successfully been used in dental practice providing acceptable mechanical properties . In general, in a FRC, fibers are the main load-carrying members, while the surrounding matrix acts as a load transfer medium and protects the fibers from environmental harms . The mechanical properties of FRCs are dependent upon the mechanical properties of each component, quality of impregnation of fiber with resin, adhesion between the fiber and matrix, quantity of fibers in the matrix resin, the fiber volume fraction, fiber architecture, and the fiber type . UHMWPE fibers in comparison with other plastic materials are found to have high impact resistance, toughness, and tensile strength. The fibers are also light weight, corrosion and wear resistant . Adhesion of UHMWPE fibers to the matrix resin is, however, difficult because the UHMWPE is non-polar in nature with low surface energy . To provide a good adhesion between the UHMWPE fibers and the polymer matrix, the surface of the fibers has been modified using different methods such as chemical modification , chemical grafting , corona discharging , oxygen plasma , high energy laser , UV , and gamma irradiation . Improving the adhesion performance of UHMWPE may results in improved dental FRCs. Corona discharge, is a process by which ionized gas species are formed around an active high potential electrode due to the electrical current . In corona treatments of polymers the ionized species transfer their charge to the polymer surface resulting in increase in surface energy by introducing polar groups on the surface. The corona discharge may also change the surface roughness . The process can impart hydrophilicity to polymer surfaces which may improve the surface affinity and sticking strength with some hydrophilic polymers . It can also improve the bonding characteristics of materials by raising the surface energy, wettability, oxygen to carbon ratio, number of functional polar groups on the surface, and specific surface area leading to enhanced adhesive capabilities, increase in the delamination force and improvement in the fracture toughness and flexural properties . Silane treatment, on the other side, is a well-known method for improvement of surface wettability and interfacial adhesion between matrix resin and OH-covered substrates .
Therefore, the combination of these two surface modification methods may result in FRCs with improved properties. This study, consequently, designed to test the hypothesis that the double surface treatment of UHMWPE fibers improves the mechanical properties of an experimental dental FRC containing the fibers as reinforcing phase and Bis-GMA/TEGDMA as matrix.
2
Materials and methods
2.1
Materials
Materials used in this study are presented in Table 1 .
Country | City | Company | Special properties | Role | Name |
---|---|---|---|---|---|
Germany | Krefeld | Evonik | – | Resin monomer | 2,2-Bis-[4-(methacryloxypropoxy)-phenyl]-propane (Bis-GMA) |
Germany | Krefeld | Evonik | – | Diluent monomer | Triethyleneglycol dimethacrylate (TEGDMA) |
Germany | Munich | Sigma–Aldrich | – | Activator | N-N′-dimethyl aminoethyl methacrylate (DMAEMA) |
Germany | Munich | Sigma–Aldrich | – | Initiator | Camphorquinone (CQ) |
China | Shanghai | Surrey Hi-Tech | Roving continuous (SP1200/320) Diameter ≈20 μm * |
Reinforcing fibers | Ultra-high-molecular-weight polyethylene (UHMWPE) |
USA | Pittsburgh | Acros organics | 98% Purity | Silane coupling agent | 3-(Trimethoxysilyl)propyl methacrylate (γ-MPS) |
2.2
Methods
2.2.1
Surface corona treatments of UHMWPE fibers
UHMWPE fibers were treated for 5 s and 7 s under atmospheric pressure air corona discharge using SpotTEC corona power Generator (Tantec, Lunderskov, Denmark, 6.5 kV/25 kHz and 550 W). The distance between electrode and UHMWPE fiber surface was 5 cm.
2.2.2
AFM and nanoindentation analysis
AFM imaging analysis was performed (SPM Dualscope/Rasterscope C26, DME, Copenhagen/Herlev, Denmark) to study the surface topography and roughness of the fibers before and after corona treatment. Hardness ( H i ) of the fibers was determined using nanoindentaion technique (CSM, Peseux, Switzerland, indentor type: Berkovich, indentor material: diamond, max depth: 500 nm, loading and unloading rates: 1000 nm min −1 ). The elastic modulus of the specimen in depth-sensing indentation techniques, used in nanoindentation test, is determined from the slope of the unloading of the load-displacement response, E i . Vickers hardness was also calculated from the H i .
2.2.3
Fourier-transform infrared spectrometer (FT-IR)
A FTIR spectrometer (EQUINOX55, Specac golden gate, ZnSe prism, Bruker, Germany) was used in order to identify functional groups formed on the fibers after corona and silane treatments. The spectra were collected in the wavenumber range of 4000–400 cm −1 , using attenuated total reflectance technique (ATR-FTIR). In this technique the infrared beam is directed into a crystal of relatively high refractive index (ZnSe) which is in contact with the fibers. Multiple reflections of the beam in the crystal collects the spectrum of the intimate fibers.
2.2.4
Silanization of UHMWPE fibers
The chemical surface modification of UHMWPE fibers was carried out using a 2 (wt/vol)% γ-MPS in ethanol/distilled-water (70/30 wt/wt, pH adjusted at 3.8 using acetic acid). Having the silane pre-hydrolyzed for 1 h, the fibers were immersed in the solution and left for 1 week at room temperature to dry.
2.2.5
Composite preparation and mechanical measurements
The composites were classified in 5 groups ( Table 2 ). Group 1, group 2 and group 3 were prepared to evaluate the effect of corona exposure time. All these three groups were made of 10 bundles for flexural test and 20 bundles for fracture toughness test. As the volume of the fracture toughness test mold was two times of the flexural test, twice fibers were inserted in the fracture toughness molds to keep the fiber content constant in both tests. The fiber corona treatment time was 0 s, 5 s and 7 s for group 1, group 2 and group 3, respectively. The fibers in these three groups were silanized after corona treatment. Group 4 and group 5 were made of 8 fiber bundles for flexural test and 16 fiber bundles for fracture toughness test, corona treatment time was 5 s for both of them. The fibers in group 4 were silanized but the fibers in group 5 received no silane treatment to evaluate the effect of silane treatment.
Groups | Aim of study | Fibers corona treatment time (s) | Silanization of fibers with γ-MPS | Number of fiber bundles for (flexural test) | Number of fiber bundles for (fracture toughness test) |
---|---|---|---|---|---|
1 | Effect of corona exposure time | 0 | Yes | 10 | 20 |
2 | Effect of corona exposure time | 5 | Yes | 10 | 20 |
3 | Effect of corona exposure time | 7 | Yes | 10 | 20 |
4 | Effect of silane treatment | 5 | Yes | 8 | 16 |
5 | Effect of silane treatment | 5 | No | 8 | 16 |
2.2.5.1
Flexural strength and flexural modulus
0.5 wt% CQ and 0.5 wt% DMAEMA, as photo-initiator system, were mixed with the matrix resin (Bis-GMA/TEGDMA, 70/30 wt/wt). The fibers were then impregnated with resin and were cut with a sharp surgical blade and placed manually into the mold in unidirectional (UD) position. 5 bar shaped specimens were made of each composite group according to Table 2 , using a metal mold with the dimensions of 25 mm × 2 mm × 2 mm. The mold was placed on a glass slide and manually filled with the pre-impregnated fibers. A second glass slide was placed on and pressed against it. The composite was cured with a LED dental light source (L.E. Demetron 1, SDS/Kerr, Orange, CA, USA) with power density of 600 mW cm −2 for 40 s in three consecutive points, producing a partial overlapping. After curing, the specimens were polished with abrasive paper 600 and 1000 grits and stored in distilled water at 37 °C (±2 °C) for 24 h. Afterwards, they were submitted to a three point bending test with a universal testing machine (STM 20, Santam, Tehran, Iran) with a crosshead speed of 1 mm min −1 . The flexural strength (FS) was calculated in (MPa) using the following formula:
where p is the maximum load ( N ); L is the span length (mm); b is the width of the specimen (mm) and h , the height (mm). The flexural modulus was calculated from the slope of the initial linear region of stress–strain curve.
2.2.5.2
Fracture toughness
To determine the fracture toughness ( K IC ), rectangular bar single-edge notch beam (SENB) specimens ( n = 5) were fabricated in a 5 mm × 2 mm × 25 mm stainless steel mold. The mold was filled with impregnated fibers according to Table 2 and cured using the same procedure as the flexural specimens. A 2.5 mm notch was then cut at the center of each specimen using a 0.6 mm thick diamond wheel cutting machine (Mecatome T 201 A, Oxford, UK). A razor blade was then pressed on the notches to provide a sharp crack. The bending fracture test was performed at a cross-head speed of 1 mm min −1 using the universal testing machine and the fracture toughness (critical stress intensity factor, K IC ) was calculated according to the following equation :
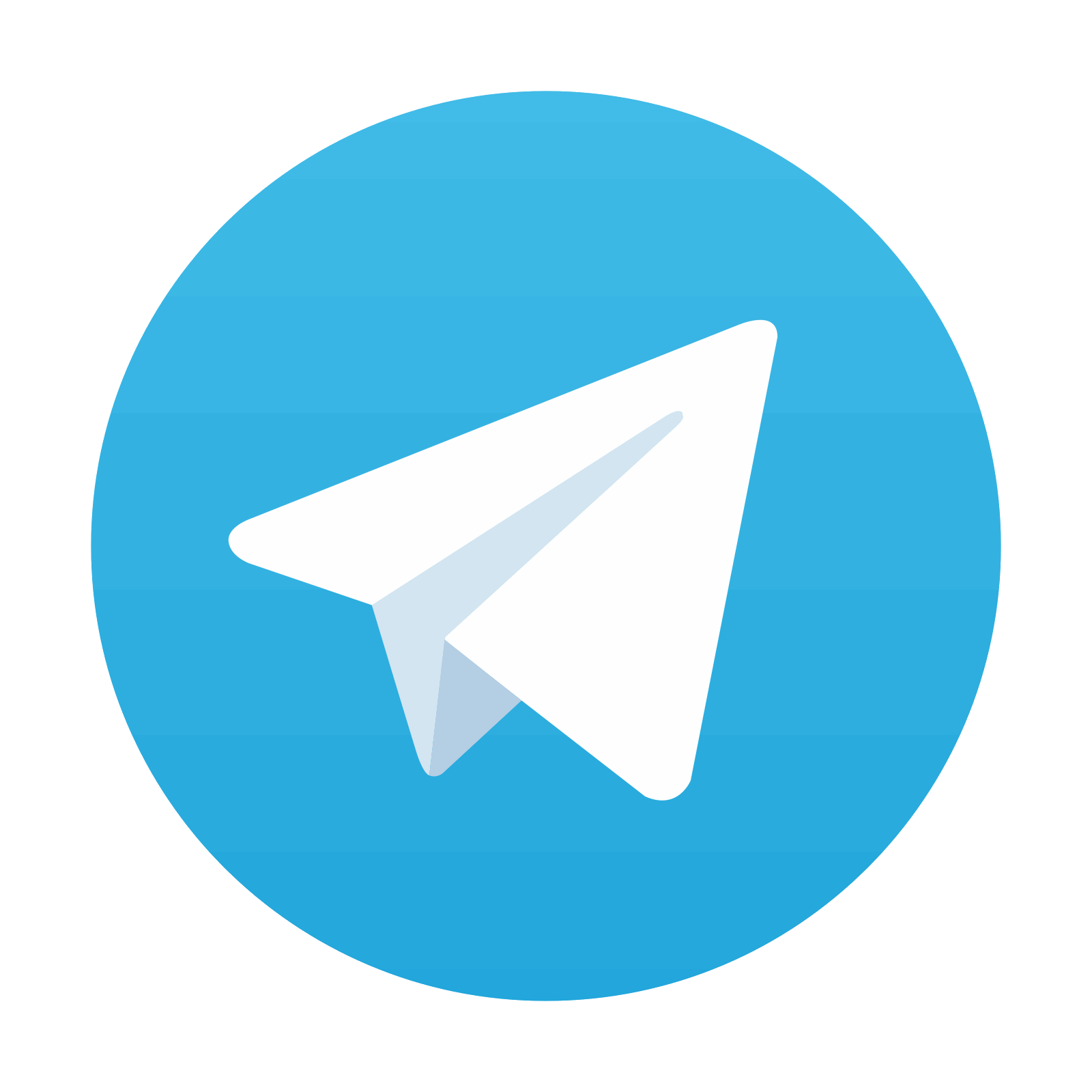
Stay updated, free dental videos. Join our Telegram channel

VIDEdental - Online dental courses
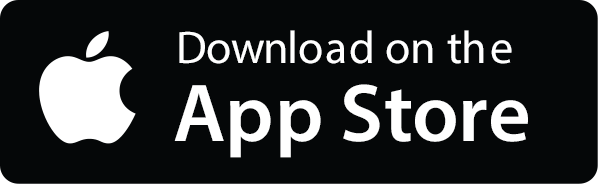
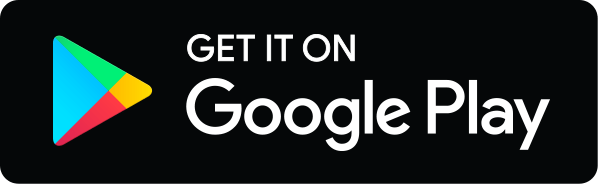