Abstract
The autoclaving, pasteurization, and freezing of bone grafts to remove bacteria and viruses, and for preservation, respectively, is considered to alter biological properties during graft consolidation. Fresh bone grafts release paracrine-like signals that are considered to support tissue regeneration. However, the impact of the autoclaving, pasteurization, and freezing of bone grafts on paracrine signals remains unknown. Therefore, conditioned medium was prepared from porcine cortical bone chips that had undergone thermal processing. The biological properties of the bone-conditioned medium were assessed by examining the changes in expression of target genes in oral fibroblasts. The data showed that conditioned medium obtained from bone chips that had undergone pasteurization and freezing changed the expression of adrenomedullin, pentraxin 3, BTB/POZ domain-containing protein 11, interleukin 11, NADPH oxidase 4, and proteoglycan 4 by at least five-fold in oral fibroblasts. Bone-conditioned medium obtained from autoclaved bone chips, however, failed to change the expression of the respective genes. Also, when bone-conditioned medium was prepared from fresh bone chips, autoclaving blocked the capacity of bone-conditioned medium to modulate gene expression. These in vitro results suggest that pasteurization and freezing of bone grafts preserve the release of biologically active paracrine signals, but autoclaving does not.
Autologous bone is used widely to bridge defects that have occurred as a consequence of malformation, resection surgery, or reconstructive trauma surgery, and prior to implant placement. Autologous bone has three potential characteristics that explain its favourable behaviour during graft consolidation. First, autologous bone is osteoconductive, providing a surface for the newly formed bone. Secondly, autologous bone is considered osteogenic when transplanted mesenchymal cells differentiate into bone-forming osteoblasts. Finally, autologous bone is considered osteoinductive when growth factors entombed in the matrix initiate the process of endochondral or intramembranous bone formation. However, there is another possible aspect: autologous bone, even when periosteal and endosteal cells have been removed, has a paracrine-like function.
Autologous bone grafting has drawbacks, such as an increased operation time, infection risk, morbidity at the donor site, and a limited supply of donor bone. Bone allografts can overcome these limitations. However, allografts carry the risk of viral transmission. For virus inactivation, bone allografts are frequently pasteurized, followed by freezing to allow storing in a bone bank. Moreover, bone allografts can also be subjected to autoclaving in order to prepare sterile bone grafts. The thermal processing of the originally vital bone by (1) autoclaving, (2) pasteurization, and (3) freezing could presumably affect the characteristics of the bone grafts, which in turn could modulate the process of graft consolidation. The underlying molecular mechanisms, however, remain unknown.
Pioneering studies on the use of autoclaved cadaveric bone in orthopaedic surgery were reported in the 1960s. Since then, autoclaving of autologous bone grafts has been described for use in the craniofacial region, such as in orbital reconstruction. The use of autoclaved tumour-bearing bone has been proposed for bone reconstruction, including reconstruction of the craniofacial skeleton. However, autoclaved human cortical bone has limited mechanical and osteoconductive properties. In a rabbit bone healing study, autoclaved bone gave less favourable results compared to pasteurized bone. In another study, autoclaved partially demineralized bone matrix demonstrated a weaker performance than when process by gamma irradiation, ethylene oxide, or ethanol in preclinical testing. It is thus likely that the extent of thermal processing affects the biological properties of the bone grafts.
Favourable long-term clinical results have been observed with pasteurization of mandibular tumour-bearing bone and other sources, such as pelvic grafts. Pasteurized bone has been used for reconstruction after resection of malignant peri-acetabular tumours, bone and soft tissue sarcomas, and distal tibiae with ‘en bloc’ intra-articular excision and ankle arthrodesis. Bone processing also involves freezing. Fresh frozen bone has been used for sinus floor elevation. Fresh frozen bone is harvested from human cadavers, then processed immediately and stored. Fresh frozen bone promotes bone formation during implant osseointegration. Interestingly, cell viability is partially preserved in frozen bone. Hence, pasteurization and freezing are commonly used procedures to preserve bone grafts. However, the impact of thermal processing on the paracrine activity of bone grafts remains to be determined.
Autologous bone from cortical sources has a paracrine function. It has been shown that when mesenchymal cells are exposed to bone-conditioned medium (BCM), a panel of strongly regulated genes becomes evident, including adrenomedullin (ADM), pentraxin 3 (PTX3), BTB/POZ domain-containing protein 11 (BTBD11), interleukin 11 (IL-11), NADPH oxidase 4 (NOX4), and proteoglycan 4 (PRG4). This panel of genes provides the scientific basis to establish a bioassay to determine the paracrine activity of bone grafts that have undergone autoclaving, pasteurization, and freezing. The aim of this study was to evaluate the impact of thermal processing on the paracrine activity released from autoclaved, pasteurized, and frozen bone grafts.
Materials and methods
Bone-conditioned medium (BCM)
Bone was obtained from adult pigs within 6 h post-mortem (Metzgerei Balsiger, Wattenwil, Switzerland). Bone chips were harvested from the buccal-side mandibular cortical bone with a bone scraper (Hu-Friedy, Rotterdam, Netherlands) and placed into sterile plastic dishes containing Dulbecco’s modified Eagle’s medium (DMEM) supplemented with antibiotics and antimycotics (all from Invitrogen Corporation, Carlsbad, CA, USA). Three independent preparations of bone chips were available. The harvested bone chips were subjected to four different conditions: (1) autoclaving at 121 °C for 20 min; (2) pasteurization at 80 °C for 30 min; (3) freezing at −80 °C for 30 min; (4) no processing. A ratio of 5 g bone chips (wet weight) per 10 ml culture medium was used. BCM was collected after 24 h of incubation at 37 °C in a humidified atmosphere and 5% carbon dioxide, sterile filtered (0.22 μm pore diameter; Merck Millipore, Billerica, MA, USA), and kept frozen at −80 °C. BCM preparations were termed ‘autoclaved bone BCM’, ‘pasteurized bone BCM’, ‘frozen bone BCM’, and ‘fresh bone BCM’, respectively. The stocks were thawed immediately before each experiment. For the indicated experiments, fresh bone BCM was autoclaved at 121 °C for 20 min; this preparation was termed ‘autoclaved BCM’. As negative control, conditioned medium of bone substitutes (60% α-tricalcium phosphate and 40% hydroxyapatite; Vivos Dental AG, Villaz-St-Pierre, Switzerland) was prepared according to the standard protocol.
Primary mesenchymal cells and cell lines
In the same way as reported previously, human gingival and periodontal ligament fibroblasts were prepared from tissue grafts obtained from two independent donors after wisdom tooth extraction. The Kantonale Ethikkommission of Bern approved the study protocol. Primary porcine bone-derived cells were allowed to emigrate from bone chips in explant cultures. The ST2 cell line – stromal cells isolated from mouse bone marrow – was obtained from RIKEN Cell Bank (Tsukuba, Japan). All cells were cultured in a humidified atmosphere at 37 °C in growth medium consisting of DMEM, 10% foetal calf serum (FCS; Invitrogen), and antibiotics. For all experiments, cells were plated in growth medium at 30,000 cells/cm 2 into culture dishes and then exposed to 20% BCM or serum-free medium alone for 24 h before the analysis of gene expression. For the time–response experiment, cells were exposed for 1, 3, 6, 12, and 24 h, respectively. In one experiment, the gingival fibroblast culture supernatant was used for IL-11 immunoassay (Enzo Life Sciences AG, Lausen, Switzerland).
Quantitative reverse transcription polymerase chain reaction (qRT-PCR) analysis
Cellular RNA was isolated with the High Pure RNA Isolation Kit (Hoffmann-La Roche, Basel, Switzerland). Reverse transcription was performed with Transcriptor Universal cDNA Master (Hoffmann-La Roche), and the PCR was done with FastStart Universal SYBR Green Master (Hoffmann-La Roche) on a 7500 Real-Time PCR System (Applied Biosystems, Life Technologies Corporation, Carlsbad, CA, USA). The probes are listed in Table 1 . mRNA levels were calculated by normalizing to the housekeeping gene beta-actin using the ΔΔCt method.
Genes | Forward primer | Reverse primer |
---|---|---|
hADM | GGACATGAAGGGTGCCTCTC | TGTTCATGCTCTGGCGGTAG |
hPTX3 | TGTATGTGAATTTGGACAACGAA | CATTCCGAGTGCTCCTGAC |
hBTBD11 | CAAAGCACTCCTCTCCAGCA | AATGAGCAGTGACTCTGGGC |
hIL-11 | TGCACCTGACACTTGACTGG | AGTCTTCAGCAGCAGCAGTC |
hNOX4 | TCTTGGCTTACCTCCGAGGA | CTCCTGGTTCTCCTGCTTGG |
hPRG4 | TCGTGATTCAGCAAGTTTCATC | CAGTTGCAGGTGGCATCTC |
hβ-actin | CCAACCGCGAGAAGATGA | CCAGAGGCGTACAGGGATAG |
susADM | CCCTGCATTTTCAGGAACC | GCTGGATTAAGCCGAAGAGA |
susPTX3 | GGTGCTCGAGGAGCTGAG | TTCTTGGAACGCATGGGGAA |
susBTBD11 | CAGCGCATCCTGAGTCATC | CATCCTCGCAGCATCTCTAA |
susIL-11 | CCAGCTGACGGAGATCGTAA | AGGAAAACAGGTCTGCTCGC |
susNOX4 | TCGCTTTTTGTCACACATGG | AGGGAGATGTGACTCCGTTTT |
susPRG4 | GAAGCAAAGTCCCACCAGTC | GAAGCTGGCTCCTTGACG |
susGAPDH | ACAGACAGCCGTGTGTTCC | ACCTTCACCATCGTGTCTCA |
mALPL | AGAACCCCAAAGGCTTCTTC | TCATGATGTCCGTGGTCAAT |
mβ-actin | CTAAGGCCAACCGTGAAAAG | ACCAGAGGCATACAGGGACA |
Alkaline phosphatase
For histochemical staining of alkaline phosphatase, ST2 cells were incubated in a serum-free medium with and without 20% BCM. Alkaline phosphatase staining was performed after 3 days. Cells were fixed as indicated and incubated with a substrate solution containing naphthol AS-TR phosphate and Fast Blue BB Salt (Sigma–Aldrich). After rinsing with distilled water, cultures were photographed. For the respective gene expression analysis, the cell line was exposed to 20% BCM for 24 h.
Statistical analysis
Experiments were repeated at least twice and data reported as the mean ± standard deviation. Analysis of variance (ANOVA) and post hoc testing with Fisher’s least significant difference (LSD) test were used for the analyses shown in Figs 1 and 2 . The t -test was used for the analyses shown in Figs 3 and 4 . Statistical significance was established at P < 0.05.




Results
Autoclaved bone BCM failed to affect gene expression in human fibroblasts
The time–response curve revealed a rapid cell response, peaking at between 6 and 24 h, depending on the target gene ( Table 2 ). All further experiments were performed with an incubation period of 24 h.
Time | |||||
---|---|---|---|---|---|
1 h | 3 h | 6 h | 12 h | 24 h | |
ADM | 0.61 ± 0.16 | 0.10 ± 0.03 | 0.05 ± 0.02 | 0.09 ± 0.06 | 0.23 ± 0.09 |
PTX3 | 0.81 ± 0.18 | 0.52 ± 0.24 | 0.21 ± 0.09 | 0.04 ± 0.01 | 0.13 ± 0.03 |
BTBD11 | 1.13 ± 0.01 | 11.88 ± 5.54 | 21.36 ± 9.68 | 7.25 ± 3.88 | 13.11 ± 7.88 |
IL-11 | 27.99 ± 2.20 | 36.66 ± 7.54 | 78.04 ± 28.31 | 107.53 ± 30.49 | 9.99 ± 5.47 |
NOX4 | 1.23 ± 0.02 | 6.86 ± 1.44 | 19.56 ± 4.02 | 26.87 ± 1.41 | 18.19 ± 4.51 |
PRG4 | 0.58 ± 0.03 | 4.71 ± 2.42 | 15.36 ± 11.14 | 23.46 ± 5.93 | 49.01 ± 13.64 |
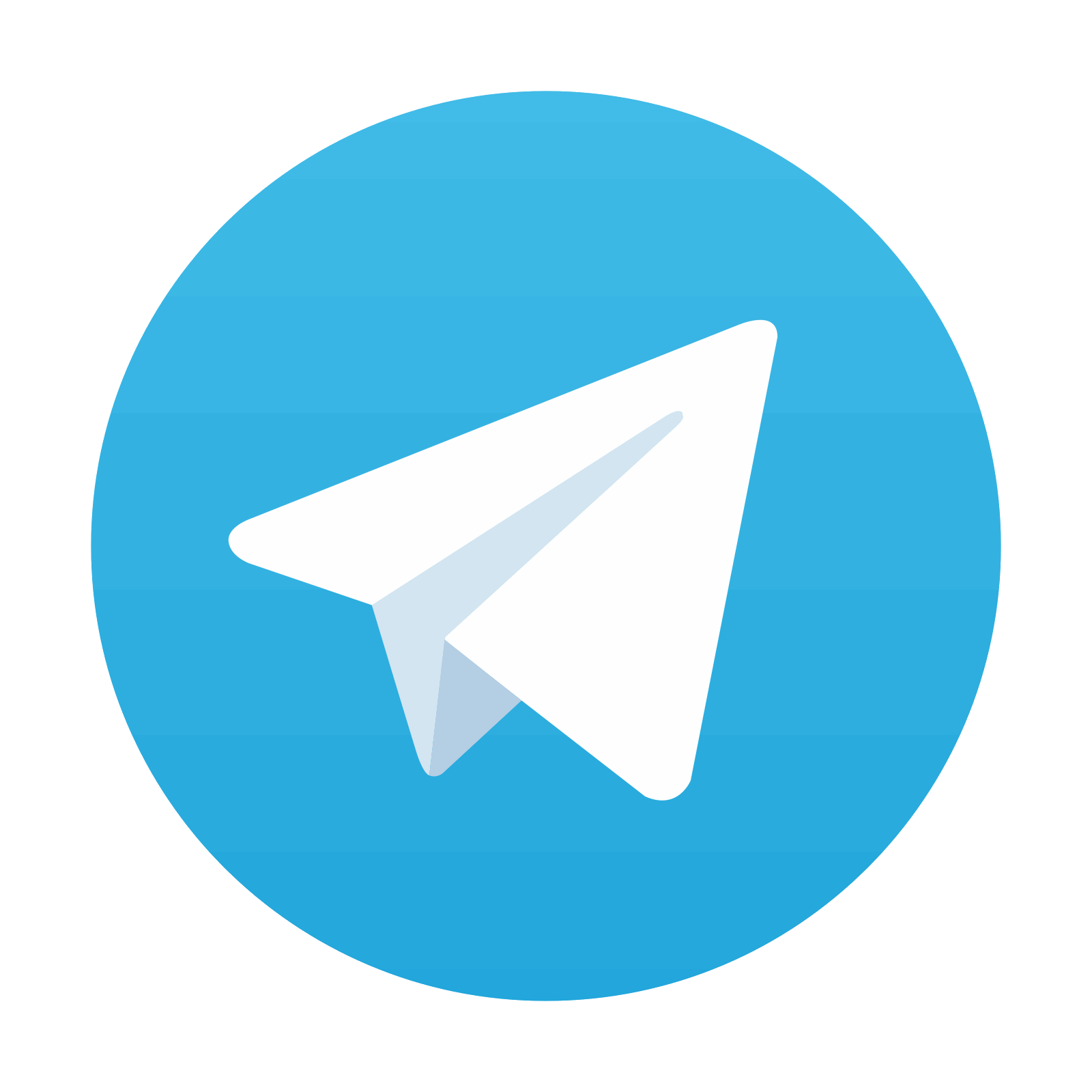
Stay updated, free dental videos. Join our Telegram channel

VIDEdental - Online dental courses
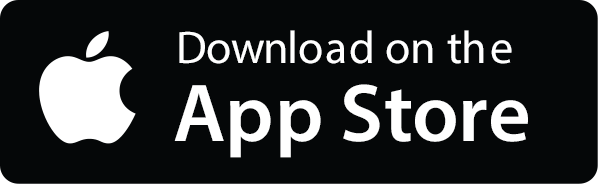
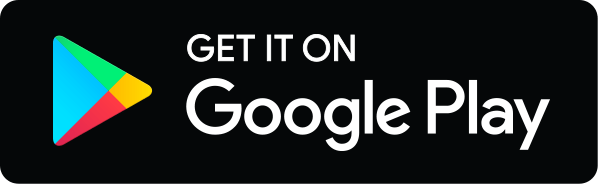
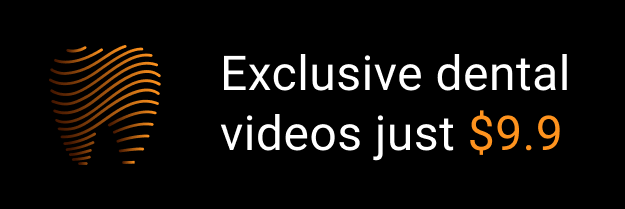