Key points
- •
The optimal evaluation and treatment of the head-injured patient is predicated on initially following established Advanced Trauma Life Support principles and preventing secondary injury.
- •
Once specific injuries have been identified, maintenance of cerebral perfusion and oxygenation are the keys to maximizing patient outcomes.
- •
When significant mass lesions are identified or intracranial pressure elevations become refractory to medical intervention, surgical intervention is necessary.
Introduction
Rapid identification, classification, evaluation, and treatment of the head-injured patient is crucial to avoiding secondary injury and potentiation of the initial neurologic insult. This article discusses basic cranial anatomy, classification of head injury, triage decision making, and management concepts for the head-injured patient.
Introduction
Rapid identification, classification, evaluation, and treatment of the head-injured patient is crucial to avoiding secondary injury and potentiation of the initial neurologic insult. This article discusses basic cranial anatomy, classification of head injury, triage decision making, and management concepts for the head-injured patient.
Cerebral anatomy
The skull is composed of more than 25 identified bones however, the cranial compartment is formed primarily by the frontal, parietal, temporal, occipital, and greater wings of the sphenoid bones. The cranial vault is an enclosed space best conceptualized as a rigid, nonexpandable box, subdivided into separate intracranial spaces partitioned by bone as well as dense connective tissue planes. The tentorium cerebelli is a fibrous connective tissue band that separates the intracranial space into supratentorial and infratentorial compartments; the supratentorial space houses the cerebral hemispheres while the infratentorial space contains the cerebellum. The brainstem passes through the tentorial incisura inside the cranial compartment ( Fig. 1 ).
The intracranial space is further divided into the anterior, middle, and posterior fossae, which contain the frontal lobes, temporal lobes, and cerebellum with brainstem, respectively. The right and left supratentorial space is separated by the falx cerebri, nearly identical in composition to the tentorium cerebelli. The brain is divided into the frontal, parietal, occipital, and temporal lobes as well as the midbrain, pons, medulla, and cerebellum ( Fig. 2 ).
Within the brain are ventricles filled with cerebrospinal fluid (CSF), which is produced by the choroid plexus within the ventricular system at a rate of approximately 0.3 mL/min (18–20 mL/h). CSF circulates from the lateral ventricles, to the foramen of Monro, the third ventricle, the aqueduct of Sylvius, the fourth ventricle, and then out the foramen of Magendie or Luschka. CSF aids in central nervous system (CNS) homeostasis, allows the brain to “float” in the intracranial cavity, and acts as a liquid suspension system, damping normal arterial pulsations. Pathophysiologic obstruction of flow or resorption will result in increased intracranial pressure and hydrocephalus.
The brain receives its blood supply from a right and left internal carotid artery that penetrate the petrous bone, and join with the basilar artery via the posterior communicating artery at the base of the brain, forming the circle of Willis and its associated branches. The middle cerebral artery provides blood supply to the majority of the lateral cerebral hemispheres as well as the base of the temporal lobes. The anterior cerebral arteries are connected by the anterior communicating artery, and provide blood supply to the medial surfaces of the cerebral hemispheres in addition to a medial strip of brain tissue extending from anterior to posterior ( Fig. 3 ). The posterior cerebral arteries provide blood supply to the occipital lobes and a portion of the posterior temporal lobes. The vertebral arteries merge to form the basilar artery, and provide blood supply to the brainstem and cerebellum.
Superficial venous drainage of the brain occurs via (1) cortical bridging veins to the superior sagittal sinus, (2) the vein of Labbe to the superior petrosal sinus, or (3) the basal vein of Rosenthal along the inferomedial border of the temporal lobe to the vein of Galen. Deep drainage of the brain occurs via the deep internal cerebral veins to the vein of Galen, which then empties into the straight sinus. The straight and superior sagittal sinuses combine to form the confluence of the sinuses located at the level of the inion posteriorly; these drain into the transverse and then sigmoid sinuses before emptying into the internal jugular veins. Avoidance of central access to the internal jugular vein ipsilateral to an injured cerebral hemisphere is important in preventing venous occlusion and outflow restriction.
Cerebral physiology
An important physiologic concept is that of intracranial pressure, which is measured in mm Hg or cm H 2 O; normal values are less than 20 mm Hg. It is important to remember that fluids move from areas of high pressure to low pressure. Intracranial contents include brain tissue, blood (arterial, capillary, venous), and CSF. Addition of a mass lesion to the intracranial space (tumor, hematoma, edema) will cause an increase in intracranial pressure because the skull is rigid and nonexpandable. As the mass lesion increases in size, so does the intracranial pressure; as a result CSF is squeezed out of the intracranial compartment via its normal channels which results in reduction of the intracranial pressure (ICP). If the mass continues to expand, venous blood in addition to CSF will be squeezed from the intracranial compartment, keeping ICP within a normal range. If the mass lesion continues to expand, this process will continue to an inflection point that, once passed, sees large increases in ICP with small increases in lesion volume ( Fig. 4 ). As ICP continues to climb, brain tissue will herniate (uncal, subfalcine, or transtonsillar) from its normal anatomic location, causing compression or displacement of delicate neurologic structures and blood vessels resulting in additional neurologic injury and possibly death. If the pressure is high enough, cerebral perfusion will become impaired because of the pressure gradient required to circulate blood into the intracranial compartment.
The noninjured brain is able to regulate and maintain a near constant blood flow despite changes in blood pressure and vascular volume. This process is termed cerebral autoregulation, which is vital to maintaining adequate cerebral perfusion and, thus, oxygenation. Cerebral perfusion pressure (CPP) is defined as the mean arterial pressure (MAP) minus the ICP. Normal values are approximately 65 to 75 mm Hg.
The traumatized brain is unable to autoregulate cerebral blood flow, thus hypotension may result in cerebral ischemia whereas hypertension will result in perfusion increases that may cause hemorrhage, edema, or both ( Fig. 5 ). For the intubated head-injured patient ICP monitors are placed into the tissue parenchyma or a drain (termed ventriculostomy) is placed into the ventricular system. These monitors allow second-to-second monitoring of ICP and, thus, CPP when measuring the MAP. Medical therapy can be instituted with a goal CPP target of 65 to 75 mm Hg in the head-injured patient. Ventriculostomies have the added advantage of allowing CSF diversion, and thus direct lowering of ICP in addition to monitoring of intracranial pressure.
Classification of head injuries
Head injuries can be categorized in many ways, including: (1) neurologic status as measured by the Glasgow Coma Score (GCS); (2) whether the injury is open or closed; and (3) whether the injury is a result of a high-velocity or low-velocity impact. Penetrating head injuries may result from low-velocity or high-velocity projectiles and course through a lobe(s) of the brain, across the ventricles (transventricular), or across the supratentorial and infratentorial compartments (bicompartmental) ( Box 1 ).
-
Glasgow Coma Score
- •
14–15: mild head injury
- •
9–13: moderate head injury
- •
3–8: severe head injury
-
Closed Head Injury
- •
Low velocity
- •
High velocity
-
Open Head Injury
- •
Blunt trauma
- ○
Low velocity
- ○
High velocity
- ○
- •
Penetrating trauma
- ○
Low velocity
- ○
High velocity
- ○
Lobar
- ○
Transventricular
- ○
Bicompartmental
- ○
Head injury is most commonly described in terms of mild, moderate, or severe based on the patient’s GCS. It may also be described in terms of open versus closed injuries and further subdivided from there. GCS scores have high interrater and intrarater reliability and can be used for prognostication.
Specific patterns of injury may be found commonly between separate categories of head injury. The most common head-injury patterns and their radiologic presentation are discussed here.
Skull fractures are frequently encountered in the head-injured patient. It is imperative to review all studies obtained during imaging, including the bone windows from computed tomography (CT) scans. Fractures of the skull base may present with raccoon eyes, Battle’s sign, and rhinorrhea or otorrhea. Traumatic CSF leaks typically resolve spontaneously and may require brief CSF diversion with a ventriculostomy. Fractures of the skull base are frequently associated with maxillofacial fractures. When the energy transfer is significant, severe fragmentation of the anterior cranial fossa floor is common. Skull-base fractures extending to the anterior clinoid process or foramen lacerum, which houses the internal carotid artery, can result in dissection and stroke. Fractures that cross the draining dural sinuses are to be identified and thoughtfully considered. Surgical exposure in these areas may lead to catastrophic bleeding and worsening of the initial neurologic injury, or possibly result in death from exsanguination.
Parenchymal injuries involve injury to the brain tissue proper and are visually evident on imaging studies (CT and magnetic resonance imaging). Contusions, a common sequela of head trauma, result from rupture of the cerebral capillaries owing to brain impact against the inner skull as well as the soft brain surfaces rubbing over the rough skull base. Contusions at the site of impact are termed “coup” injuries, whereas “contrecoup” injuries are seen opposite the site of impact. Contrecoup injuries result from low pressure opposite the site of impact caused by sudden brain shift.
Intraparenchymal hemorrhages (IPH) result from active bleeding within the brain substance as a result of trauma. IPH may be punctate or large, and can quickly expand, resulting in elevated ICP. Hemorrhages can extend into the ventricular system, and lead to obstructive hydrocephalus and increased ICP. Patients with significant intraventricular hemorrhage (IVH) often require placement of a ventriculostomy for CSF diversion and ICP monitoring.
Violent changes in velocity (velocity is defined as speed and direction of motion) may result in diffuse axonal injury (DAI). The gray matter containing neuronal cell bodies rests on white matter composed of myelinated axons and glia. These tissues have different inertial moments that allow the development of shear forces during trauma, resulting in axonal stretch and rupture. DAI is typically seen at the gray-white junction, corpus callosum, centrum semiovale, and pons, and is treated nonoperatively.
The most common finding after head injury is acute blood in the subarachnoid space, termed traumatic subarachnoid hemorrhage (tSAH), which can be found with relatively minor impacts as well as in severe head injury. The effects are generally self-limited; however, extensive tSAH may result in communicating hydrocephalus, seizure, or even vasospasm of the large to medium-sized cerebral arteries.
Vascular injuries are not as common as brain tissue trauma but can have devastating consequences when present. Arterial dissection results from tearing of the intima and can result in complete or partial vessel occlusion. These injuries are most commonly seen in the internal carotid and vertebral arteries. Complete occlusion commonly results in significant ischemic infarction unless the circle of Willis is intact, allowing perfusion from alternative arterial pathways. Partial occlusions with an intimal “flap” result in thromboembolic events caused by platelet aggregation and intermittent dislodgment. Dissections are common with whiplash injuries and in fractures through the skull base.
Disruption or transection of epidural arteries caused by skull fractures will result in the formation of an epidural hematoma. The dura tightly adheres to the inner table of the skull but can be displaced by systolic arterial pressure and result in an expanding epidural hematoma (EDH). If untreated, the hematoma will often result in compression and displacement of vital brain tissue, resulting in loss of consciousness and death. The middle meningeal artery is classically described in this situation ( Fig. 6 ).
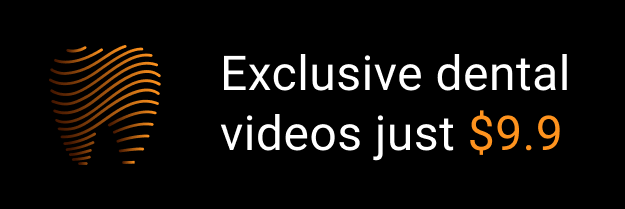