20
Transplantation Medicine
Sharon Elad, DMD, MSc
Marie Laryea, BSC, MDCM, FRCP (Int Med, GI)
Noam Yarom, DMD
INTRODUCTION
Transplantation is the treatment of choice for the restoration of function in end-stage organ disease. Innovative surgical techniques have played a major role in enhancing the success of organ transplants, leading to improved graft and patient survival. Advances in the biology of organ preservation have led to improved cold storage of the solid organs, improving the overall quality of the transplanted organs and diminishing the damage incurred by warm ischemia, allowing optimization of the medical conditions of the donor and the recipient. Furthermore, insights into the biology of immune responses to transplanted tissues have aided in the development of immunosuppressive techniques to prevent the rejection of the organ transplant while minimizing the morbidities associated with chronic immunosuppression. The main limitation to even greater use of transplantation as a treatment modality for end-stage organ damage remains the shortage of organ donors.
Attempts at organ and bone marrow transplantation date back to the 1800s. Dr. Joseph Murray performed the first successful human renal transplantation between identical twin brothers in 1954. This procedure was well tolerated, as there was no rejection by the genetically identical recipient. The first successful allogeneic (not genetically identical) transplantation was a kidney transplant between fraternal twins performed in 1959, in which the recipient was “conditioned” (immunosuppressed to prevent rejection) by total body irradiation. In 1962, a successful cadaveric donor renal transplantation was achieved, and in 1966, a pancreas transplantation was successfully performed. In the following year, the first human liver transplantation was performed, resulting in a 13-month survival. In the same year, a heart was transplanted. In 1968, a genetically related bone marrow transplantation (today referred to as a hematopoietic stem cell transplantation [HSCT]) was performed, and in 1973, a genetically unrelated HSCT was performed. Lung transplantation has also been performed both as a single procedure and in combination with a heart transplantation. The first heart–lung transplantation in the United States was done in 1981, and the first ever single-lung transplantation was performed in Canada in 1983. Other organs that have been successfully transplanted include the small bowel, skin, various limbs, face, and components of the human eye.
In past decades, significant advances—including tissue typing and the development of immunosuppressive medications and medication regimens—have increased the success of transplantation. Overall, long-term patient survival has also significantly increased over the past 30 years, in both solid organ and HSCT recipients. Most transplant clinicians consider the discovery of the immunosuppressive agent cyclosporine (CSA) to be the most significant advance in transplantation medicine. This medication was approved for use in 1983. Both solid organ and non–solid organ transplantations are becoming more routine throughout the world. In November 2013, over 120,000 patients in the United States alone were waiting for a solid organ transplant. In 2012, 28,052 solid organs were transplanted, approximately the same number as was seen in 2005, and in 2019, 39,719 transplants were performed (Table 20-1).1
Table 20‐1 Waiting lists and transplanted solid organs (2019) in the united states.
Source: Based on Organ Procurement and Transplantation Network (OPTN). https://optn.transplant.hrsa.gov/data/view-data-reports/national-data/#. Accessed March 24, 2020.
Organ | Awaiting Transplants | Transplants Performed | Total Transplants Performed to Date |
---|---|---|---|
All* | 112,402 | 39,719 | 804,607 |
Liver | 12,698 | 8,896 | 174,582 |
Kidney | 94,552 | 23,401 | 473,811 |
Lung | 1,275 | 2,714 | 41,529 |
Heart | 3,669 | 3,552 | 76,493 |
* Note that these numbers do not equal those cited in the text, since other organs, including the small intestine and pancreas, are not included in this table. All transplants up to end of 2019.
As the process of solid organ transplantation expands, there is a continued need to increase the supply of organs suitable for transplantation. The limitation of available donor organs will hopefully become less of an issue with increased awareness of organ donation and perhaps with collaborative sharing initiatives, alternative organ procurement methods, including xenografts or stem cell–derived tissue, or printed organs.
The likelihood of a dentist having to treat a transplanted patient is increasing, as many of these transplant recipients resume a normal life after transplantation and many achieve long-term survival beyond 10 years. This chapter reviews different aspects of transplant medicine pertinent for the oral health professional.
CLASSIFICATION
Most transplant clinical classification systems employ both the type of tissue transplanted and the genetic relationship of the tissue to the recipient. It is extremely important for the dental clinician to know exactly what type of transplantation was performed, as both the management and the prognosis are intimately related. This will become evident later in this chapter.
Some authorities broadly divide transplantations into solid organ/tissue transplant or HSCT. Virtually all solid organs/tissues have been transplanted, including heart, lung, kidney, liver, stomach, intestine, pancreas, skin, tendons, nerves, veins, and eye components, as well as composite tissue transplants, including the face and the limbs. HSCT is another type of transplantation frequently used to treat various hematologic and some nonhematologic malignancies, as well as other disorders. HSCT uses either an autologous “self” donor graft or a “nonself” donor (allogeneic graft).
Classification of transplants can also be based broadly on the genetic relationship of the recipient to the donor. For the purpose of this chapter, the transplanted cell, tissue, or organ is referred to as the graft. A transplant to and from one’s self (autograft) is known as an autologous transplant. A transplantation from an identical twin (identical genetic makeup) is known as an isograft, with the process of this type of transplantation known as isogeneic or syngeneic transplantation. Most transplants are from donors that are not genetically identical to the recipient (allografts). These types of transplants are known as allogeneic transplants. Finally, transplants from donors of one species to recipients of another species (xenografts) are known as xenogeneic transplants.
Due to the scarcity of isografts and the obvious limitations to autografts, allogeneic transplants are most commonly used today. The success of allogeneic transplantation relies on more or less sophisticated mechanisms for identifying and matching specific genetic markers between the donor and the recipient depending on the organ, as well as suppressing the recipient’s immune system to prevent transplant rejection. These are concepts that serve as the basic foundation in transplantation medicine. In the future, these concepts may be extended and improved to allow transplantation across species. Tissue xenografts, which have been treated to reduce their immunogenicity, have been used as a successful treatment modality in some applications (i.e., porcine heart valves), but whole-organ xenografts have been unsuccessful. Research regarding genetically altered xenografts is ongoing. When the important immunologic barriers to xenogeneic transplantation are eliminated, the use of animal donors may possibly alleviate the relative paucity of available organs. Of course, significant ethical questions, as well as transplant longevity and transmissible infectious diseases from animals, are issues that must be addressed before these types of transplants become commonplace. Bioengineered tissues that have been fabricated in vitro and transplanted back into the patient have also been successful, including a trachea and a bladder.
TRANSPLANTATION IMMUNOLOGY
Transplantation immunology encompasses most aspects of the human immune response to alloantigens expressed by the recipient as well as the donor organ/tissue. When donor and recipient genetic disparities exist, the recipient mounts a specific immune response to the alloantigens expressed by the donor grafted organ/tissue. In addition, donor T cells contained within the transplanted graft or organ can recognize foreign tissue in the host and mount a “graft-versus-host” reaction. Transplantation medicine would be grossly unsuccessful if this concept were not appropriately appreciated and manipulated.
T lymphocytes are the primary, though not the only, cells that mediate graft rejection as well as graft-versus-host disease (GVHD). T cells can become activated shortly after transplant by recognizing foreign major and minor human leukocyte antigen (HLA) molecules. The major histocompatibility complex (MHC), a genetic region found on the short arm of chromosome number 6 of all mammalian cells, codes for HLA molecules that allow immune cells to identify self from nonself. Although there are many other gene products, from more than 30 histocompatibility gene loci, which can stimulate graft rejection, it is the HLA system that produces the strongest immunologic response.
MHC genes are inherited from each parent; every child has components of both the mother’s and the father’s HLAs on their cell surfaces. The MHC/HLA system is broadly divided into regions. MHC class I and class II regions are those significantly involved in rejection and GVHD (see later in the chapter). MHC class I regions include HLA-A, -B, -C, -E, -F, and -G. (The present role of the -E, -F, and -G regions in transplantation is not well understood.) MHC class II regions include HLA-DR, -DQ, -DP, -DO, and -DN. MHC class II genes have two chains, allowing for four different gene products for each locus.
The MHC has extensive polymorphism, allowing remarkable diversity among genes of the HLA system. There are over 180 different class I alleles in the HLA-B region alone and over 220 class II alleles in just one loci of the HLA-DR region that have been recognized in humans. Deoxyribonucleic acid (DNA)-based typing (see below) has led to a more specific and detailed classification of the transplantation genes, such that the HLA alleles are related to their DNA sequences.
HLA class I antigens are expressed on most nucleated cells and on red blood cells, whereas class II antigens are expressed only on certain cells known as antigen-presenting cells (APCs). APCs include macrophages, B cells, dendritic cells, and some endothelial cells. The expression of these MHC gene products (antigens) on a cell’s surface is regulated by various cytokines such as interferon (IFN) and tumor necrosis factor (TNF).
In the setting of solid organ transplantation, the transplanted foreign MHC molecules activate the immune response by stimulating the recipient’s T cells to respond to foreign antigens. The interaction of the MHC of the donor cells with the recipient’s T-cell receptor initiates the immune reaction that can lead to rejection. T cells can be activated either by the donor’s or the recipient’s APCs, resulting in the expression and production of lymphokines and cytokines that promote activation of cytotoxic T cells, activation of B cells, and activation of natural killer cell activity, as well as promote enhanced expression of MHC and increased macrophage activity. This, in turn, causes further immune reactions that result in direct tissue damage and damage to the vascular endothelium of the graft, which may ultimately result in graft rejection.
Despite the treatment of recipients with immunosuppressive drugs, the potential for immunologic rejection is not entirely eliminated. Rejection may be characterized as acute, a process evolving rapidly over days to weeks. Acute rejection can occur at any time, from hours after transplant to years later. This acute process is related to the primary activation of T cells and usually can be reversed by modifying or intensifying the immunosuppressive regimen.
Chronic rejection of the allograft is also a significant problem leading to organ failure. This type of rejection is slow and insidious and in most cases cannot be reversed by conventional immunosuppressive drugs. It likely occurs by continued, albeit muted, cell-mediated toxicity that results in vascular endothelial damage of the transplanted organ (as well as other actions, which have not been fully elucidated), leading ultimately to graft failure.
Another type of rejection is known as hyperacute rejection; this occurs within minutes to hours after a transplantation procedure. This pattern of rejection occurs in patients who have undergone previous transplantations, patients who have had multiple pregnancies, and patients who have had multiple blood transfusions. It is caused by the presence of preformed antidonor antibodies in the recipient that activate complement cascade, resulting in severe damage to the parenchymal constituents of the graft, which often cannot be reversed.
Transplant immunology is even more complicated in the setting of allogeneic HSCT. Without proper immune suppression, residual host lymphocytes can mediate graft rejection, leaving a patient aplastic. However, unlike solid organ transplant, a hematopoietic stem cell graft has large numbers of mature lymphocytes capable of reacting against nonself antigens in the host in inducing GVHD. Donor leukocytes tend to react against the skin, liver, and gastrointestinal tract. Occasional involvement of the lungs can be seen as well. GVHD varies from nonexistent in approximately 50% of patients to severe and life-threatening. GVHD remains one of the major limitations to successful HSCT. Intensive therapies designed to minimize GVHD have resulted in higher rates of relapse from loss of the potential “graft-versus-tumor” (GVT) effect and from higher rates of infection. Ultimately, therapies that may limit GVHD and retain the important GVT activity of the donor graft will be needed to improve the outcomes of HSCT, and several strategies are in clinical testing.
It should also be noted that in unusual cases after solid organ transplant, mature donor lymphocytes transplanted with the organ can engraft and likewise cause GVHD. This is particularly complicated and similar to “transfusion-associated GVHD” (TA-GVHD), which can lead to marrow aplasia without the support of a donor stem cell graft. Similar to TA-GVHD, the prognosis is often poor, and treatment requires early recognition and intensive intervention with immune suppression.
CLINICAL INDICATIONS
The clinical indications for transplantation vary, but the disease outcome can be fatal without the transplant. The more common indications for transplantation are listed in Table 20-2.
Other indications can be added to this list when quality of life can be improved by transplantation. For example, autologous HSCT may ameliorate the effects of systemic lupus erythematosus or other autoimmune disorders. HSCT has been performed as a treatment for the management of various metabolic disorders and solid tumors, germ cell tumors, and neuroblastoma.2
In recent years there has been a steady increase in vascularized composite allograft transplantations. These include facial, craniofacial, hand, limb, and genitourinary transplantations. Such transplantations prompt new ethical questions.3
MEDICAL MANAGEMENT
Medical management of the transplant candidate focuses on successfully preventing rejection. In solid organ transplantation, when the donor and recipient tissue are genetically identical (autologous or syngeneic), the outcome of the transplantation relies upon the surgical success of the procedure. For autologous HSCT, success is largely dependent on the high-dose chemotherapy or radiation used to eradicate residual malignant cells prior to HSCT. When tissue from genetically different sources is transplanted, a sophisticated means of preventing rejection must be instituted to ensure graft survival, and in the setting of allogeneic stem cell transplant, methods must be used to prevent both graft rejection and severe GVHD. Transplantation surgeons and oncologists have improved the surgical procedures for various transplantations. Medical management to achieve longer-term successful grafts and longer-term overall patient survival has been quite successful, yet it still is fraught with longer-term complications. The success of an allogeneic transplantation relies on the ability to identify and match certain genetic markers between the donor and the recipient while suppressing the recipient’s immune system in order to prevent rejection.
Table 20‐2 Major indications for transplantation*.
Type of Transplant | Indications |
---|---|
Kidney** | End-stage renal disease |
Diabetic nephropathy | |
Glomerulonephritis | |
Pyelonephritis | |
Congenital abnormalities | |
Nephrotic syndrome | |
Liver | Fatty liver disease (both alcohol and nonalcoholic fatty liver) |
Viral cirrhosis | |
Autoimmune chronic liver disease | |
Biliary atresia (children) | |
Pancreas | Severe diabetes |
Severe hypoglycemic unawareness | |
Hypertensive nephrosclerosis | |
Isolated pancreatic islets | Severe hypoglycemic unawareness |
Intestinal | Short bowel syndrome |
Functional bowel problems | |
Heart | Hypertrophic cardiomyopathy |
Dilated myopathy | |
Valvular heart disease | |
Severe coronary artery disease | |
Congestive heart failure | |
Congenital heart defect | |
Heart and lung | Multiorgan end-stage disease |
Congenital abnormalities | |
Amyloidosis | |
Lung | Primary pulmonary hypertension |
Chronic obstructive pulmonary disorder/emphysema | |
Pulmonary fibrosis | |
Cystic fibrosis | |
Hematopoietic cell transplantation (autologous)** | Acute myelogenous leukemia |
Multiple myeloma and amyloidosis | |
Lymphoma (Hodgkin and non-Hodgkin) | |
Some solid tumors (germ cell, ovarian) | |
Systemic lupus erythematosus/autoimmune disorders | |
Ewing sarcoma | |
Neuroblastoma | |
Wilms tumor | |
Osteosarcoma | |
Medulloblastoma | |
Juvenile rheumatoid arthritis | |
Hematopoietic cell transplantation (allogeneic)** | Acute myelogenous leukemia |
Acute lymphoblastic leukemia | |
Chronic myelogenous leukemia | |
Multiple myeloma | |
Aplastic anemia | |
Primary immune deficiencies | |
Hemoglobinopathies (sickle cell anemia, thalassemia) | |
Myelodysplastic syndrome | |
Multiple sclerosis | |
Fanconi anemia | |
Dyskeratosis congenital | |
Blackfan–Diamond anemia | |
Wiskott–Aldrich syndrome | |
Lymphoproliferative disorder | |
Chronic granulomatous disease | |
Mucopolysaccharidoses (MPS-I and MPS-VI) | |
Some metabolic diseases | |
Osteopetrosis | |
Globoid cell leukodystrophy (Krabbe) | |
Metachromatic leukodystrophy | |
Cerebral X-linked adrenoleukodystrophy | |
Face and/or craniofacial | Composite midface defects/mutilation |
Limb | Upper-extremity disabilities Lower-extremity disabilities |
Genitourinary transplantations | Absolute uterine-factor infertility (uterus) Genital loss (penile) |
* Partial listing only. Additional specification on the Organ Procurement and Transplant Network webpage.1
** Per the specifications of the Guidelines from the American Society for Blood and Marrow Transplantation.2
BLOOD AND TISSUE TYPING
Standard ABO and Rh blood typing is performed to prevent hyperacute rejection of the transplant based on isoagglutinin incompatibility. ABO matching is mandatory for solid organ transplant and directly impacts short-term graft survival. ABO matching does not appear to impact graft survival after allogeneic HSCT; the impact on patient outcomes such as relapse is controversial, but is likely to be small and of unclear significance that may depend on graft source and disease.
Cross-matching (crossing recipient serum with donor lymphocytes) is usually done to prevent hyperacute rejection in allogeneic solid organ transplants. This is a basic serologic test that is regarded as necessary, particularly in those allogeneic transplant recipients who have previously experienced massive immune challenges such as a prior transplantation, multiple pregnancies, or multiple blood transfusions, and have developed preformed anti-HLA antibodies. The existence of preformed alloantibodies is detected by single HLA antigen bead technology. Since transplantation of solid organs (heart, lung, liver) often requires some expediency, time-consuming complex cross-matching or tissue typing cannot be performed. Instead, absence of antibodies to a panel of cells (defined in advance and known as panel-reactive antibodies) is usually adequate for heart and lung transplantation. Interestingly, MHC compatibility in liver transplantation seems negligible in achieving better outcomes. This is fortunate, because the timing of liver transplantation often precludes HLA typing.
The most critical matching criterion for allogeneic HSCT is tissue typing to determine and match HLA. HLA molecules are found on donor and recipient cells, and in the setting of HSCT are critical for determining engraftment and complications such as GVHD. HLA typing is much less critical in renal transplantations and for graft survival in liver or heart transplantations. Tissue typing can be performed by serologic assays, but more commonly, most typing is performed using rapid DNA-based testing methods. Polymorphisms in HLA antigens are common, and it is now practical and routine to test not just for HLA antigens but for HLA alleles. In the setting of allogeneic HSCT, it is clear that allele level matching improves outcomes. Matching at class I HLA-A, -B, -C, and class II (HLA-DR) is critical in all cases. The role of matching at the HLA class II molecule DQ is less clear and a single DQ mismatch does not seem to impact the outcome after HSCT.
IMMUNOSUPPRESSION
Immunosuppressive regimens vary among transplant centers and according to organ type (intestines, liver, etc.). Since tissues in allogeneic transplants are not genetically identical, medications used to control the immune response are essential for graft survival. All allogeneic transplantations initially require immunosuppression to prevent acute and hyperacute and/or acute cellular rejection. Furthermore, most allogeneic solid organ transplant recipients require lifelong maintenance immunosuppression and may require even more intensive immunosuppression should they develop rejection. Long-term immunosuppression is usually not required in allogeneic HSCT, and autologous HSCT generally requires no immunosuppression at all.
Most immunosuppressive medications are nonspecific and cannot prevent a specific component of the immune response. More sophisticated and directed agents are currently in development that will allow for graft tolerance while allowing the immune reaction to infection or other detrimental antigens to remain intact.
One of the most significant advances in transplantation has been made in pharmacotherapeutic immunosuppression. As mechanisms of graft rejection are better understood, agents become more specific in their mode of immune modulation.
Immunosuppression in solid organ transplant can be divided into induction (used at the time of implantation of the graft or soon after) and maintenance (to prevent rejection long term) (Figure 20-1). The most frequently used contemporary medication classes are discussed in this chapter (see Table 20-3), with a brief review of some key formulations.
Calcineurin Inhibitors
Calcineurin activates a nuclear component of T cells that is thought to initiate gene transcription for the formation of interleukin (IL)-2. The development of calcineurin inhibitors (known as CNI), specifically cyclosporine A (CSA) in 1981, was a watershed moment in solid organ transplantation and immediately lead to drastically lower rates of rejection.
Cyclosporine
CSA is a cyclic polypeptide macrolide medication derived from a metabolite of the fungus Tolypocladium inflatum. It specifically and reversibly inhibits immunocompetent lymphocytes in the G0 and G1 phase of the cell cycle by binding with the intracellular protein cyclophilin, and inhibiting calcineurin, which is presumed to inhibit IL-2 by preventing gene expression. CSA also reduces the expression of IL-2 receptors. This medication has some effect on humoral immunity but not on phagocytic function, neutrophil migration, macrophage migration, or direct bone marrow suppression. Absorption of this drug is variable, and blood levels must be drawn 2 hours after dosing to ensure that the drug is in the therapeutic range.

Figure 20‐1 Phases of immunosuppression in solid organ transplantation. During the induction phase, the goal is to prevent early rejection. During the maintenance phase, the goals are to prevent acute/chronic rejection, to maximize immunosuppression, and to minimize toxicity. If rejection develops, the treatment goals are to increase immunosuppression, filter/remove/block offending immune components, and enable residual organ function. In some patients, retransplantation is needed in order to overcome the rejection.
Table 20‐3 Major immunosuppressive agents*.
Drug | Type | Indications | Major Side Effects† | Implications in the Dental Office† |
---|---|---|---|---|
Cyclosporine | Calcineurin inhibitor | Maintenance IS | Nephrotoxicity Neurotoxicity Elevation of blood pressure Cosmetic side effects PTLD |
Immunosuppression related‡ Drug–drug interaction (P-450 CYP3A)§ Vital signs monitoring Gingival overgrowth Risk of neoplasm |
Tacrolimus | Calcineurin inhibitor | Maintenance IS | Nephrotoxicity Neurotoxicity Post-transplantation diabetes mellitus Elevation of blood pressure PTDM |
Immunosuppression related‡ Drug–drug interaction (P-450 CYP3A)§ Vital signs monitoring Risk of neoplasm |
Sirolimus | mTOR inhibitor Antiproliferative |
Maintenance IS Potential treatment for chronic rejection Used in CNI-sparing IS |
Delayed wound healing Hyperlipidemia Hypertriglyceremia Proteinuria Bone marrow suppression Interstitial pneumonitis |
Immunosuppression related§ Drug–drug interaction (P-450 CYP3A)§ Vital signs monitoring Risk of neoplasm |
Everolimus | mTOR Inhibitor Antiproliferative |
Maintenance IS Potential treatment for chronic rejection Used in CNI-sparing IS |
Delayed wound healing Hyperlipidemia Hypertriglyceremia Proteinuria Bone marrow suppression |
Risk for osteonecrosis of the jaws Risk of neoplasm |
Azathioprine | Antimetabolite | Adjuvant to maintenance IS | Bone marrow suppression Hepatotoxicity |
Immunosuppression related‡ Risk of neoplasm |
Mycophenolate mofetil | Antimetabolite | Adjuvant to maintenance IS | Immunosuppressant‡ Leukopenia |
Immunosuppression related‡ Absorption is altered by antibiotics, antacids, and bile acid binders Risk of neoplasm |
ATG/ALG | Polyclonal antibody | Induction IS Treatment of very severe rejection |
Leukopenia PTLD Pulmonary edema Renal dysfunction |
Immunosuppression related‡ Risk of neoplasm |
Basiliximab | Monoclonal antibody | Induction IS | Pulmonary edema Renal dysfunction |
Immunosuppression related‡ Risk of neoplasm |
Corticosteroids | Nonspecific immunosuppressant | Induction IS Maintenance IS Treatment of acute rejection |
(see Box 20-1) | Immunosuppression related‡ Peptic ulcer related (avoid NSAIDs) Vital signs monitoring Poor wound healing Risk of neoplasm Steroid supplement may be needed with stressful procedures As applicable for diabetes, if develops Possible history of bisphosphonates |
ASA, acetylsalicylic acid; ATG/ALG, antithymocyte globulin/antilymphocyte globulin; CNI, calcineurin inhibitor; CV, cardiovascular; IS, immunosuppression; mTOR, mammalian target of rapamycin; NSAIDs, nonsteroidal anti-inflammatory drugs; PTDM, post-transplantation diabetes mellitus; PTLD, post-transplantation lymphoproliferative disorder.
* Major mechanisms of action are outlined in the text.
† Partial listing only.
‡ Use of an immunosuppressant results in an increased risk of infection.
§ Dental/oral pharmacotherapeutics that are metabolized by the liver’s cytochrome P-450 CYP3A system alter this drug’s serum levels. This group of medications includes, but is not limited to, erythromycin, clarithromycin, azole antifungals, benzodiazepines, carbamazepine, colchicine, prednisolone, and metronidazole.
Tacrolimus
Tacrolimus (FK-506; Prograf) is a macrolide immunosuppressant produced by Streptomyces. Similar to CSA, it suppresses cell-mediated reactions by suppressing T-cell activation. Tacrolimus inhibits calcineurin by interacting with an intracellular protein known as the FK-binding protein. Consequently, T cells are not activated, and cell-mediated cytotoxicity is impeded. There may be a lower incidence of rejection with the use of tacrolimus compared with the use of CSA in liver, kidney, and lung transplantations. Overall graft and patient survival rates in kidney transplantations do not seem to differ significantly with the use of this medication, although the safety profiles do differ and seem to favor tacrolimus.4
Inhibitors of Mammalian Target of Rapamycin
Sirolimus (rapamycin; Rapamune) and everolimus (Afinitor) are other macrolide immunosuppressive agents. Sirolimus is produced by Streptomyces hygroscopicus. These mammalian target of rapamycin (mTOR) inhibitors are used for prophylaxis against acute rejection of solid organ transplants and may be appropriate for use in chronic rejection. They inhibit the activation of a particular cellular kinase (target of rapamycin [TOR]), which then interferes with the intracellular signaling pathways of the IL-2 receptor, thereby preventing lymphocyte activation. Everolimus is more selective for mTORC1 and may have less impact on glucose homeostasis. Both sirolimus and everolimus lead to inhibition of T-cell response to IL-2 and other cytokines. The overall effect is interference of T-cell activation during the cells’ G1 to S phase. Sirolimus and everolimus are used in conjunction with CNIs. Their antiproliferative effects lead to potential inhibitory effects on neoplasia and fibrosis as well, but can impair wound healing.4 They can also be associated with significant toxicity. Sirolimus has been shown to reduce acute rejection when azathioprine (AZA) is used.
Inhibitors of Purine and Pyrimidine Synthesis
Azathioprine
AZA is an antimetabolite that inhibits ribonucleic acid and DNA synthesis by interfering with the purine synthesis that results in decreased T- and B-cell proliferation. It does not interfere with lymphokine production, but has significant anti-inflammatory properties. AZA can be bone marrow suppressive, leading to pancytopenia, and it can also cause hepatotoxicity, usually mild but potentially severe. Therapeutic response is delayed, normally taking 3 months but occasionally requiring up to 6 months in some patients.5 In addition, polymorphisms in the TPMT gene that codes for an enzyme involved in AZA metabolism are associated with severe bone marrow toxicity, including aplastic anemia. For these reasons, AZA has lost favor with the advent of mycophenolate mofetil (MMF).
Mycophenolate Mofetil
MMF, an ester of mycophenolic acid, is an antimetabolite used for prophylaxis against graft rejection, and may have some action in reversing ongoing acute rejection. It inhibits inflammation by interfering with purine synthesis. Both T cells and B cells, which are dependent on this synthesis for their proliferation, are prevented from reproducing. Additionally, MMF interferes with intercellular adhesion of lymphocytes to endothelial cells. It does not inhibit IL-1 or IL-2. MMF is used as an adjunct to CNI-based immunosuppression and is commonly used in all solid organ transplants.
Corticosteroids
Corticosteroids are consistently used in all allogeneic transplantations for prophylaxis against graft rejection and for reversal of acute rejection. The mechanism of action of this medication is nonspecific, as it affects the immune system in many complex ways. Steroids have anti-inflammatory effects and are able to suppress activated macrophages. They also interfere with antigen presentation and reduce the expression of MHC antigens on cells. Steroids reverse the effect of IFN-γ and alter the expression of adhesion molecules on vascular endothelium. These medications also have significant effects on IL-1 activity and block the IL-2 gene and its production.
Antibody-Based Immunosuppression
Antithymocyte and Antilymphocyte Globulin
Polyclonal antilymphocyte sera, antilymphocyte globulin (ALG), and antithymocyte globulin (ATG) are part of the same medication class. These agents are produced by immunizing animals with human lymphoid cells; the animals produce antibodies, which then reduce the number of circulating T cells after infusion into transplanted recipients. Individually, these agents affect lymphocyte immunosuppression by reacting with common T-cell surface markers and then coating (opsonizing) the lymphocyte—marking it as foreign for phagocytosis. Polyclonal antibodies are used as conditioning agents prior to transplantation as well as for reversal of steroid-resistant rejection.
Basiliximab
Basiliximab (Simulect) is made of synthetic monoclonal antibodies used in some centers for induction immunosuppression. These monoclonal antibodies bind the CD25 receptor (IL-2 receptor) on the surface of activated T cells (IL-2 receptor antagonists), preventing the expansion of CD4 and CD8 lymphocytes. They may be effective in conjunction with MMF and corticosteroids to eliminate the need for CNI use in the early post-transplantation period. Anti-CD25 agents have also been reported to be efficacious in treatment of corticosteroid-resistant GVHD.
Alemtuzumab
Alemtuzumab (previously known as Campath-1; currently, Lemtrada) is a monoclonal antibody that binds to and depletes CD52, which is expressed on the surface of mature lymphocytes. It is used for the treatment of chronic lymphocytic leukemia, and in some conditioning regimens for bone marrow, kidney, and islet transplantation.
Conditioning in Hematopoietic Stem Cell Transplantation
Unlike solid organ transplant, HSCT requires “conditioning” of the patient with cytotoxic chemotherapy. Traditional conditioning regimens were designed to take advantage of the dose–response activity against cancer cells. High doses of chemotherapy or radiation are used to eradicate residual tumor prior to infusion of the hematopoietic stem cell product. Typical myeloablative agents include cyclophosphamide in combination with busulfan, etoposide, fludarabine, or often total body irradiation (TBI). A complication of the conditioning regimen is myeloablation, requiring the need to transplant additional donor hematopoietic stem cells. The conditioning therapy must also be sufficiently immunosuppressive to prevent graft rejection, or patients would be left aplastic and die from complications of cytopenias and infections. It has been recognized for years, however, that the success of transplantation is also related to the immunologic activity of the donor graft, independent of the conditioning therapy. Donor T cells have the ability to react against and kill residual tumor cells and induce a GVT effect. This observation led to the development of reduced-intensity conditioning (RIC) regimens designed primarily for immunosuppression to allow donor cell engraftment, and to take advantage of the GVT activity without the organ toxicity often associated with very high doses of chemotherapy and radiation. Typical regimens for RIC HSCT include similar drugs and TBI as used in myeloablative transplant, but, as the name implies, often at reduced doses. While treatment-related morbidity and mortality are less with these regimens, this is often offset by higher relapse rates. RIC HSCT results in significantly less organ toxicity, including mucositis.
Other Immune-Modulating Agents
Rituximab (Rituxan and Biogen) is a chimeric monoclonal antibody that binds to CD20 antigen of B cells. It depletes the level of mature B cells by various mechanisms such as mediation of antibody-dependent cellular cytotoxicity, complement-dependent cytotoxicity, and B-cell apoptosis.6
Bortezomib (Velcade) is a proteasome inhibitor. Proteasome inhibition can downregulate NF-κB activity; decrease cell proliferation/differentiation; induce apoptosis via cell cycle arrest, endoplasmic reticulum stress, and caspase induction due to the accumulation of unfolded or misfolded proteins; and downregulate antigen presentation, cell–cell interaction, and cell migration.7 Bortezomib is approved in the United States for multiple myeloma and mantle cell lymphoma; however, based on its mechanism of action, it is used extensively for the management of rejection in transplantation.
Eculizumab (Soliris) is a humanized monoclonal antibody directed against the complement C5a component. It is currently being used in renal transplantation and HSCT.
Newer Immunosuppressive Strategies
A scientific breakthrough concept is the chimeric antigen receptor (CAR) T-cell therapy. The CAR is directed at a disease-specific antigen. During this process, the patient’s leukocytes are collected via leukapheresis. These T cells are processed ex vivo using retrovirus or lentivirus to transduce expression of vector containing a gene coding the CAR construct. The CAR construct is a protein that has an extracellular domain that can identify the abnormal cell, and at least two intracellular domains. The intracellular domains have two roles: direct activation of the T cell and co-stimulation of the T-cell activation (CD28 or 4-1BB domains). Then the engineered CAR T cells are grown in culture to produce an appropriate quantity. Finally, the CAR T cells are infused into the patient, typically following a lymphodepleting conditioning regimen, such as fludarabine and cyclophosphamide. These CAR T cells identify the disease-specific abnormal cells and destroy them.
Two types of engineered T cells are approved by the US Food and Drug Administration: axicabtagene ciloleucel and tisagenlecleucel. The early indications were refractory lymphoid malignancies, especially diffuse large B-cell lymphoma (DLBCL) and acute lymphoblastic leukemia (ALL), which often express CD-19. Therefore, the CAR T cells are targeted at the CD-19. This technology is being expanded in other diseases. Promising results were reported in the treatment of multiple myeloma, with CAR T-cell therapy targeting the B-cell maturation antigen.8 This CAR T-cell therapy is also being explored with certain efficacy in clinical trials in solid malignancies such as glioblastoma, neuroblastoma, non-small-cell lung cancer, osteosarcomas, and Ewing sarcoma.9,10 Therefore, the number of patients undergoing transplants of this type is expected to increase.
Although CAR T cells achieved complete remission in a large portion of the patients with relapse-free survival of up to 12 months, it is associated with severe toxicities, including cytokine release syndrome and neurotoxicity. Current research is aimed at improving the response rate and duration of remission and decreasing toxicity.11
Similar immunosuppressive therapies are used in patients undergoing HSCT. Immunosuppression in this setting is used not only to prevent graft rejection, but also to prevent GVHD. Approaches used for GVHD prevention and management include pharmacologic agents that inhibit T-cell function, antibody therapy directed against T cells, selection of stem cell grafts in ways that eliminate T cells prior to transplant, various approaches to inhibit T-cell trafficking to sites where GVHD can be initiated, enhancement of suppressive mechanisms that can limit T-cell activity (such as infusion of regulatory T cells and mesenchymal stem cells), and the use of other novel compounds that may modulate T-cell function and activity. More recently, tyrosine kinase inhibitors (TKIs) were attempted for steroid-resistant chronic GVHD (cGVHD). The first agent was imatinib (Gleevec) for sclerodermatous cGVHD, and more recently ibrutinib (Imbruvica) for second-line treatment for cGVHD.12 Ibrutinib inhibits the Bruton tyrosine kinase, as well as the interleukin-2–inducible kinase (ITK), which initiates a cascade that ends with T-cell activation, cytokine release, and rapid proliferation.13 The T-cell activation by ibrutinib inclines toward Th2, which is linked to cGVHD. The most promising therapies for cGVHD in early-phase trials include Janus kinase (JAK) inhibitors (ruxolitinib and bacritinib), immune checkpoint inhibitors (abatacept), monoclonal antibodies against integrin (natalizumab), proteasome inhibitors (ixazomib and carfilzomib), cytokines (IL-2 and IL- 22), CCR5 receptor antagonists (maraviroc), and cytokine modulators (alpha-1 antitrypsin).14 This is a rapidly changing landscape and has been reviewed in detail.
ANTIMICROBIAL MEDICATION
In addition to immunosuppressive medication regimens, antimicrobial medication regimens are important in preventing infection in the transplant recipient. These regimens vary from center to center and from program to program. Transplant patients are typically profoundly immunosuppressed for months. Many factors affect the pace of immune reconstitution, but it is common to use prophylactic antibiotics for bacterial, protozoan, fungal, and viral infections. Antimicrobial medication coverage has proven to be effective in the prevention of common transplant-associated infections (Table 20-4).
The Centers for Disease Control and Prevention (CDC) have published updated guidelines for preventing opportunistic infections among HSCT recipients based upon the quality of the evidence supporting the recommendation. Prophylaxis is, however, very different from the empiric treatment required in febrile neutropenia.
Table 20‐4 Most common pathogens requiring prophylaxis post liver transplantation.
Pathogen | Prophylaxis | Duration of Prophylaxis* |
---|---|---|
Cytomegalovirus (CMV) | Ganciclovir Valganciclovir |
6 months |
Pneumocystis jiroveci | TMP–SMX† Atovaquone Dapsone Aerosolized pentamidine Dapsone plus pyrimethamine |
3–12 months |
Candida Albicans | Fluconazole Itraconazole Echinocandins |
3–6 months |
Herpes simplex virus | Ganciclovir Valacyclovir Acyclovir§ |
3–6 months |
* Varies according to transplant center.
† Trimethoprim–sulfamethoxazole.
§ Choice in patients that do not require concomitant CMV prophylaxis.
COMPLICATIONS
Complications with transplantation are still common and require close evaluation and management. General complications can be broadly characterized into those caused by rejection, side effects from medication, and those induced by immunosuppression. Additionally, there are some organ-specific complications observed in certain types of transplantation.
Rejection
As previously mentioned, rejection of the transplanted organ remains a significant obstacle to long-term transplant graft and patient survival. Rejection is characterized by time course and mechanism of injury. Rejection leads to end-organ damage and can lead to allograft failure, often associated with reappearance of complications of organ failure. Clinically, rejection may manifest in many ways, including abnormal liver function such as bilirubin, albumin, and International Normalized Ratio (INR; liver failure); a decreased metabolism of medications (rejection of liver/kidney); or even complete organ failure and death (rejection of liver/lung/heart). In cases of end-organ disease (except those of kidney failure), retransplantation may be the only way to prevent death.
Rejection is continually screened for throughout the post-transplantation period. Most chronic rejections are insidious and are detected by laboratory analysis and by organ biopsy. Graft biopsy provides a reliable means to assess rejection. An alternative approach to monitoring rejection in a transplanted heart is the use of pacemakers to record changes in ventricular evoked response (VER). Subtle changes in VER have been correlated with rejection of heart transplants.
Complications of Immunosuppression Medication
Medications used to produce immunosuppression and prevent graft rejection have significant systemic side effects, which pose serious complications to the transplant recipient. Some of the major side effects are listed here (Table 20-3); however, complete drug information can be obtained through an appropriate medication reference source.
CSA, a mainstay in immunosuppression, is nephrotoxic and may alter renal function. It is also associated with hypertension and lowering of seizure threshold, as well as rare cases of hepatotoxicity. It also has cosmetic side effects such as gingival hyperplasia and hirsutism that can be quite concerning to patients. CSA is metabolized via the P-450 CYP3A system of the liver; therefore, it has many drug interactions, including interactions with drugs frequently used in dentistry, such as lidocaine and oxycodone.
Tacrolimus has been associated with hypertension and nephrotoxicity. In addition, it is neurotoxic and rare hepatotoxicity has been described.4 There are many other side effects associated with the use of this medication, one of which is the development of insulin-dependent post-transplantation diabetes mellitus (PTDM). The incidence of PTDM appears to be higher with tacrolimus use than with CSA use in liver transplantation. Tacrolimus is metabolized by the P-450 CYP3A system in the liver. It is 99% protein bound and requires titration. Tacrolimus also has significant interactions with medications used in dentistry, such as ibuprofen and metronidazole.
Sirolimus causes or exacerbates proteinuria and may cause abnormal liver enzymes. It can cause stomatitis and oral ulceration and lead to interstitial pneumonitis in rare cases.4 Sirolimus is also associated with a high incidence of hyperlipidemia owing to elevated triglyceride and cholesterol levels. Being a substrate for P-450 CYP3A, sirolimus interferes too with the metabolism of other medications.
AZA may cause bone marrow suppression, resulting in pancytopenia, which leaves the patient not only susceptible to opportunistic infections, but also at significant risk for bleeding. It also causes skin rash and pancreatitis in some patients.4
MMF has significant drug interactions that are particularly important to the dentist. One interaction commonly cited occurs as a result of antibiotic regimens that can alter gastrointestinal flora, leading to dramatic changes in MMF drug levels. For example, if a patient is taking a broad-spectrum antibiotic for a dentoalveolar infection, the possibility and probability of an abnormal MMF level do exist. Other medications, such as antacids (containing magnesium or aluminum) and bile acid binders, may also interfere with the absorption of MMF. MMF is usually well tolerated, without significant hepatotoxicity (although higher doses are associated with gastrointestinal symptoms of nausea, vomiting, and diarrhea) or nephrotoxicity, but hematologic alterations (mostly leukopenia) can be a side effect. It is also teratogenic.
Monoclonal and polyclonal antibodies can be associated with a severe reaction known as cytokine release syndrome. Cytokines (including TNF-α) are rapidly released, resulting in significant medical issues, including fever, chills, nephrotoxicity, vomiting, pulmonary edema, and, in a few instances, arterial thrombosis.
Both monoclonal and polyclonal antibodies have been associated with significant side effects (in addition to significant cytokine release), including a high risk of viral/fungal infection and an increased incidence of post-transplantation lymphoproliferative disorder (PTLD).
Corticosteroids, another mainstay used in transplantation immunosuppression, can have multiple detrimental side effects (Box 20-1).
Cytotoxic agents such as cyclophosphamide, busulfan, and TBI cause bone marrow suppression, resulting in pancytopenia.
Complications of Immune Modulation
Immunosuppression used to prevent rejection of a transplanted organ also can pose serious complications to the recipient, including life-threatening infections and cancer.
Infections after HSCT are a significant problem. The type of transplant and the time that has transpired since the transplantation often predict the specific infection. For example, patients who have had an HSCT usually have broad immunologic defects, either due to their underlying disease or more likely from a combination of immunosuppressive drugs and delayed immune reconstitution. This impacts all components of the immune system. These patients are at a significantly higher risk of infection than are those patients transplanted with solid organs. Additionally, transplants of certain organs are associated with a greater likelihood of a particular infection.
Timing following the transplantation may correspond with a specific infective process. Bacterial infections are usually seen in the early postoperative period (immediately after transplantation) in solid organ transplantations. The type of bacteria varies with each specific organ and may include both gram-positive and gram-negative bacterial species. Drug-resistant bacterial infections have been documented, such as staphylococcal infections associated with skin wounds, upper and lower respiratory infections (pneumonia), and tuberculosis. Infective endocarditis has also been seen in transplant recipients. In this population, endocarditis is often related to Staphylococcus infection or aspergillosis.
Systemic viral infections are a common problem in immunosuppressed patients. CMV and herpes simplex viruses (HSVs) are often the etiologic viral agents involved. Other viral agents, including adenovirus, respiratory syncytial virus (RSV), varicella-zoster virus (VZV), Epstein–Barr virus (EBV), and human parvovirus B19, are also common causes of disease in a transplant population. Viral infections may be related to time following transplantation. HSV infections usually occur at 2–6 weeks after organ transplantation, whereas CMV infections generally occur at 1–6 months after transplantation, and VZV infections typically occur between 2 and 10 months post transplantation.
Patients who are immunosuppressed are susceptible to local and systemic fungal infections that vary from those of Candida species to deep fungal infections caused by Aspergillus, Cryptococcus neoformans, Fusarium, and Trichosporon. Invasive fungal infections are usually seen later in the transplantation process. Systemic fungal infections are often difficult to treat in the immunosuppressed patient and require systemic antifungal agents. Some have considered the role for macrophage colony-stimulating factor, a cytokine used to stimulate macrophages and monocytes, in the treatment of patients with fungal infections.
Parasitic infections caused by Toxoplasma gondii and others can be seen in immunosuppressed transplant recipients, but are uncommon in the context of solid organ transplantation.
In addition to, and perhaps directly related to, infectious complications, immunosuppression renders the patient at higher risk for the development of secondary cancers. The immune system provides surveillance against antigens that may act as initiators or promoters of cancer. When the immune response is muted, so, too, is this surveillance system. Cancers most commonly associated with immunosuppression are squamous cell carcinomas of the skin, lymphomas (mostly B-cell lymphomas including PTLD), and Kaposi’s sarcoma. Human herpesvirus 8 has been implicated in Kaposi’s sarcoma and EBV in PTLDs. The most important factors in the development of PTLD are the level of immunosuppression and the EBV serology status. Additionally, there seems to be a clinico-pathologic difference between those transplant recipients who are diagnosed with PTLD early (within the first year of transplantation, which are EBV + PTLD) versus those diagnosed after the first year.
Specific Complications of Transplantation
Solid Organ Transplantation
A significant medical complication seen in patients receiving solid organ transplants is accelerated advanced cardiovascular disease (CVD), including coronary artery disease (CAD). The cause of this rapid CVD is unknown, but some researchers postulate it could be either infectious (CMV), medication induced, or potentially both. In this population, CVD is likely multifactorial. For instance, many investigators have explored the etiologic role of immunosuppression in the development of CVD. Hypertension is a common side effect of CNIs. Steroids and CNIs are diabetogenic, CSA and sirolimus cause hyperlipidemia. All these medical complications are part of the metabolic syndrome and recognized as risk factors for CVD.
In many transplantation facilities, hypertension is treated by calcium-channel blockers (CCBs). Some clinicians note that this group of medications may raise serum levels of CSA, thus decreasing the cost of immunosuppression. Caution must be exercised with any drug affecting CSA metabolism; for this reason, most clinicians prefer to prescribe medications that do not alter CSA levels. Non-dihydropyridine CCBs are often first line, but can have adverse oral effects, such as gingival overgrowth (see below).
PTDM is another significant condition frequently seen post transplant. Experimental and clinical observations both suggest that this phenomenon is related in some part to immunosuppressive agents. PTDM may cause both macro- and microvascular changes, which affect both graft and patient survival.
Neuropsychiatric complications, such as anxiety, seizures, and neuropathies, can also be noted in transplant recipients.
More specifically in lung transplant, the second most common long-term cause of morbidity and mortality (infection being the first) after transplantation is bronchiolitis obliterans. This disorder is caused by inflammation and constriction in bronchioles. It may be related to chronic rejection and infection and perhaps altered microvasculature.
Heart transplantation can also be associated with particular complications. As previously mentioned, post-transplantation CAD is common in all transplants, including heart transplants. Additionally, early after transplantation the heart is denervated, such that symptoms of angina may be absent and the heart may have diminished vagal response. There is, however, evidence of sympathetic and possibly parasympathetic nerve regeneration later in the post-transplantation period, suggesting that angina and heart-rate changes from stress are regained. Care of patients with cardiac transplants must recognize these cardiac abnormalities. Mitral and tricuspid regurgitation has also been observed after heart transplantation (see Chapter 14 on Cardiovascular Disease).
Hematopoietic Stem Cell Transplantation
Perhaps the most significant complications are those observed after an allogeneic HSCT. Allogeneic transplantation often involves both administration of intensive chemotherapy and/or radiation, as well as the administration of immunosuppressive therapy. The major complications of allogeneic HSCT include (1) end-organ damage from pretransplantation conditioning therapy; (2) GVHD; (3) infections.
Major complications occur after HSCT that are directly related to the conditioning regimen. Unlike solid organ grafting, HSCT requires intensive chemotherapy and/or radiation to “condition” the recipient to accept the graft. The conditioning regimen must provide deep immunosuppression to prevent rejection and typically has cytotoxic effects to kill any residual malignant cells. Direct organ toxicity can occur with all types of HSCT, but appears higher after myeloablative conditioning than after nonmyeloablative or reduced-intensity conditioning. One major complication is sinusoidal obstructive syndrome (SOS), previously known as veno-occlusive disease of the liver. SOS is felt to be initiated by endothelial injury that leads to nonthrombotic sinusoidal occlusion, with an increase in sinusoidal pressures. This leads to cholestasis and ultimately can result in portal hypertension. It is associated with renal abnormalities and increased capillary permeability. SOS can ultimately lead to multisystem organ failure, encephalopathy, and even death. Clinical manifestations of SOS include jaundice, hepatomegaly, and fluid retention. Treatment for this process is supportive, and severe SOS is associated with high mortality rates. Another major complication is pulmonary toxicity, manifested as interstitial pneumonitis or alveolar hemorrhage. Pulmonary complication of both solid organ transplantation and HSCT has been reviewed in detail. The use of RIC for allogeneic transplantation appears to minimize direct organ toxicity and nonrelapsed mortality.
Perhaps the most frequently cited complication uniquely associated with HSCT is GVHD, which is a complex immunologic phenomenon that occurs when immunocompetent cells from the donor are given to an immunodeficient host. The host possesses antigens foreign to the transplanted graft that stimulate an immune response by the newly engrafted immune cells. GVHD affects the entire gastrointestinal system, including the mouth, as well as the skin and the liver. Other organs that may be involved in GVHD are the eyes, vagina, joints, lungs, and musculoskeletal tissues. This reaction can be lethal and requires therapy with intensive immunosuppression. Mucosal ulceration seen in GVHD may serve as an entry port for other infectious pathogens. This graft-versus-host reaction can attack the host’s hematologic cancer cells as well, leading to an antitumor effect that may be protective against relapse.
LONG-TERM PROGNOSIS POST TRANSPLANT
Transplantation outcomes have improved over the past several decades. Outcomes of solid organ transplants are summarized in Table 20-5. Clinical outcomes of solid organ transplantation are reported as graft survival and patient survival.
The number of patients on waiting lists for solid organ transplants continues to increase. The deaths of patients awaiting kidney transplants increased in 2019 to 3,759 while the death rates for patients awaiting heart and liver transplants in 2019 was 224 and 1,200, respectively. Since 2015, there has been a consistent reduction in number of deaths of heart transplant candidates (from 406 in 2015 to 224 in 2019). This may be due to adoption of new medical innovations such as ventricular assistance device.
Current estimates of HSCTs performed annually are greater than 50,000 worldwide. The annual rate of growth of this procedure has been estimated to between 40% and 50%. Improved HSCT-related healthcare has resulted in less morbidity and lower mortality rates. Historically, HSCTs for hematologic malignancies were undertaken as salvage therapy for refractory cancers, but outcomes are actually better for patients who are treated with HSCT soon after diagnosis or in remission, rather than after multiple relapses of hematologic disease. Outcomes have improved in both autologous and allogeneic HSCTs. There are various reasons that the success of HSCT has improved. In the setting of autologous HSCT, changes in conditioning regimens, hematopoietic growth factors, and the use of peripheral blood stem cells rather than bone marrow stem cells have been credited in part with improving mortality by shortening the duration of neutropenia (and incidence of severe infections) after intensive chemotherapy or radiation. In addition, better supportive care, antibiotic use, and blood product support have all improved outcomes for autologous HSCT. For allogeneic HSCT, CSA was introduced in the 1980s as an immunosuppressive agent limiting the severity of GVHD, making allogeneic donor HSCT practical. Decrease in severe GVHD has improved outcomes in allogeneic HSCT. Additionally, CMV accounted for high rates of mortality seen in patients treated with an allogeneic HSCT. Viral transmission can be limited by using screened “CMV-free” blood products for CMV-negative patients or using leukocyte-reduced blood products for transfusions. CMV-positive patients are treated with a prophylactic or, more recently, “preemptive” strategy using new high-sensitivity assays for CMV reactivation. These procedures have decreased the mortality associated with CMV interstitial pneumonitis. Post-transplantation cell growth factors have also been cited as improving outcomes in allogeneic HSCT patients. In allogeneic HSCT, advances have led to a decrease in overall mortality. The introduction of nonmyeloablative HSCT with RIC regimens allows for faster recovery of blood lineages and fewer complications post transplant. There are many patients who have survived HSCT for 5 years or more, and the future holds even greater promise as various transplantation techniques become further refined.
Table 20‐5 Patient survival rates for transplants performed 2008–2015.
Source: Based on Organ Procurement and Transplantation Network (OPTN). https://optn.transplant.hrsa.gov/data/view-data-reports/national-data/#. Accessed March 24, 2020.
Type of Survival | Type of Transplant | |||
---|---|---|---|---|
Renal (Living Donor) |
Renal (Cadaveric Donor) |
Liver (Cadaveric Donor) |
Lung (Cadaveric Donor) |
|
1-year graft survival (%) |
97.5 | 93.2 | 89.1 | 86.7 |
5-year graft survival (%) |
85.6 | 74.4 | 71.9 | 52.5 |
1-year patient survival (%) |
98.8 | 96.3 | 91.2 | 87.4 |
5-year patient survival (%) |
92.1 | 83.3 | 75.0 | 55.0 |
Outcomes of recipients who have received both bone marrow and solid organ transplantation have also been reviewed. There are many clinical and immunologic considerations that are highlighted by reviewing this unique patient population. Further research regarding the concept of immunologic tolerance/chimerism in patients who have had both a solid organ and an HSCT transplant may provide clues for future studies or for consideration of routine treatment regimens, including HSCT with the transplanted solid organ. Close monitoring of these patients will allow a better understanding of the concept of chimerism and tolerance.
ORAL HEALTH SEQUELAE IN TRANSPLANTATION
Oral complications post transplantation may affect the patient at any time. Some may subside and others may become chronic and be detrimental to patient quality of life. The following section will extend on each oral complication (Box 20-2).
Infections
Following transplantation, patients are more susceptible to bacterial, viral, and fungal infections, to include the oral cavity. Signs of oral infection may be muted by a decreased inflammatory response, or occasionally signs of infection may be exaggerated. Oral infection is often related to the level of immunosuppression and the patient’s ability to mount an immune response. Oral infections must be diagnosed and aggressively treated, as these localized infections can spread quickly. Furthermore, systemic infections can present as changes in the oral tissues and it is important to remember that in severely immunocompromised patients, infections could be caused by opportunistic microbes that are not usually associated with oral infection. Laboratory tests such as culture, polymerase chain reaction (PCR), and sensitivity testing are prudent to target the therapy to the source of the infection.

VIDEdental - Online dental courses
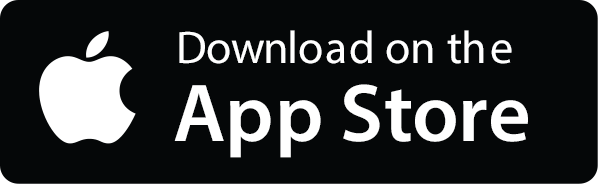
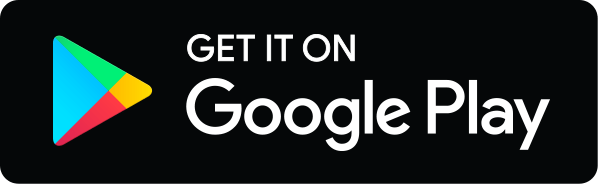