Introduction
This study aimed to assess the accuracy of virtual surgical planning (VSP) performed by Dolphin Imaging software (version 11.9; Dolphin Imaging and Management Solutions, Chatsworth, Calif).
Methods
Ten people requiring bimaxillary surgery and genioplasty were followed up prospectively. All patients had preoperative cone-beam computed tomography, plaster models, and photographs allowing for VSP. Interocclusal intermediate surgical splints were produced using a 3-dimensional (3D) printer. Postoperative images were acquired 15 days after surgery using cone-beam computed tomography. ITK-Snap (version 3.6; Cognitica, Philadelphia, Pa) allowed the segmentation of reliable 3D models. Geomagic Qualify 2013 (3D Systems, Rock Hill, SC) and MeshValmet (version 3.0) were used to identify the differences between VSP and actual surgical results through the root mean square values and the 3D translational displacement (3-axes) of the 3D centroid of each model.
Results
Discrepancies between the VSP and the actual result were found at the mandible ( P = 0.013) and the chin ( P = 0.013) when considering the root mean square values. In addition, 3D centroid differences were found in the transverse and sagittal direction of the right ramus ( P = 0.034 and P = 0.005, respectively) and the sagittal aspect of the left ramus ( P = 0.025). Considering 2 mm as a threshold of clinical relevance, almost all the bone fragments (maxilla, proximal, and distal mandibular segments) were accurately corrected by surgery, although not in the chin.
Conclusions
On the basis of the obtained values, it is possible to consider the Dolphin Imaging software as clinically acceptable for performing virtual orthognathic surgical planning.
Graphical abstract

Highlights
- •
The accuracy of Dolphin Imaging software for virtual surgical planning was assessed.
- •
The accuracy was evaluated using voxel-based superimposition.
- •
Translational displacements were measured in the 3 planes of space.
- •
Measurements were made on high-quality virtual 3D models generated by semiautomatic segmentation.
Orthognathic surgery is an integrated area of orthodontics and oral and maxillofacial surgery that aims at treating dentofacial deformities by repositioning the maxillomandibular complex to a more balanced, functional, and esthetically pleasing position. Because of the complexity of the procedure, accurate surgical planning is essential. ,
Advances in imaging, planning software, and prototyping technology have enabled the adoption of 3-dimensional (3D) virtual planning protocols and the manufacturing of prototyped splints. Thus, virtual surgical planning (VSP) offers new possibilities to visualize the relationship between the dental arches and its surrounding bones, in a unique virtual model. This approach presents several advantages when compared with conventional surgical planning, such as the visualization of deformities and asymmetries that sometimes are undetected, freedom to simulate distinct surgical procedures to obtain optimal results for the patient, detect possible complications, and ease in evaluating and correcting the centric relation in the temporomandibular joint. ,
To ensure the accuracy of the virtual planning, each employed software should be tested, given that they use different algorithms to operate. There are several methodologies to test the accuracy of orthosurgical treatment methods, but the most current and recommended methodology involves 3D assays using automatic or semiautomatic measuring techniques and assessments that decompose results across the different axes of space.
Dolphin Imaging software (version 11.9; Dolphin Imaging and Management Solutions, Chatsworth, Calif) is one of the most widely used software for VSP, so it is paramount to know its accuracy in such procedures. Unfortunately, studies using 3D evaluations, following adequate quality parameters, are not available in the literature. This study aimed to test the accuracy of VSP using Dolphin Imaging software.
Material and methods
This prospective clinical trial was reviewed and approved by the institutional review board. A sample calculation using a power of 90%, level of significance of 5%, and detected difference of 1.4 mm determined that a sample of 10 patients was needed.
The value of 1.4 mm was based on the spatial resolution error of the cone-beam computed tomography (CBCT) scans, which is equal to 0.7 mm. As we compared 2 different examinations, adding the errors of each examination, we reach the value of 1.4 mm. Thus, the sample was composed of 6 males and 4 females with a mean age of 26 ± 3.92 years and a diagnosis of Class II (n = 5) or Class III (n = 5) malocclusion.
All patients with a Class II malocclusion had ANB angles ranging from 6.4° to 10.8° and SN-GoGn angles from 37.1° to 46.9°. Only 1 patient presented a mild anterior open bite; 2 patients were asymmetric (one showed a mild maxillary cant, and the other showed a lateral mandibular discrepancy of 3 mm). In patients with a Class III malocclusion, ANB angle ranged from −1.8° to −6.4° and SN-GoGn angle from 22.4° to 50.6°; only 1 patient presented a mild anterior open bite; 2 exhibited clinical relevant asymmetries (1 presented an occlusal cant associated to a lateral shift of the mandible, and 1 displayed just a lateral mandibular asymmetry).
The sample selection was carried out consecutively by selecting the first 10 cases that met the study’s criteria. The inclusion criteria included: the presence of sufficient teeth to ensure surgical and postsurgical occlusal stability, with at least 2 stable occlusal contact points (tripod contact) on each side; the need for bimaxillary surgery; maxillary surgery with LeFort I osteotomy; mandibular surgery with bilateral sagittal osteotomy; chin surgery; use of rigid fixation in all segments; and adult patients, lacking remaining growth. The exclusion criteria included: a history of maxillary bone surgery, facial trauma or bone pathologies syndromes, and joint or autoimmune diseases.
All patients were submitted to a CBCT, frontal and lateral facial photographs, intraoral photographs, and dental plaster models for the presurgical planning (T1), and another CBCT 15 days after surgery (T2). Full head scans were obtained using iCAT Classic software (Image Sciences, Hatfield, Pa), with a voxel size of 0.3 mm and patients maintained in centric occlusion.
The dental plaster casts were digitized using the Maestro 3D MDS 350 scanner (Age Solutions, Pontedera, Pisa, Italy). The virtual files of the scanned models were imported to Dolphin Imaging software and merged into the teeth of the tomographic image, following the workflow proposed by the software. All the scanned models were trimmed beneath the orthodontic wire, excluding areas that could be distorted in the impression and leaving only the useful teeth surface for proper occlusal splint fabrication. After superimposing the trimmed scanned models to the segmented occlusal surfaces from the CBCT scans, the results were verified in the sagittal, axial, and coronal multiplanar reconstructions to ensure an adequate registration between both.
VSP was performed with the Dolphin Imaging software. The CBCT scans were guided according to the natural head position of each patient. The surgical occlusal splints (wafers) were saved in stereolithography (STL) file format and printed using a computer-aided design–computer-aided manufacturing system with photo-activated resin (OrthoDeskprinter; Stratasys, Ltd, Eden Prairie, Minn).
All the patients were operated by surgeons that belonged to the same department, using the protocol created for the research project, to standardize the procedures. All surgeons had significant experience with conventional and virtual orthognathic surgery. Such expertise should be attributed not only to the large number of surgeries performed annually by the service but also the constant evaluation of the results.
After obtaining the 2 CBCT scans (T1 and T2) and the surgical simulation, the 3 were submitted to a sequence of steps using different software, to perform an accurate 3D comparison between them.
The CBCT voxels were downsized to 0.4 mm because of the limitation of Dolphin Imaging software in superimposing images with a greater resolution. Then, the postoperative scan was superimposed onto the preoperative CBCT (voxel-based), which had already been oriented according to the patient’s natural head position. A presurgical bone model of one-eighth of a skull was cropped by the current sagittal, coronal, and axial planes creating a coordinate system transfer model (CTM) for future use in the creation of the Cartesian Coordinate System in Geomagic Qualify 2013 (3D Systems, Rock Hill, SC) ( Fig 1 ). After the previously described steps, the segments of the virtual surgical simulation and the CTM were exported in the STL file format.

Next, accurate 3D virtual models of each of the CBCTs (T1 and T2) of each patient were generated using the ITK-SNAP free access software ( http://www.itksnap.org/pmwiki/pmwiki.php ) by semiautomatic segmentation. The tomographic segmentations were performed in regions of interest for the surgery evaluation, such as the maxilla below the LeFort I osteotomy region, the mandibular body up to the first molar region including the chin, and both mandibular rami above the lingula (bilaterally). Each region generated individual files of each fragment in the STL file format.
The Geomagic Qualify was used to compare what was planned with what was achieved in the actual surgery. For this purpose, the virtual planning, the CTM segments, and the pre- and postsurgical fragments generated in ITK-SNAP were imported to this software.
In the next step, all the segments of the surgical simulation and T2 of each region were duplicated, and superimposed (best fit), so that they were all cut together concomitantly and had the same limits. These models were then brought back to its initial position (defined by voxel-based superposition at the cranial bases) ( Fig 2 ).

At this time, a specific coordinate system was created using the CTM as a reference, allowing Geomagic Qualify to use the same Dolphin Imaging software coordinate system. By using the 2 segments of each region (cropped with the same limits and resolution), it was possible to determine the centroid point. The centroid of an anatomic region is automatically calculated, by the software, considering the average spatial position of all centroids of the triangles that make up the mesh and, in turn, the centroid of each triangle consists of the meeting point of its 3 medians. Thus, the 3D centroid is a point that systematically represents the 3D spatial position of the anatomic region of interest, in relation to the 3 planes of space ( Fig 3 ). By eliminating the manual landmark placement, it was possible to prevent inaccuracies that could be incorporated by the operator.

To verify the reproducibility of the quantitative evaluation performed by the Geomagic Qualify 2013 software, we randomly simulated virtual surgeries for all the patients in the sample. A set of vertical, lateral, and sagittal movements in the maxilla and mandible were selected for each patient, respecting the limits described by Proffit and Ackerman’s discrepancy envelope. To compare the position of the centroids in the x, y, and z coordinates of the maxillary and mandible at presurgery with the generated surgical simulation, the displacement of each structure in each direction was obtained and compared with what had been planned in the Dolphin Imaging software surgical simulation module. Intra- and interexaminer correlations were conducted for the measurements, achieving an intraclass correlation coefficient of ≥0.99 in all the cases.
All the fragments processed in Geomagic were converted into the open inventor file format, and its position shifts over time were quantified in the MeshValmet software (version 3.0). The root mean square (RMS) values were recorded ( Fig 4 ).

The RMS value provided data regarding the absolute displacement of the fragments without considering the displacement direction, whereas the centroid values tracked the translational changes of the fragments in the 3 spatial planes.
Statistical analysis
The statistical analyses were performed using SPSS for Windows (version 23; IBM Corp, Armonk, NY). Additional graphics were generated in the MedCalc software (version 17.4; MedCalc Software, Mariakerke, Belgium). Sample normality was assessed using the Shapiro-Wilk test. Because the distribution of the sample regarding the RMS values proved to be asymmetrical for 2 of the 5 measurements, the Wilcoxon test was used to evaluate these measures. The distribution was normal for most of the coordinate values of the centroid measurements in the x, y, and z axes, so the paired t test was applied, complemented by Bland-Altman and box plots.
Results
The sample consisted of 10 patients, all of whom underwent bimaxillary orthognathic surgery with genioplasty. A total of 4 segments (maxilla, mandible body, right ramus, and left ramus) were evaluated in each patient ( Fig 5 ). The Altman-Bland graphs show the mean deviation between the planned and the obtained position for each region ( Fig 6 ).


When comparing the position achieved in orthognathic surgery with the expected one in virtual planning, evaluated by the RMS value of each structure, the results showed no statistically significant difference concerning the segments of the maxilla and ramus. However, a significant difference was observed for the distal mandibular segments ( P = 0.013), as shown in Table I .
Error threshold of the tests | Z | P |
---|---|---|
RMS-maxilla | −1.172 | 0.241 |
RMS-mandible | −2.497 | 0.013 |
RMS-right ramus | −1.274 | 0.203 |
RMS-left ramus | −0.866 | 0.386 |
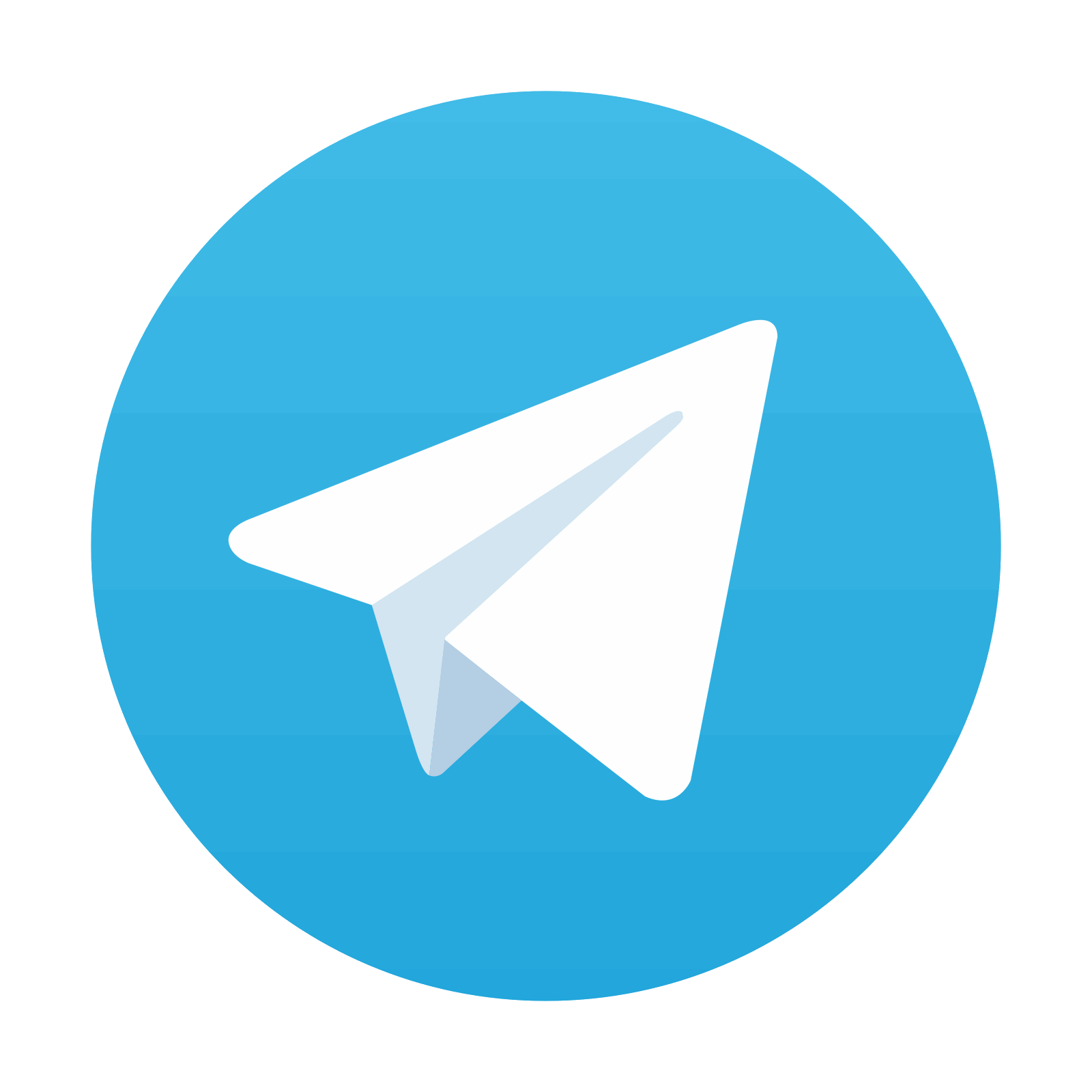
Stay updated, free dental videos. Join our Telegram channel

VIDEdental - Online dental courses
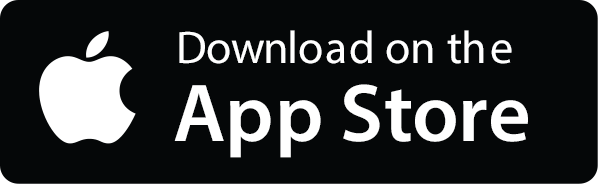
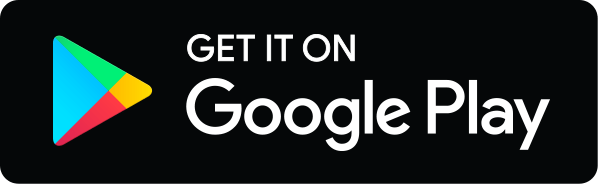
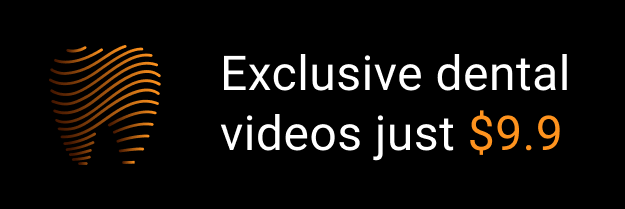