Soft and hard tissue engineering has expanded the frontiers of oral/maxillofacial augmentation. Soft tissue grafting enhancements include improving flap prevascularization and using stem cells and other cells to create not only the graft, but also the vascularization and soft tissue scaffolding for the graft. Hard tissue grafts have been enhanced by osteoinductive factors, such as bone morphogenic proteins, that have allowed the elimination of harvesting autogenous bone and thus decrease the need for other surgical sites. Advancements in bone graft scaffolds have developed via seeding with stem cells and improvement of the silica/calcium/phosphate composite to improve graft characteristics and healing.
Key points
- •
Soft tissue engineering includes the use of mesenchymal stem cells to develop tissue sheets for donor soft tissue graft and incorporate other cell types to create new vascular structures within the graft.
- •
Osteoconductive scaffolds mixed with osteoinductive growth factors, including osteogenic stem and osteoprogenitor cells, enhance maxillofacial bone reconstruction.
- •
Hard tissue engineering of bone grafts, such as those with a silica-calcium phosphate composite seeded with human adipose-derived stem cells, creates better grafts with enhanced resorption profiles.
Soft tissue engineering
Oral soft tissue engineering has long been associated with the reconstruction of mucosal or gingival defects associated with chronic disease or trauma, as well as congenital defects. The premise of the tissue engineering follows a requisite triad of cells, scaffolds, and physiologically active substances, along with angiogenesis and proper environmental factors/stimuli to induce tissue regeneration. This tenet holds true for both hard and soft tissues. Soft tissue augmentation has evolved beyond conventional autogenous-only grafts to a variety of donor grafts. The regeneration of soft tissue has just begun to tap its potential through advancements in stem cell technologies as well as cell signaling and cell trafficking. The focus here is to provide an overview of some key areas of these advancements.
Soft Tissue Augmentation
Soft tissue augmentation around implants has, for many years, focused on autogenous conventional epithelialized, free gingival grafts, or connective tissue grafts to gain keratinized tissue or address mucosal recession. Studies indicate that shallow recession defects (≤2 mm) have shown success; there is no support for larger defects. The adaptation of various tunneling techniques have led to minimal surgical access that produce both successful root coverage and esthetics. These minimally invasive procedures, such as the vestibular incision supraperiosteal tunnel access or modified vestibular incision supraperiosteal tunnel access technique, have been used to address defects around dental implants. Further studies are needed to better address outcomes in the esthetic zone.
Autogenous soft tissue grafts rely on donor sites, usually the hard palate, which can have significant sequelae, such as postoperative pain or bleeding after the procedure. A number of commercially available soft tissue grafts allow the elimination of harvesting the graft from the donor as well as the grafting of multiple sites in 1 visit. Allogenic human acellular dermal matrix is commonly used for soft tissue augmentation and has similar results to autogenous grafts. A common feature of several of these allogenic grafts is the preservation of the basement membrane. Xenografts, or those derived from other species, such as porcine or bovine species, have also been used. One such porcine collagen matrix studied in comparison to a free gingival graft showed mature collagen in the tissue samples from the porcine graft, whereas a more fragmented collagen tissue was seen in the free gingival graft samples.
Although enhancements of harvested donor tissues allow for a greater extent of grafting and less patient morbidity, regeneration relies on the manipulation of cells and tissues to maximize gain of lost tissues.
Soft Tissue Regeneration
Soft tissue regeneration blends the processes of wound healing with the enhancement of cell types and functions present during the phase of regeneration. A number of key cell processes, including proliferation, viability, migration, gene expression, and differentiation have been studied in in vitro monolayer models and 3-dimensional (3D) models provide insight into higher order activity, such as cell contractility in a fibroblast model. A 3D model was used to generate autologous cell sheets in a rat model. Oral mucosal cells, specifically fibroblasts, as well as fibrin were used to create scaffolds for mucosal keratinocytes. In this rat model, reepithelization via cell sheets with fibroblasts was much faster than those without fibroblasts. The authors concluded that not only was the overall process relatively fast, but also that the outcome included no scarring. The 3D tissue models have also provided a platform to study various oral infection models, such as oral candidiasis, allowing for a better understanding of cytokine release and gene expression of the tissues as well as the process of invasion of the epithelium by the candidiasis.
Tissue models rely on selected cells for specific applications. Mesenchymal stem cells (MSCs) give rise to various specialized cells. Recent efforts have focused on progenitor cells of the periodontal ligament (PDL) because these cells can form cementoblasts, osteoblasts, or fibroblasts. Differentiated induced pluripotent stem cells that turn into MSCs are generally safer than undifferentiated pluripotent stem cells, but there are limitations on obtaining adequate quantities of cells. Further research is needed to develop more sources of stem cells. Once an adequate source and quantity of therapeutic stem cells can be generated, the next challenge is getting the cells where they are needed. Local delivery may suffice if the defects are large and are accessed surgically; however, early defects may require attracting cells to the site. Enhancing attraction of the patient’s own cells may be done through endogenous cell homing via chemokines or scaffolds that influence the recruitment of specific cells. In a rat model, systemically transplanted MSCs were harvested from donor rats and implants were placed after tooth extractions. The authors noted improved attachment and proliferation of oral mucous epithelial cells. The PDL is known for its regenerative capacity to enhance the replacement of lost cementum and bone on natural teeth. A novel concept of developing a PDL on dental implants has been investigated. In a rat model, dental progenitor cells were used to seed and develop a PDL on titanium implants. Advancements in obtaining and maintaining a PDL on implants would be significant for regeneration around implant surfaces.
All augmentation procedures, both soft and hard tissue, rely on the establishment of vascular and nutrient supply after augmentation. The collection, harvesting, and delivery of specific cells would be of little use without an environment that allows proliferation and maturation. Assessment of various scaffolds via micro-computed tomography evaluation of perfusion can be done using a chorioallantoic membrane that allows for 3D imaging of the tissue engineered construct while reducing extensive animal use in research. Vascularized connective tissue grafts in humans, when compared with conventional subepithelial connective tissue grafts, showed less shrinkage and maintained their initial volume better. In another study, human adipose-derived cells and human umbilical vein endothelial cells were used to create vessel structures for vascularization of the engineered soft tissue flap.
Bone engineering
Repair of bone defects, both large and small, in the maxillofacial skeleton have proved to be a treatment challenge for many years. Although autogenous bone grafts have been considered the mainstay and gold standard of treatment, allografts, xenografts, and alloplastic substitutes have been used with success for resolving bony defects in the maxilla and the mandible. The traditional autogenous bone graft often required a donor site that added another source of morbidity to the patient. Searches for alternative methods that eliminate a donor site have been many and led to the understanding of osteoconductive and osteoinductive materials. Autogenous bone grafts stimulate bone regeneration through its osteogenic, osteoinductive, and osteoconductive properties, and avoid an immune reaction. In addition, autogenous grafts contain live stem or osteoprogenitor cells that can migrate, proliferate, and potentiate bone healing. An osteoinductive material can recruit immature cells and stimulate them to develop into osteoblasts. Although well beyond the scope of this article, several growth factors are involved in bone healing. They include vascular endothelial growth factor, fibroblastic growth factors, insulin-like growth factors, platelet-derived growth factor, and bone morphogenic proteins (BMP), to name a few. Their concentration, interaction with other growth factors, and the timing of their application is critical in normal bone regeneration.
Osteoconductive materials allow bone to grow on a surface and act as a scaffold. A scaffold should not only provide structural support, but also should be biodegradable at a rate comparable with that of newly formed bone and should have a porous structure similar to bone to promote capillary infiltration and cell proliferation. Tricalcium phosphate is an example of an osteoconductive material that will resorb with time.
Bone Morphogenic Proteins
BMPs are widely recognized for their important role in the postnatal bone formation. They are members of transforming growth factor-β group. Out of nearly 20 different BMPs that have been identified, BMP-2 and BMP-7 are the most extensively studied and are considered to possess the most significant osteoinductive properties.
Recombinant human BMP-2 (rhBMP-2) with an absorbable collagen sponge carrier is commercially available in the United States as INFUSE bone graft (Medtronic, Minneapolis, MN) and is approved by the US Food and Drug Administration. Current indications include sinus augmentations, alveolar ridge augmentation, and socket preservation procedures. A series of clinical trials have demonstrated the effectiveness of rhBMP-2/absorbable collagen sponge for maxillary sinus augmentation and alveolar ridge augmentation procedures. Separately, complete healing of alveolar grafting of premaxillary clefts was demonstrated in 10 patients treated with BMP-2/collagen sponges when compared with 2 control patients treated with conventional autogenous cancellous bone grafts.
Even though good evidence exists regarding the effectiveness of rhBMP-2, its safety has been questioned based on a metaanalysis of individual participant data by Simmonds and colleagues (2013). Their research was conducted in response to the finding from a review of clinical trials conducted by of Carragee and colleagues (2011), where the risk of adverse events seemed to be significantly higher than original estimates when study bias was taken into consideration.
Conway and associates (2014) compared the treatment of long bone union in 63 patients treated with BMP-2 versus 112 patients treated with BMP-7. They found that treatment with BMP-2 showed a better healing (15 weeks vs 23 weeks average time to weight bearing) and faster recovery (19 weeks vs 30 weeks average healing time) than treatment with BMP-7. In contrast, another comparison study discovered that rhBMP-7, especially in higher doses, showed less inflammation and soft tissue edema than rhBMP-2.
To deliver rhBMP-7 into the bony defect, bovine collagen is reconstituted with saline to form a paste or carboxymethylcellulose is added into the rhBMP-7/bovine collagen/saline paste to form a putty as a carrier. These are available as OP-1 Putty (Skryker Biotech, Hopkinton, MA) under limited approval from the US Food and Drug Administration. However, its use was discontinued in the United States in 2014 because the product failed to clearly demonstrate its efficacy in comparison with autogenous bone grafting. Nevertheless, the use of rhBMP-7 has continued in other countries with promising results. Ayoub and colleagues (2016) described a successful regeneration of alveolar cleft with the use of rhBMP-7.
One of the challenges with collagen-based carrier systems is a lack of strength to withstand forces exerted by the muscles of mastication. With increased pressure, the slow controlled and sustained release of BMPs can be compromised. With pressure, there is also an increased risk of the release of high doses of rhBMPs. Thus, BMPs and a collagen sponge have been studied in conjunction with porous solid osteoconductive scaffolds such as hydroxyapatite, tricalcium phosphate, and chitosan to achieve the required mechanical support.
Herford and colleagues (2012) combined recombinant BMP with a compression-resistant osteoconductive matrix in an animal model mandibular continuity defect. They showed a significantly higher bone density and space maintenance than just recombinant BMP2 and an absorbable collagen sponge. Their compression resistant matrix was composed of 15% hydroxyapatite and 85% β-tricalcium phosphate.
A number of studies that advocated for the use of osteoconductive scaffolds in combination with osteoinductive materials showed successful bone healing or regeneration that is comparable with those with autogenous bone grafts. However, in patients with limited native viable osteoprogenitor cells (such as osteoporotic bone or radiation-induced hypocellular bone), the addition of bone marrow aspirate may be beneficial. Melville and colleagues (2017) used recombinant BMP2 with an allogeneic avascular bone graft combined with a bone marrow aspirate with a poly-D, l -lactic acid mesh to reconstruct the maxillary alveolus in a patient following a traumatic maxillary defect. Six months later, they were able to successfully place 3 dental implants.
Clinical Examples
Previously, our group published a report on the use of reconstruction plates with the use of rhBMP2 and tricalcium phosphate for patients with fractures and continuity defects of the mandible. A successful outcome was achieved for 2 patients with no significant medical comorbidities, thus avoiding a secondary donor site. In 1 case, the defect was 4 cm. We present an additional case using a similar technique.
Six months after maxillary and mandibular osteotomies, a 40-year-old patient presented with an infection and shifting of the occlusion. After the resolution of the infection and loss of bone, a diagnosis of a nonunion on the left side of the mandible was made ( Fig. 1 ). A cone beam computerized tomogram was obtained that allowed for the fabrication of a 3D model with the patient in the proper occlusion. Intraoperatively, a 2-mm reconstruction plate was contoured to stabilize the segments ( Fig. 2 ). This patient is still under active treatment.


Bone Grafts: The Next Generation
Annually, more than 4 million bone grafting surgeries are performed worldwide, at an estimated cost of US$10 billion. In 2016, the global market for craniomaxillofacial implants was US$1.79 billion and is expected to reach US$2.49 billion by 2021. Despite the huge advances of the state-of-the-art bone graft technology, the reconstruction of large craniofacial and maxillofacial bone defects remains a major challenge. The use of autografts is currently the gold standard technique despite the associated mortality and morbidity problems. Cadaver bone grafts from bone banks is second in popularity after autografts; however, it carries risks of decreases in mineral density owing to osteoclastic resorption and immune reaction because of genetic differences. Synthetic grafts made of metals or polymers are not bioactive because they do not bond to bone or support bone cell function. The formation of discontinuous fibrous tissue at the interfaces with polymeric and metal implants has been reported in preclinical and clinical studies. Several studies have shown that excessive fibrous capsule formation at the bone–implant interface can prevent tissue integration, and lead to bone resorption and fracture and implant failure. Calcium phosphate ceramics such as hydroxyapatite and tricalcium phosphate are bioactive because they are able to enhance bone cell function and tissue formation. Nevertheless, a main concern in the application of hydroxyapatite bone grafts is the poor resorption. Several studies have reported fibrous encapsulation around hydroxyapatite ceramic particles inside alveolar bone. These limitations create a significant need for improvements in bone graft technology.
Tissue engineering approaches for bone reconstruction use porous implant materials, like calcium phosphate ceramics, as a carrier for bone cells and/or osteoinductive molecules. MSCs have been widely studied for bone tissue engineering because they are able to differentiate to bone-forming cells (osteoblasts) and participate in fracture healing. Animal models have indicated that harvesting cells from bone marrow and delivering high numbers of cells into a graft site is inadequate to ensure appropriate healing and bon regenration. Ideally, a bioactive ceramic carrier releases ions that signal the stem cells and induce their osteogenic differentiation. Culturing bone marrow MSCs onto osteoconductive or osteoinductive carrier matrix until they attach, spread, differentiate, and/or generate a specific amount of extracellular matrix in vitro before implantation has resulted in a significant improvement in outcomes. The repair of a critical-sized femoral segmental defect using MSCs was accomplished without adverse immune responses and without the use of immunosuppressive therapy. Other studies have shown the feasibility of treating children with osteogenesis imperfecta using isolated allogeneic bone marrow-derived mesenchymal cells without significant toxicity.
The successful use of stem cells in bone reconstruction depends on the use of a suitable carrier. In a comparative study, the bone healing effect of MSCs seeded on 2 matrices, bovine bone hydroxyapatite and α-tricalcium phosphate, was compared with that of an autologous bone transfer. Histomorphometric analyses showed that the bone volume in defects treated with tricalcium phosphate seeded with mesenchymal cells was comparable with that of defects treated with autogenous bone. However, although defects treated with autogenous bone showed uniform platforms of bone formation, bone formation in defects treated with tricalcium phosphate was associated with the ceramic particles. Defects treated with bovine bone hydroxyapatite (Bio-Oss) seeded with or without mesenchymal cells scored the lowest value of bone formation and highest value of fibrosis around the ceramic particles.
Human adipose-derived stem cells showed the ability to expand in vitro and to differentiate into osteogenic, chondrogenic, adipogenic, and myogenic mesenchymal cell lineage, in addition to others. Adipose-derived stem cells are more favorable for bone regeneration than other multipotent stem cells such as bone marrow MSCs owing to the relative ease of collection, abundance, high proliferation, and fast robust mineralization. The ability of silica-calcium phosphate composite (SCPC) scaffold seeded with human adipose-derived stem cells to regenerate bone in a critical size canine calvarial defect was evaluated (Ahmed El-Ghannam, Unpublished data, 2019). Bone tissue regeneration and graft material resorption was evaluated ay 3 and 6 months postoperative using computed tomography scanning and histology. Calvarial defects grafted with SCPC alone or SCPC-cell construct were grossly repaired, whereas a control ungrafted defect did not repair. Microscopically, the newly formed bone in the grafted defects was compact and integrated with the surrounding host tissues. Histomorphometric analysis demonstrated higher bone surface area and near complete graft material resorption in defects grafted with SCPC-cell hybrid compared with SCPC alone. Resorption of the graft material takes place by 2 mechanisms, solution mediated or cell mediated. These 2 mechanisms are intertwined; the dissolution of ions from bioactive ceramic into tissue fluids stimulates bone cell function and tissue formation. The enhanced resorbability of SCPC preseeded with differentiated osteoblasts points to the significant role played by these cells in the resorption of the graft material.
Vascularization of the newly formed bone is essential for tissue viability and functionality. The composition and porosity of the bone graft can facilitate vascularization and perfect the quality of the bone. Silicon ion release from the bioactive ceramic facilitates endothelization. Histologic analysis done on critical size saddle-type bone defects grafted with SCPC were noticeably vascularized. In addition, in vivo and in vitro studies showed that silica plays an important role in bone cell differentiation and mineralized tissue formation. A systematic increase of silica in calcium phosphate ceramic showed a significant increase in alkaline phosphatase activity and collagenous protein synthesis. Other studies have showed that silica plays important role in the synthesis and stabilization of collagen I. Calcium ions facilitate osteogenic gene expression and many cell functions, including mineralization of the collagenous bone matrix. Mineralization of the collagenous matrix is further enhanced by the alkaline pH created when the bioactive ceramic releases sodium ions into the surrounding tissue fluids.
The efficacy of MSCs matrix graft in promoting healing is attributed to multiple mechanisms including osteoconduction, osteoinduction, and osteogenesis. The 3D porous scaffold provides guided bone growth in 3 dimensions, similar to natural bone. In addition, protein adsorption and surface characteristics enhance cell adhesion and osteoblastic differentiation. The high number of differentiated cells preseeded on the porous matrix provides more bone-forming cells necessary to expedite abundant bone formation. Nevertheless, barriers to the application of this bone engineering technology in bone grafting is related to the absence of a therapeutic strategy for the sterilization, packaging, and preservation of an MSC matrix hybrid to make it readily available for grafting applications. Culture and expansion of cells in vitro before seeding on the matrix is a lengthy process with complicated logistics; therefore, it presents operational hurdles at the clinical stage. Moreover, the concentration of osteogenic cells from fresh bone marrow by selective adhesion is recommended to eliminate the remnant of the animal protein added to the tissue culture medium during cell expansion. Cryopreservation has been used for the long-term storage of MSCs, enabling cells to be expanded in culture when needed, with full retention of functionality after thawing. These studies clearly indicate that seeding porous biomaterial matrix with cryopreserved MSCs and using the MSC matrix construct as a bone graft is a promising approach that can enable enhanced bone reconstruction. Therefore, cryopreservation and storage of cell material constructs can maintain the osteogenic activities of stem cells and provide a strategy for unlimited supply of off-the-shelf tissue engineered bone graft for immediate use in craniofacial and maxillofacial surgeries.
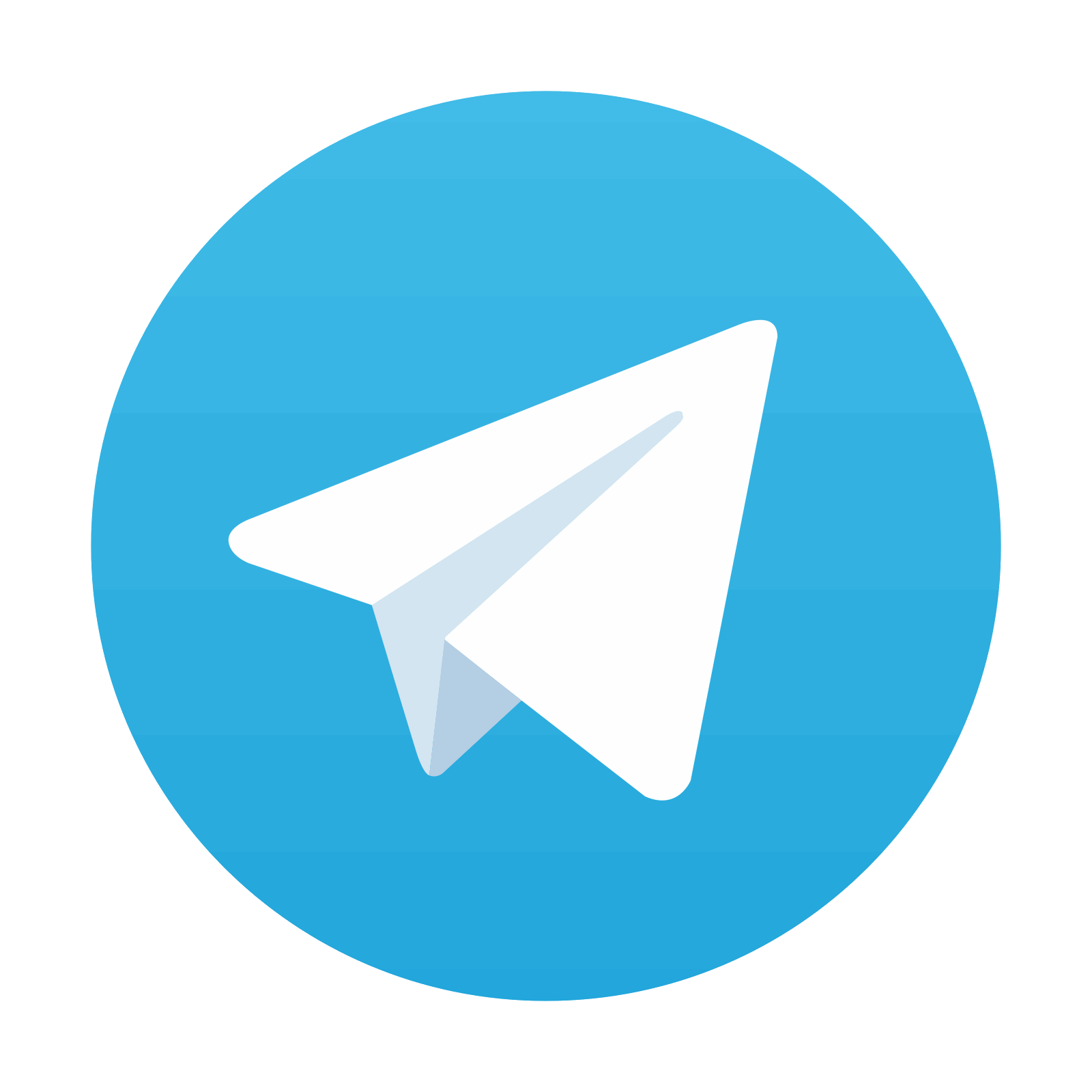
Stay updated, free dental videos. Join our Telegram channel

VIDEdental - Online dental courses
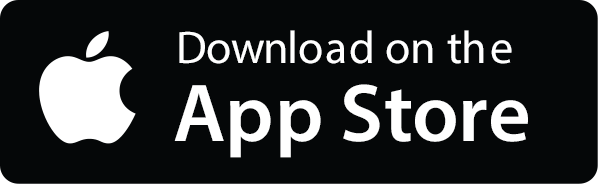
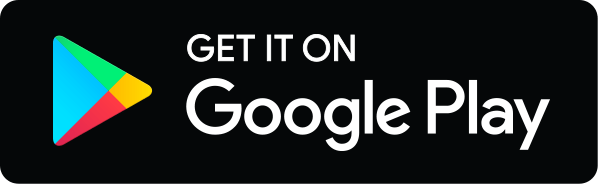