Abstract
The aim of this study was to evaluate the transdentinal cytotoxicity of experimental adhesive systems (EASs) with different hydrophilicity and dentin saturation solutions on odontoblast-like cells. One hundred 0.4-mm-thick dentin discs were mounted in in vitro pulp chambers and assigned to 10 groups. MDPC-23 cells were seeded onto the pulpal side of the discs, incubated for 48 h. The EASs with increasing hydrophilicity (R1, R2, R3 and R4) were applied to the occlusal side after etching and saturation of etched dentin with water or ethanol. R0 (no adhesive) served as controls. R1 is a non-solvated hydrophobic blend, R2 is similar to a simplified etch-and-rinse adhesive system and R3 and R4 are similar to self-etching adhesives. After 24 h, cell metabolism was evaluated by MTT assay ( n = 8 discs) and cell morphology was examined by SEM ( n = 2 discs). Type of cell death was identified by flow cytometry and the degree of monomer conversion (%DC) was determined by infrared spectroscopy (FTIR) after 10 s or 20 s of photoactivation. Data were analyzed by the Kruskal–Wallis and Mann–Whitney tests ( α = 0.05). Dentin saturation with ethanol resulted in higher necrotic cell death ratios for R2, R3 and R4 compared with water saturation, although R2 and R3 induced higher SDH production. Photoactivation for 20 s significantly improved the %DC of all EASs compared with 10 s. A significant positive correlation was observed between the degree of hydrophilicity and %DC. In conclusion, except for R1, dentin saturation with ethanol increased the cytotoxicity of EASs, as expressed by the induction of necrotic cell death.
1
Introduction
Several factors can influence the adhesion of polymeric materials to dentin substrate, such as the type, composition and application mode of adhesive systems, and the intrinsic characteristics of this tissue, which determine its regional heterogeneity. For this reason, resin bonding to dentin is not considered as safe and predictable as to enamel and therefore, producing a resin–dentin bond strong enough to resist oral conditions remains a challenge.
Collagen fibrils may remain exposed after formation of resin–dentin bonds due to incomplete impregnation of demineralized dentin by resin monomers . This occurs as a result of a series of factors namely gradual reduction of the interfibrillar spaces toward the mineralized dentin, the high molecular weight and hydrophilic nature of some resin monomers present in the adhesive systems and moisture gradient of dentin , which permit the occurrence of the phase separation phenomenon . In addition to these immediate factors, collagen fibrils may also be exposed due to long-term hydrolytic degradation of polymer and leaching of residual monomers and low-weight polymers. Once exposed, these fibrils become susceptible to hydrolytic and enzymatic degradation, which is mediated by dentin proteases (matrix metalloproteinases – MMPs and cathepsins). Although the effects of enzymatic degradation can be minimized by the simple incorporation of chlorhexidine to dentin bonding protocols , hydrolytic degradation also exerts a significant, and perhaps predominant, effect on resin–dentin bond deterioration, affecting not only the organic, but also the polymeric content.
The concept of dentin saturation with ethanol to improve dentin infiltration by hydrophobic monomers and subsequently reduce the susceptibility of the resin–dentin interface to hydrolytic degradation was introduced in 2007 . According to Tay et al. , the possibility of dentin infiltration by hydrophobic monomers, such as BisGMA, is based on changing the intrinsically hydrophilic nature of etched dentin to a more hydrophobic characteristic by replacing water by ethanol to maintain dehydrated collagen matrices in an extended state to facilitate resin infiltration, in a technique known as ethanol-wet bonding. A significant increase in the bond strength and durability of adhesive interfaces is observed when this technique is used. This result can be justified by a better resin–dentin infiltration and sealing of the collagen matrix, and minimizing the endogenous collagenolytic action of dentin MMPs . Additionally, a significant decrease of dentin permeability has been demonstrated after application of experimental adhesive systems (EASs) with known solubility parameters on demineralized dentin saturated with ethanol .
Although dentin saturation with an alcoholic solution has been shown to enhance monomer infiltration quantitatively and qualitatively and improve the long-term stability of resin–dentin bonds, it remains unclear whether this technique enhances the cytotoxicity of adhesive systems to pulp cells. It has been demonstrated that resin monomers can diffuse through dentin and reach the subjacent pulp tissue at concentrations high enough to elicit adverse reactions in a dose- and time-dependent manner. Resin monomers can induce apoptosis in vitro and cause untoward cellular and biological events such as changes in ATP synthesis and dentin matrix mineralization, immunological reactions and genetic alterations .
As ethanol-wet bonding facilitates resin infiltration, especially hydrophobic monomers, this study investigated whether this technique increases the cytotoxicity of EASs to cultured odontoblast-like cells. The null hypothesis is that EASs bonded to ethanol-saturated acid-etched dentin are no more cytotoxic than the same EASs bonded to water-saturated acid-etched dentin.
2
Materials and methods
2.1
Preparation of dentin discs
One hundred noncarious third molar human teeth free of cracks, defects or morphological alterations were obtained upon approval by the Ethics Committee of the School of Dentistry at Araraquara – UNESP (Protocol #25/09). After removal of surface-adhered debris, the teeth were stored in 0.12% thymol solution at 4 °C and used within 3 months after extraction.
On the day of the experiment roots were removed 2 mm above the cementoenamel junction by a precision cutting machine equipped with a water-cooled diamond saw (Isomet 1000, Buehler Ltda, Lake Bluff, IL, USA). Sequential transversal cuts were made until reaching a flat dentin surface immediately above the pulp horn region. For each tooth, one 0.5-mm-thick dentin disc was cut from the mid-crown dentin as close as possible to the pulp region but without the presence of pulp horn projections. The teeth were carefully examined with a stereoscopic microscope (SZX7, Olympus, São Paulo, SP, Brazil) to confirm the absence of enamel islets on the occlusal side and defects resulting from pulp horn projections on the pulpal side. Next, the occlusal side of the discs was manually polished with wet 320-grit silicon carbide paper to reach a final thickness of 0.4 mm, as measured with a digital caliper accurate to the nearest 0.01 mm (Mitutoyo South Americana Ltd, Suzano, SP, Brazil). The dentin discs ( n = 100) were stored in phosphate buffer saline (PBS, pH 7.2) at 4 °C, until the measurement of dentin permeability.
2.2
Measurement of dentin permeability (hydraulic conductance)
Dentin permeability was measured to permit a homogeneous distribution of the dentin discs into the groups, eliminating the influence of this variable on the analysis of transdentinal cytotoxicity of the EASs applied to the discs. The simplest form to measure dentin permeability is calculating its hydraulic conductance (Lp) by filtration. Permeability is calculated from the hydraulic conductance or ease of fluid flow through a surface with known area (e.g. dentin disc) and under a constant hydrostatic pressure within certain amount of time, using the following equation:
where Lp is a hydraulic conductance (μL cm −2 min −1 cm H 2 O −1 ); Jv is the volume of fluid in μL or distance traveled by a microbubble in mm; A is the dentin surface area in cm 2 ; Δ P is the pressure gradient in cm H 2 O; and t is the time in minutes.
For determination of the hydraulic conductance, the discs were individually placed in in vitro pulp chambers (IVPCs) developed originally by Hanks et al. . In all dentin discs, the area for measurement of hydraulic conductance was standardized as 0.28 cm 2 . Both sides of the discs were etched with 0.5 M EDTA (acid ethylenediaminetetraacetic), pH 7.2, for 60 s to remove the smear layer created during disc polishing, followed by copious rinsing with distilled water. A metallic cannula attached to the middle compartment of the IVPC was connected to a 180 cm column of water through a 0.5-mm-diameter polyethylene tubing. The disc remained under this pressure for 5 min, after which time the movement of a microbubble introduced through the cannula was recorded during 1 min and the obtained values (Jv) transformed into conductance values using the equation presented above.
After measurement of hydraulic conductance, the dentin discs were allocated to 10 groups of 10 discs each, in such a way that the statistical analysis of the hydraulic conductance data showed similar dentin permeability among the groups (ANOVA, p > 0.05). The discs were frozen individually in PBS (pH 7.2) until when they were used for analysis of cytotoxicity.
2.3
MDPC-23 cell culture on dentin discs
Odontoblast-like MDPC-23 cells were cultivated in Dulbecco’s modified Eagle’s medium (DMEM; Sigma–Aldrich Corp., St. Louis, MO, USA) supplemented with 10% fetal bovine serum (FBS; Cultilab, Campinas, SP, Brazil) and containing 100 IU/mL penicillin, 100 μg/mL streptomycin and 2 mmol/L glutamine (Gibco, Grand Island, NY, USA) in a humidified incubator with 5% CO 2 and 95% air at 37 °C (Isotemp Fisher Scientific, Pittsburgh, PA, USA). The MDPC-23 cells were sub-cultured every 3 days until an adequate number of cells were obtained for the study. All cell culture procedures were carried out in a vertical laminar flow chamber (Veco do Brasil Ind. Com. de Equip. Ltda, Campinas, SP, Brazil). In preparation for the cytotoxicity assays, the occlusal side of the discs was hand polished with 600-grit silicon carbide paper for 10 s to produce a fresh smear layer and simulate clinical condition. Then, the discs were mounted in modified IVPCs and both sterilized in ethylene oxide.
The odontoblast-like MDPC-23 cells (3 × 10 4 ) cultivated in plain DMEM medium were seeded onto the pulpal side of the dentin discs (0.28 cm 2 ). The IVPCs were placed in 24-well plates (COSTAR 3595 – Corning Incorporated, Corning, NY, USA) in an inverted position and maintained in an incubator with 5% CO 2 and 95% air at 37 °C for 48 h for adherence of cells on the pulpal dentin surface. After this time, the IVPCs were placed with the occlusal side of the discs turned upwards to receive the adhesive procedures.
2.4
Dentin saturation solutions and EASs
Four EASs (R1, R2, R3 and R4) with known increasing degrees of hydrophilicity were evaluated. These experimental adhesive systems have been used in many studies, which focused mainly on mechanical tests . The composition and Hoy’s solubility parameters of the EASs are presented in Table 1 . R1 is similar to the non-solvated hydrophobic component used in 3-step etch-and-rinse adhesive systems or 2-step self-etch systems. R2 represents the basic composition of a simplified etch-and-rinse adhesive system, and R3 and R4 contain carboxylic and phosphoric acid derivatives, respectively, representing simplified self-etch adhesive systems. R0 was the control group (no adhesive).
EAS composition a | Solubility parameter Non-solvated adhesive |
pH | ||||
---|---|---|---|---|---|---|
δ d | δ p | δ h | δ t | |||
R1 | 70.0% BisGMA 28.5% TEGDMA 1.0% EDMAB 0.25% CQ |
15.9 | 12.4 | 6.9 | 21.2 | ∼7 |
R2 | 70.0% BisGMA 28.74% HEMA 1.0% EDMAB 0.25% CQ |
15.6 | 13.0 | 8.5 | 22.1 | ∼7 |
R3 | 40.0% BisGMA 30.0% TCDM 28.75% HEMA 1.0% EDMAB 0.25% CQ |
16.2 | 13.5 | 9.0 | 23.0 | ∼4 |
R4 | 40.0% BisGMA 30.0% BisMP 28.75% HEMA 1.0% EDMAB 0.25% CQ |
15.1 | 13.5 | 11.1 | 23.1 | ∼3 |
a Cadenaro et al. . Effect of adhesive hydrophilicity and curing time on the permeability of resins bonded to water vs. ethanol-saturated acid etched dentin.
In all groups, the smear-covered dentin was etched with 35% phosphoric acid (Scotchbond Etchant, 3M ESPE, St. Paul, MN, USA) for 15 s, copiously rinsed with sterile distilled water for the same amount of time, and blotted dry with sterile absorbant paper to maintain a moist dentin surface. Then, 20 μL of distilled water or 100% ethanol were passively applied to dentin surface for 60 s and excess was removed with a sterile absorbant paper to keep dentin moist.
Two layers of each EAS were applied to the discs saturated with distilled water or ethanol. Five microliters of each EAS were first applied to dentin, gently scrubbed with a microbrush for 10 s, and other 5 μL were applied. All EASs were photoactivated for 20 s with a halogen light-curing unit (Optilux 500, Kerr Company, Orange, CA, USA) with 510 ± 10 mW/cm 2 light intensity, as measured with a curing radiometer.
All adhesive procedures were performed in a vertical laminar flow chamber to avoid microbial contamination. Immediately after the adhesive procedures, the IVPC were stored again in the incubator for additional 24 h.
2.5
Analysis of cell viability (SDH production – MTT assay)
Cell metabolism was evaluated using the methyltetrazolium (MTT) assay. This method determines the activity of succinic dehydrogenase (SDH) enzyme, which is a measure of cellular (mitochondrial) respiration, and can be considered as the metabolic rate of cells. Eight out of 10 discs were used for this analysis 24 h following application of EASs. The eluates (culture medium containing the EAS components that diffused through the dentin discs) were collected for subsequent analysis of type of cell death by flow cytometry. After the 24-h incubation, the discs were removed from the IVPCs and placed in new 24-well plates with the pulpal side containing the MDPC-23 cells turned upwards. For each disc, the culture medium was aspirated and replaced by 900 μL of fresh DMEM plus 100 μL of MTT solution (Sigma–Aldrich, St. Louis, MO, USA) (5 mg/mL sterile PBS). The cells in contact with the MTT solution were incubated at 37 °C for 4 h. Thereafter, the culture medium with the MTT solution was aspirated and replaced by 400 μL of acidified isopropanol solution (0.04 N HCl) in each well to dissolve the violet formazan crystals producing a homogeneous bluish solution resulting from the cleavage of the MTT salt ring by the SDH enzyme present in the mitochondria of viable cells. After agitation and confirmation of the homogeneity of the solutions, three 100 μL aliquots of each well were transferred to a 96-well plate (Costar Corp., Cambridge, MA, USA). Cell metabolism was evaluated by photometry as being proportional to the absorbance measured at 570 nm wavelengths with an ELISA plate reader (Thermo Plate, Nanshan District, Shenzhen, Gandong, China). The values obtained from the three aliquots were averaged to provide a single value for each sample. The first three wells of the plate were filled with 100 μL of the 0.04 N acidified isopropanol solution to determine the value corresponding to total light transmission, that is, the maximum value for decrease of cell metabolism. The absorbance was expressed in numerical values, which were subjected to statistical analysis to determine the effect of the EAS components on the mitochondrial activity of the cells.
2.6
Analysis of cell morphology by scanning electron microscopy (SEM)
The other two discs were used for SEM analysis 24 h following application of EASs. As described above, the eluates were collected for subsequent analysis of type of cell death by flow cytometry.
After the 24-h incubation period, the cells were fixed in 1 mL of 2.5% glutaraldehyde (Sigma–Aldrich) in PBS for 1 h at room temperature. Then, the glutaraldehyde was aspirated and the cells were washed with PBS and post-fixed with 1% osmium tetroxide (Electron Microscopy Science, Fort Washington, PA, USA) for 1 h at room temperature. The cells that remained adhered to the dentin substrate were washed again with PBS and then dehydrated in a series of increasing ethanol concentrations (30%, 50% and 70%, one time for 30 min each; 95% and 100%, two times for 60 min each). The cells were immersed for 60 min (three 20-min changes) in 1,1,1,3,3,3-hexamethyldisilazane (HMDS, ACROS Organics, Morris Plains, NY, USA). Finally, the specimens were mounted on metallic stubs and then stored in a desiccator for 24 h, and sputter-coated with a gold layer (SDC 050; Bal-Tec AG, Balzers, Germany), and their morphology was examined with a scanning electron microscope (DSM 960, Carl. Zeiss Inc., Oberkochen, Germany).
2.7
Flow cytometry analysis
The type of cell death was determined by the propidium iodide (PI) flow cytometry assay. The cells were analyzed by fluorescence-activated cell sorting (FACS) in a flow cytometer (FACSCanto; BD Biosciences, San Jose, CA, USA) equipped with argon laser and Cell Quest software (BD Biosciences, San Jose, CA, USA). For identification of the type of cell death, MDPC-23 cells (3 × 10 4 cells/cm 2 ) were cultured in 24-well plates for 48 h. Next, the culture medium was replaced by the eluates collected prior to the MTT assay and SEM analysis. After 24 h in contact with the test eluates, the cells were harvested with trypsin, centrifuged at 5000 rpm for 2 min to remove the supernatant and resuspended in 300 μL of ligation buffer containing 10 mM HEPES pH 7.4, 150 mM NaCl, 5 mM KCl, 1 mM MgCl 2 and 1.8 mM CaCl 2 . For acquisition of cells stained positively for apoptosis, an aliquot of 300 μL of cell suspension of each experimental condition was treated with anexin-V (Institute of Biomedical Sciences, São Paulo, SP, Brazil) at a concentration of 1:500 for 20 min in the dark. The samples were acquired in the FL-1 channel of the flow cytometer. Acquisition of cells stained positively for necrosis was made immediately after the addition of 3 μg/mL of propidium iodide (Sigma–Aldrich Corp., St. Louis, MO, USA) at a concentration of 100 μg of reagent per mL of buffering solution. The samples were acquired in the FL-2 channel of the flow cytometer. Data were obtained as percentage of the number of cells stained for each reagent from the total number of MDPC-23 cells recognized by the flow cytometer. One group (R+) served as an internal control of the method for identification of necrotic cell death, in which the cells were placed in contact with 29% hydrogen peroxide for 30 min before flow cytometry analysis. Data for statistical analysis were obtained as percentage of the number of cells stained by the fluorochrome (anexin or propidium iodide) in relation to the percentage of the total number of MDPC-23 cells identified by the flow cytometer.
2.8
Analysis of degree of monomer conversion
The degree of monomer conversion (%DC) was determined using a Fourier transform infrared (FTIR) spectrometer (Spectrum 100 Optica; PerkinElmer, MA, USA) equipped with an attenuated total eflectance (ATR) device, which is composed of a horizontal selenide zinc (SeZn) crystal (Pike Technologies, Madison, WI, USA). The %DC was calculated using previously established methods , based on the ratios between the peaks 1638 cm −1 (carbon double-bonds in aliphatic chains) and 1608 cm −1 (aromatic chains of carbon atoms that originate the benzene rings of monomer molecules, which are used as a reference since their intensity remains unaltered during the polymerization process). Three microliters of each EAS were applied directly to the horizontal face of the crystal and coated with a polyester strip to prevent the formation of an oxygen-inhibited layer. The graphs for calculation of baseline data (non-polymerized sample) were constructed and photoactivation (510 ± 10 mW/cm 2 , Optilux 500, Kerr Company, Orange, CA, USA) of specimens was performed for 10 s or 20 s, generating the graph representative of the values used for calculating the degree of monomer conversion. Five repetitions were made for each EAS and photoactivation time.
2.9
Statistical analysis
Data for each response variable (SDH enzyme production, apoptotic and necrotic cell death, and degree of monomer conversion) did not fulfill the requisites of normal distributions and homoscedasticity. Kruskal–Wallis and Mann–Whitney non-parametric tests for pairwise comparisons of groups were used, in comparing the variables factors “EAS”, “saturation solvent” and “photoactivation time”. The significance level was set at 5%.
2
Materials and methods
2.1
Preparation of dentin discs
One hundred noncarious third molar human teeth free of cracks, defects or morphological alterations were obtained upon approval by the Ethics Committee of the School of Dentistry at Araraquara – UNESP (Protocol #25/09). After removal of surface-adhered debris, the teeth were stored in 0.12% thymol solution at 4 °C and used within 3 months after extraction.
On the day of the experiment roots were removed 2 mm above the cementoenamel junction by a precision cutting machine equipped with a water-cooled diamond saw (Isomet 1000, Buehler Ltda, Lake Bluff, IL, USA). Sequential transversal cuts were made until reaching a flat dentin surface immediately above the pulp horn region. For each tooth, one 0.5-mm-thick dentin disc was cut from the mid-crown dentin as close as possible to the pulp region but without the presence of pulp horn projections. The teeth were carefully examined with a stereoscopic microscope (SZX7, Olympus, São Paulo, SP, Brazil) to confirm the absence of enamel islets on the occlusal side and defects resulting from pulp horn projections on the pulpal side. Next, the occlusal side of the discs was manually polished with wet 320-grit silicon carbide paper to reach a final thickness of 0.4 mm, as measured with a digital caliper accurate to the nearest 0.01 mm (Mitutoyo South Americana Ltd, Suzano, SP, Brazil). The dentin discs ( n = 100) were stored in phosphate buffer saline (PBS, pH 7.2) at 4 °C, until the measurement of dentin permeability.
2.2
Measurement of dentin permeability (hydraulic conductance)
Dentin permeability was measured to permit a homogeneous distribution of the dentin discs into the groups, eliminating the influence of this variable on the analysis of transdentinal cytotoxicity of the EASs applied to the discs. The simplest form to measure dentin permeability is calculating its hydraulic conductance (Lp) by filtration. Permeability is calculated from the hydraulic conductance or ease of fluid flow through a surface with known area (e.g. dentin disc) and under a constant hydrostatic pressure within certain amount of time, using the following equation:
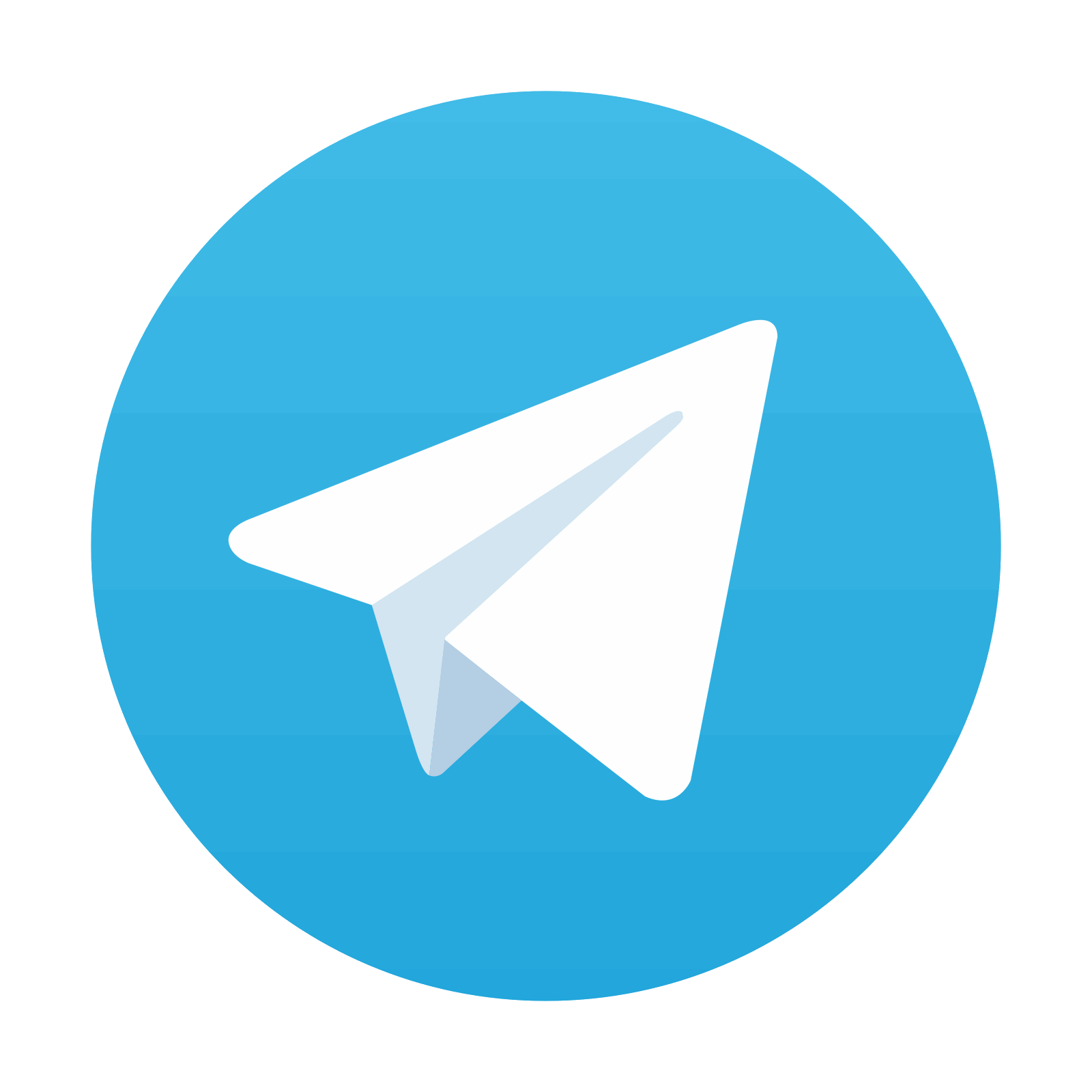
Stay updated, free dental videos. Join our Telegram channel

VIDEdental - Online dental courses
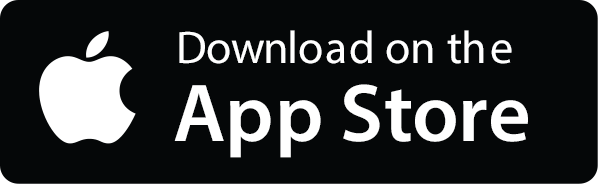
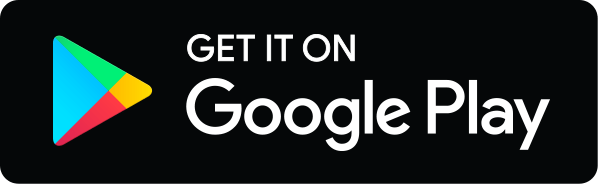