Introduction
The aim of this prospective study was to assess the 3-dimensional skeletal and dental effects induced by a new maxillary protraction approach using customized miniplates for anchorage compared with a control group of traditional tooth-borne maxillary protraction.
Methods
Forty-one growing patients at prepubescent stage with skeletal Class III malocclusion participated in this study. These patients were randomly split into 2 groups. In group 1 (n = 20), patients underwent maxillary protraction anchored with customized miniplates. The miniplates were individually designed and inserted using the surgical templates. In group 2 (n = 21), patients underwent tooth-borne maxillary protraction. Pretreatment and posttreatment cone-beam computed tomography scans were obtained, and 30 measurements of cone-beam computed tomography images were acquired and calculated. The changes after treatment and the comparison of the 2 groups were assessed.
Results
After maxillary protraction, group 1 showed a greater forward movement of the maxilla than group 2. The maxilla length increased more in group 1 than in group 2. The rotations of the palatal and mandibular planes in group 1 were less than those in group 2. In group 1, the maxillary incisors proclined less, the mandibular incisors retroclined less, and the maxillary first molars extruded less.
Conclusions
Compared with tooth-borne maxillary protraction, customized miniplates anchored maxillary protraction produced more maxillary growth, fewer dental changes, and less maxillary and mandibular plane rotation.
Highlights
- •
The 3-dimensional printed miniplates were stable and can be used in maxillary protraction.
- •
Bone anchorage induced more maxillary growth and fewer dental changes.
- •
Bone anchorage is more suitable for patients with hyperdivergent skeletal patterns.
Treatment of patients with a skeletal Class III malocclusion is difficult for the procedure is often long and complex, and malocclusion may relapse as patients grew up. Chincup therapy and maxillary protraction were considered 2 orthopedic approaches, which could treat an excessive mandible and a retrusive maxilla, respectively. However, chincup therapy was found to adjust the mandibular growth direction rather than inhibit the growth of the mandible. , Therefore, maxillary protraction has been recognized as the only orthopedic protocol for the correction of skeletal Class III malocclusion in patients in the prepubertal stage. However, there are some side effects, including more proclined maxillary incisors, more retroclined mandibular incisors, extrusion of the maxillary first molars, and rotation of mandibular plane, when it is performed using teeth and palate as anchorage. In an attempt to overcome the limitations of traditional maxillary protraction, some researchers used miniplates as anchorage. When orthopedic forces are directly applied to the maxilla, dental changes are minimal and skeletal effects may be better. De Clerck et al proposed a bone-anchored maxillary protraction method that used bone-anchored Class III traction and obtained favorable maxillary growth response in patients with Class III malocclusion. Many investigators have proclaimed orthopedic changes after bone-anchored maxillary protraction treatment.
However, because of the complex anatomic structure in mixed dentition and inadequate understanding of the anatomic structure of the implant area, injury to the root or tooth germ occasionally occurs during implant surgery, and the stability of the implant decreases with contact or penetration of the root. , Moreover, the surgical procedure for implanting miniplates is complicated and traumatic. From the cone-beam computed tomography (CBCT) images, the operator can know in detail of the bone thickness, bone density, and positions of anatomic structures such as roots and permanent tooth germs, before implantation, and then design an individualized miniplate. Furthermore, the position, direction, and length of miniscrews that are used to fix the miniplate can be designed and realized precisely with the help of a guide template.
Similar to the dental implant guide template system, Qiu et al designed an orthodontic microimplant guide plate to avoid damage of the root. Some studies have reported on orthognathic surgery guided by template, some researchers , have studied on the use of individualized titanium miniplates and guide plates for maxillary and mandibular orthognathic surgery.
However, there are few reports on the utilization of customized miniplates and guide templates in orthodontic treatment. This study is aimed to assess the 3-dimensional (3D) skeletal and dental effects induced by a new maxillary protraction approach using customized miniplates for anchorage, compared with a control group of subjects with traditional tooth-borne maxillary protraction Class III malocclusion.
Material and methods
Forty-one patients with skeletal Class III malocclusion participated in this study. The inclusion criteria for patient selection were as follows: maxillary deficiency (distance from point A to nasion perpendicular <0 mm) and skeletal Class III malocclusion; an Angle Class III occlusal relationship with an anterior crossbite; a concave lateral profile with midface deficiency; and prepubescent stage of skeletal age, as verified by the hand-wrist radiographs. The exclusion criteria were as follows: prior orthodontic or orthopedic treatment, other craniofacial anomalies or syndromes, and poor compliance of patient (patients who declared disagreement with the cooperation degree required for this treatment). This study was approved by the Institutional Review Board of Beijing Stomatological Hospital, Capital Medical University (institutional review board no. CMUSH-IRB-KJ-PJ-2017-04F). All patients submitted consent forms by their parents. Pretreatment (T1) and posttreatment (T2) CBCT scans were taken using the NewTom VGi system (Verona, Italy) with the following standardized parameters: 110 kV; 1.5-2.7 mA; 0.25 mm voxel size; scan time, 3.6 seconds; field of view of 15 cm in diameter × 15 cm in height; the number of images, 610; axial thickness, 0.250 mm; CTDIw, 1.72 mGy; and were stored in digital imaging and communications in medicine format.
The patients were randomly divided into 2 groups. All patients were treated by 2 operators (S.L and F.W). Before maxillary protraction, all participants underwent rapid maxillary expansion (RME) to disrupt midpalatal suture. The RME appliance was expanded twice per day (0.25 mm per turn) until the posterior buccal crossbite had been achieved. The traction forces were applied to each group on the day after RME, and the RME appliance was retained for 6 months to keep the expanded space.
Group 1 included 20 adolescent patients (8 boys, 12 girls; mean age, 10.75 ± 1.3 years; mean observation time, 10.6 months) treated with maxillary protraction anchored with customized miniplates on the maxillary anterior segment. Group 2 included 21 adolescent patients (11 boys, 10 girls; mean age, 10.5 ± 1.1 years; mean observation time, 12.1 months) treated with traditional maxillary protraction anchored with teeth.
In group 1, 2 customized miniplates were implanted in the anterior segment on both sides of the maxilla for each patient. The design and manufacturing process of the customized miniplate and the surgical template was as follows ( Fig 1 ): first, the individual safe area in the anterior segment of the maxilla was selected according to the cortical bone thickness, bone density, and positions of roots and permanent tooth germs from CBCT images, and the position and direction of miniscrews were determined (2-3 miniscrews for each miniplate) ( Fig 2 ). Second, 2 customized miniplates were designed according to the positions of miniscrews and the contour of cortical bone for each subject, with a hook located at the gingival margin above the maxillary canine. Then, surgical templates were designed to precisely realize the determined position and direction of miniscrews. These templates were designed according to the virtual implantation plans and positioned through maxillary posterior teeth. The accurate occlusal surface of the maxillary posterior teeth was acquired by superimposing the CBCT images with a digital model of maxillary dentition, which was scanned by a 3Shape scanner (3Shape, Copenhagen, Denmark). Finally, 3D printed customized miniplates and surgical templates. The customized miniplates and surgical templates were designed by using software 3-Matic (version 11.0; Materialise, Leuven, Belgium). The customized miniplates were 3D printed by Concept Laser M2 (Concept Laser GmbH, Lichtenfels, Bavaria, Germany) using titanium powder (Renishaw, New Mills, Gloucestershire, United Kingdom). The surgical templates were 3D printed by Objet Eden260VS (Stratasys, Eden Prairie, Minn) using resin VeroDentPlus (Stratasys).


The customized miniplates were inserted by the guidance of templates under local anesthesia in the maxilla ( Fig 3 ). Each miniplate was fixed to the bone with 2 or 3 titanium miniscrews (1.2-2.0 mm in diameter, 4-6 mm in length; Synthes, Zuchwil, Switzerland). Four weeks after insertion, traction forces were applied to the miniplates ( Fig 4 , A ).


Tooth-borne maxillary protraction ( Fig 4 , B ) was used in patients in group 2, and the RME appliance was bonded on the maxillary first molars and first premolars or deciduous first molars. Two hooks above maxillary canines on both sides were soldered to the RME appliance to receive elastics for protraction.
A 400-500 g of orthopedic force on each side was applied to each patient, and the direction of force was at 30° below the occlusal plane to offer a force through the resistance center of the nasomaxillary complex so that the maxillary counterclockwise rotation would be minimized. It was required to wear for at least 14 hours per day. Thirty-eight miniplates remained stable through the protraction process, and 2 miniplates showed mobility but could still bear the force until the treatment was completed.
Maxillary protraction was stopped when Class III molar relationship and the anterior crossbite were overcorrected. Then the miniplates were removed under local anesthesia.
T1 and T2 CBCT measurements were performed for each patient by 1 operator (S.L.) using software Simplant (version 13.0; Materialise). The landmarks included in this study were listed in Table I ( Fig 5 ). The 3D reference planes were as follows ( Fig 6 ): The Frankfort horizontal plane (FH plane) was determined by 3 points (OrL, OrR, and the midpoint of PoL and PoR), the midsagittal plane (MS plane) was crossed 2 points (S and N) and was vertical to the FH plane, the vertical plane (V plane) passed point S and was vertical to both the FH plane and the MS plane, the SN plane passed points S and N and was vertical to the MS plane, the palatal plane was crossed 2 points (ANS and PNS) and was vertical to the midsagittal plane. The measurements involved in this study were listed in Table II .
Landmark | Definition |
---|---|
A | The most posterior point in the concavity of the maxilla |
N | The most anterior point of the frontonasal suture |
B | The most posterior point in the concavity of the mandible |
S | Center point of sella turcica |
PNS | The most posterior point of the posterior nasal spine |
ANS | The most anterior point of the anterior nasal spine |
CoL/CoR | The most posterosuperior point of left/right mandibular condyle |
U1L/U1R | The midpoint of maxillary left/right first incisor’s incisal edge |
ApU1L/ApU1R | Root apex of maxillary left/right first incisor |
U6L/U6R | Mesiobuccal cusp tip of maxillary left/right first molar |
ApU6L/ApU6R | Mesiobuccal root apex of maxillary left/right first molar |
L1L/L1R | The midpoint of mandibular left/right first incisor’s incisal edge |
ApL1L/ApL1R | Root apex of mandibular left/right first incisor |
GoL/GoR | The most posteroinferior point of left/right mandibular angle |
PoL/PoR | The most superior point of left/right external acoustic meatus |
OrL/OrR | The most inferior point of the left/right infraorbital rim |
Pog | The most anterior point of the chin |
Me | The most inferior point of the chin |
Gn | The most anterior and inferior point on the contour of the mandibular symphysis |


Measurement | Definition |
---|---|
ANB angle (°) | The angle between points A-N-B |
SNA angle (°) | The angle between points S-N-A |
SNB angle (°) | The angle between points S-N-B |
PNS-A distance (mm) | Distance from PNS point to A point |
PNS-ANS distance (mm) | Distance from PNS point to ANS point |
MidCo-A distance (mm) | Distance from MidCo point (midpoint of CoL and CoR) to A point |
ANS-V plane distance (mm) | Distance from ANS point to the V plane |
A-V plane distance (mm) | Distance from A point to the V plane |
PNS-V plane distance (mm) | Distance from PNS point to the V plane |
MidU1-V plane distance (mm) | Distance from MidU1 point (midpoint of U1L and U1R) to the V plane |
U6L-V plane distance (mm) | Distance from U6L point to the V plane |
U6R-V plane distance (mm) | Distance from U6R point to the V plane |
B-V plane distance (mm) | Distance from B point to the V plane |
Pog-V plane distance (mm) | Distance from Pog point to the V plane |
MidL1-V plane distance (mm) | Distance from MidL1 point (midpoint of L1L and L1R) to the V plane |
SN-U1 angle (°) | The angle between the projection of line MidU1-MidApU1 (midpoint of ApU1L and ApU1R) to the MS plane and the SN plane |
U1-PP angle (°) | The angle between the projection of line MidU1-MidApU1 (midpoint of ApU1L and ApU1R)to the MS plane and the PP plane |
U1-PP distance (mm) | Distance from MidU1 point to the PP plane |
L1-GoGn angle (°) | The projection of angle between line MidL1-MidApL1 (midpoint of ApL1L and ApL1R) and line MidGo (midpoint of GoL and GoR)-Gn to the MS plane |
A-FH plane distance (mm) | Distance from A point to the FH plane |
ANS-FH plane distance (mm) | Distance from ANS point to the FH plane |
PNS-FH plane distance (mm) | Distance from PNS point to the FH plane |
Me-FH plane distance (mm) | Distance from Me point to the FH plane |
U1-FH plane distance (mm) | Distance from MidU1 point (midpoint of U1L and U1R) to the FH plane |
U6L-FH plane distance (mm) | Distance from U6L point to the FH plane |
U6L-PP plane distance (mm) | Distance from U6L point to the PP plane |
U6R-FH plane distance (mm) | Distance from U6R point to the FH plane |
U6R-PP plane distance (mm) | Distance from U6R point to the PP plane |
PP-SN angle (°) | The angle between the PP plane and the SN plane |
Mp-SN angle (°) | The angle between the projection of line MidGo-Gn to the MS plane and the SN plane |
Statistical analysis
SPSS software (version 22; IBM, Armonk, NY) was used to analyze all data. Shapiro-Wilk test was used to evaluate data distribution. The means and standard deviations of all measurements at T1 were calculated ( Table III ), and the changes of T2 and T1 were compared by paired t test (measurements fit normal distribution) or Wilcoxon rank test (measurements do not fit normal distribution). Differences between the 2 groups at T1 and T2 − T1 were calculated by independent sample t test (measurements fit normal distribution) or Mann-Whitney U test (measurements do not fit normal distribution). The significance level was set at P <0.05 for all tests. We have calculated the effect size, which equals to the mean difference (measurements fit normal distribution) or the median difference (measurements do not fit normal distribution) between the 2 groups ( Table IV ).
Measurement | Group 1 | Group 2 | P value | ||
---|---|---|---|---|---|
Mean | SD | Mean | SD | ||
ANB angle (°) | −3.07 | 1.82 | −2.76 | 1.78 | 0.394 ∗ |
SNA angle (°) | 78.78 | 2.99 | 78.65 | 2.13 | 0.933 † |
SNB angle (°) | 81.85 | 3.18 | 81.40 | 2.83 | 0.645 ∗ |
PNS-A distance (mm) | 42.21 | 2.10 | 42.37 | 2.55 | 0.837 ∗ |
PNS-ANS distance (mm) | 44.94 | 2.75 | 45.94 | 3.28 | 0.312 ∗ |
MidCo-A distance (mm) | 73.64 | 3.31 | 74.46 | 3.68 | 0.472 ∗ |
ANS-V plane distance (mm) | 63.03 | 3.77 | 65.32 | 3.32 | 0.211 † |
A-V plane distance (mm) | 59.87 | 3.30 | 61.28 | 3.10 | 0.160 † |
PNS-V plane distance (mm) | 18.14 | 2.49 | 19.44 | 2.92 | 0.126 † |
MidU1-V plane distance (mm) | 64.29 | 2.48 | 64.11 | 4.85 | 0.336 † |
U6L-V plane distance (mm) | 38.10 | 2.47 | 38.43 | 3.87 | 0.754 ∗ |
U6R-V plane distance (mm) | 38.69 | 3.02 | 38.14 | 4.02 | 0.635 ∗ |
B-V plane distance (mm) | 61.26 | 2.77 | 62.72 | 5.13 | 0.322 † |
Pog-V plane distance (mm) | 61.50 | 2.84 | 63.42 | 6.07 | 0.187 † |
MidL1-V plane distance (mm) | 65.35 | 1.84 | 66.38 | 4.96 | 0.574 † |
SN-U1 angle (°) | 75.43 | 5.05 | 76.29 | 8.28 | 0.699 ∗ |
U1-PP angle (°) | 66.24 | 4.47 | 67.86 | 8.39 | 0.431 † |
U1-PP distance (mm) | 26.02 | 2.50 | 25.47 | 2.36 | 0.384 † |
L1-GoGn angle (°) | 85.01 | 4.32 | 86.90 | 5.63 | 0.249 † |
A-FH plane distance (mm) | 27.21 | 2.76 | 28.24 | 3.50 | 0.314 ∗ |
ANS-FH plane distance (mm) | 21.17 | 2.79 | 21.65 | 2.40 | 0.319 † |
PNS-FH plane distance (mm) | 21.50 | 2.18 | 21.93 | 2.42 | 0.556 ∗ |
Me-FH plane distance (mm) | 83.42 | 6.84 | 81.08 | 5.96 | 0.263 ∗ |
U1-FH plane distance (mm) | 47.26 | 3.67 | 47.07 | 4.24 | 0.883 ∗ |
U6L-FH plane distance (mm) | 41.94 | 4.12 | 41.35 | 4.06 | 0.790 † |
U6L-PP plane distance (mm) | 20.63 | 2.87 | 19.59 | 2.40 | 0.224 ∗ |
U6R-FH plane distance (mm) | 41.84 | 3.39 | 41.42 | 3.71 | 0.716 ∗ |
U6R-PP plane distance (mm) | 20.51 | 2.34 | 19.61 | 1.92 | 0.195 ∗ |
PP-SN angle (°) | 9.19 | 2.92 | 8.70 | 3.34 | 0.629 ∗ |
Mp-SN angle (°) | 34.48 | 5.17 | 32.13 | 3.03 | 0.090 ∗ |
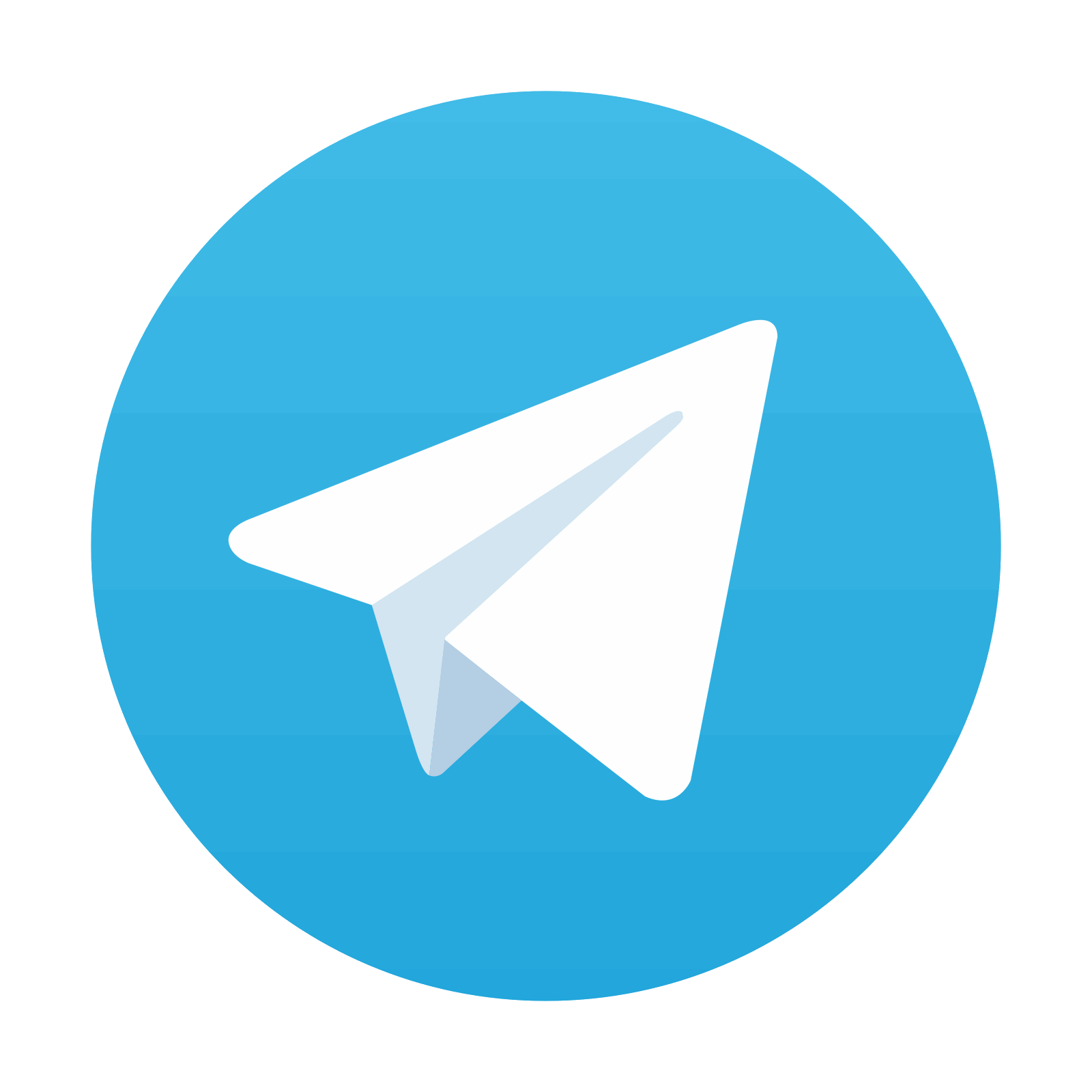
Stay updated, free dental videos. Join our Telegram channel

VIDEdental - Online dental courses
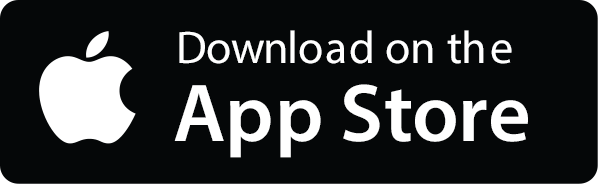
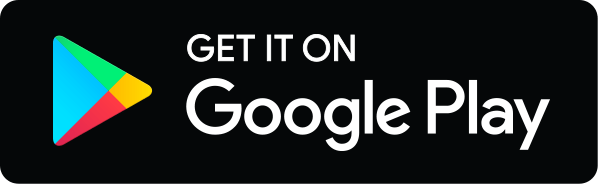
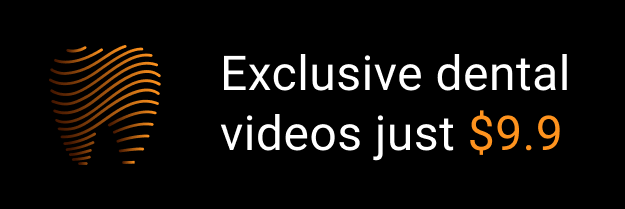