Abstract
Objectives
The purposes of this study were to investigate the effects of thermal residual stresses on the reliability and lifetime of zirconia–veneer crowns.
Methods
One hundred and twenty eight second upper premolar zirconia–veneer crowns were manufactured for testing the initial strength ( n = 64) and under cyclic fatigue ( n = 64). Zirconia copings (YZ Cubes, VITA Zahnfabrik, CTE: alphac = 10.5 ppm/°C) were milled using a Cerec3 InLab (Sirona) machine and sintered to a final thickness of 0.7 mm. Sixty-four copings were sandblasted with 105 μm alumina particles (15 s, 3 cm distance, 45° angle, 0.4 MPa pressure) in order to trigger a tetragonalmonoclinic transformation and to produce a rough surface. The copings were veneered using two different porcelains (VM9, VITA Zahnfabrik, CTE: alphaVM9 = 9.1 ppm/°C, Lava Ceram, 3M ESPE, CTE: alphaLava = 10.2 ppm/°C) so to result in crowns with either high thermal mismatch (+1.4 ppm/°C with VM9) and low thermal mismatch (+0.3 ppm/°C with Lava Ceram). The porcelains were applied by the same operator and fired (VITA Vacumat 4000) according to the firing schedules defined by the manufacturers to a final thickness of 1.4 mm (total crown thickness = 2.1 mm, core/veneer ratio = 0.5). After the last glaze firing the crowns were cooled following a fast (600 °C/min) or a slow (30 °C/min) cooling protocol. The glazed crowns were submitted to a sliding-motion (0.7 mm lateral movement) cyclic fatigue in a chewing simulator (SD Mechatronik) under 20 kg (∼200 N load) weight until failure (chipping) ( n = 16). The other half of the crowns were subjected to a compressive loading test in an universal testing machine (Instron model 4240) until failure at a cross-head speed of 0.75 mm/min ( n = 16). The failure probability for initial strength and cyclic fatigue was performed using a Weibull distribution approach at a scale factor of n = 16.
Results
The compressive strength test showed a low sensitivity to detect reliability variations regarding thermal stresses created within the veneer layer of tested crowns. For cyclic fatigue, slow cooling resulted in statistically higher cycles to failure only for the crowns that presented a high thermal mismatch between core and veneer (VM9 group). Comparisons between veneers with high or low thermal mismatches showed statistically higher sigma0 for Lava Ceram-veneered crowns only when the groups were fast-cooled.
Significance
Minimizing the thermal residual stresses within the veneer through the use of a veneer with a closer CTE to the zirconia delays the failure of zirconia–veneer crowns. Slow-cooling increases the lifetime of crowns presenting large differences in CTE between the zirconia core and the veneering porcelain.
1
Introduction
Yttria-stabilized Tetragonal Zirconia Polycrystals (Y-TZP) used as infrastructures for crowns, bridges, and long-span fixed partial dentures (FPDs) rarely fracture under function, provided that basic mechanical principles are respected . At least that is the current state of affairs regarding the clinical performance of zirconia as a porcelain-veneered high-strength core ceramic according to the available prospective clinical trials . Unfortunately, due to the short history of zirconia as a dental ceramic, literature supplies little clinical performance data longer than 5 years of function.
To balance this shortage in clinical predictability, in vitro studies supply forecast data on failure/survival probability through fatigue experiments. Results on parameters for subcritical crack growth under static loading for soft-machined and HIPed Y-TZP have shown that the greatest loss in strength occuring due to stress corrosion cracking happens within the first year and nearly stabilizes between 5 and 10 years . Under dynamic fatigue, lifetimes of porcelain-veneered zirconia bridges have been estimated to surpass 20 years of function . Compared to other reinforced ceramics used as infrastructures, zirconia outranks all in clinical survivability , and has been shown to match metal alloys as a framework regarding mechanical stability at equivalent evaluation periods .
The reassurance on the long-term structural integrity of zirconia as infrastructure provided by clinical and in vitro results deviated the attention of clinicians and researchers to the high incidence of veneer chipping reported in some clinical trials. High incidence rates of veneer chipping in zirconia-based FPDs have been reported to range from 15% to 36% in 5 years, 25% in 2.5 years to 54% in 1 year. In a recent 10-year evaluation period of zirconia FPDs, a complication rate of 32% was recorded due to veneer chippings . Fracture within the veneer in reinforced bilayer systems is expected to occur since cracks approaching a high-modulus, high-toughness infrastructure tend to stop or deflect instead of passing through . This behavior is observed for zirconia-based as for metal–ceramic systems. Nonetheless, the unusual high incidence of chipping in zirconia-based FPDs suggests an escalation of the usual factors involved in the veneer fracture phenomenon in bilayer systems. A randomized controlled clinical trial comparing the performances of zirconia-based and metal–ceramic FPDs has recently shown that the incidence rate of minor chippings were similar for both systems, whereas unacceptable chippings occured only for a zirconia-based system in 8.4% of the cases after 3 years . Such observation has shown consistency throughout clinical studies .
First analytical models and experimental data on metal–ceramic bilayers have identified stress gradient developments within the veneering porcelain as the main factor governing veneer fracture occurance . Upon cooling from sintering temperature, porcelain and metal contract to the final dimensions as porcelain transforms from the viscoelastic state to the solid state at the glass transition temperature ( T g ). Downwards through the T g range, elastic and viscoelastic strain take place but structural relaxation of the glass still occurs and contraction stresses can be dissipated through molecular rearrangement . Below T g the glass becomes a solid and all structural contraction is reverted into residual stress locked inside the porcelain layer. A temperature gradient builds-up within the porcelain due to its inhomogeneous cooling. In a pure porcelain bar, the surfaces cool first as the interior remains hot, forming a parabolic temperature profile at fast cooling rates . Mismatch in the coefficient of thermal expansion (CTE) between core and veneer adds further stress in the veneer as consequence of the contraction strain difference between materials. Since perfect matching is unlikely to happen, a slightly positive mismatch ( α core − α veneer = +Δ α ppm/°C) is recommended in order to keep the surface under compression and avoid crack nucleation at tensile stresses .
Due to the universality of thermodynamic laws, the interaction of different ceramic components in reinforced all-ceramic prostheses also creates thermal stresses upon cooling. As far as zirconia–porcelain bilayers are concerned, Swain has shown an increase in temperature difference between the core and the veneer due to the low thermal diffusivity of zirconia in comparison to other core materials. During the pure elastic strain range under T g , the zirconia core keeps the internal portion of the porcelain under higher temperatures for longer periods, resulting in high residual stresses, especially for thick porcelain layers. By varying the cooling rate from sintering temperature, T g of the veneer is dislocated and time and kinetics for structural relaxation change . By allowing the prostheses to cool slowly inside the oven until the temperature drops below T g (usually between 600 and 550 °C for dental porcelains), the temperature differences between the veneer surface and the veneer interior are dramatically reduced . The veneer then solidifies more homogeneously throughout its thickness, and the resultant residual stress development becomes contingent on the thermal mismatch difference between core and veneer alone .
In view of the white appearance of fractured surfaces in zirconia-based prostheses, many suggested that a core/veneer delamination was taking place rather than sole chipping, implying an issue involving the interfacial bonding between zirconia and porcelain. Some authors proposed sandblasting the zirconia surface prior to veneering as a strategy to improve interfacial quality . Interestingly, optimal interfacial bond strengths have been shown using different porcelains on a zirconia substrate . Others have expressed their concerns about the interfacial bond stability of veneered transformed ( t → m ) zirconia surfaces, whether triggered by the wet veneering slurry or by the impact of alumina particles upon sandblasting . Since evidences show that not all transformed monoclinic grains transform back to the tetragonal phase after the subsequent veneer firings , the possible effect on the interfacial bond of remanescent monoclinic phase present at the interface has been put to question, but unaddressed yet.
The experimental evidences produced in the last few years to clarify the chipping phenomenon in Y-TZP–veneer systems have been valuable, but their designs fall short on clinically relevant aspects, such as the reliability of complex crown-like geometries under cyclic fatigue. Therefore, the purposes of this study are: (1) to evaluate how thermal residual stresses affect the reliability of Y-TZP–porcelain crowns under fatigue and static testing conditions and (2) to compare the reliability results of both test configurations. The present paper evaluates the mechanical response of sandblasted and as-sintered zirconia copings veneered with porcelains of different CTEs and cooling rates. The crowns were tested under compression and under sliding motion cyclic fatigue to simulate a clinical loading scenario.
2
Materials and methods
One hundred and twenty eight zirconia–veneer crowns were fabricated according to two different procedures of coping surface treatment, veneered with two different porcelains and cooled following a fast or a slow cooling protocol. Half of the crowns of each group were assigned either to an initial compression test or to a cyclic fatigue experiment until failure.
2.1
Copings fabrication, surface treatment and veneering process
An intact human second maxillary premolar was prepared for a full coverage all-ceramic crown. With diamond burs, the tooth was reduced at the occlusal, free surfaces (palatal and buccal) and proximal surfaces in 2.1, 1.7, and 1.2 mm, respectively. The margins were chamfered with a circumferential depth of 1 mm. During preparation, sectioned silicone indices were used to control the reduction depth. The final polished preparation was scanned and a coping was designed for further milling (Cerec3 Inlab, Sirona, Germany). Yttrium-stabilized tetragonal zirconia blanks (YZ Cubes, VITA Zahnfabrik, Bad Säckingen, Germany, Lot 30820, CTE: α Y-TZP = 10.5 ppm/°C) were milled using a Cerec3 Inlab machine and sintered at a final temperature of 1530 °C for 2 h to produce copings of 0.7 mm thickness. Sixty-four of the 128 copings were sandblasted with 105 μm Al 2 O 3 particles with 0.4 MPa pressure at a distance of 3 cm and 45° angle for 15 s in order to roughen the surface and to trigger a t → m transformation to produce a surface rich in monoclinic phase. The other 64 copings remained as-sintered.
The copings were veneered using two different porcelains (VM9, VITA Zahnfabrik, CTE: α VM9 = 9.1 ppm/°C; Lava Ceram 3M ESPE, CTE: α lava = 10.2 ppm/°C) so to create with the zirconia core a high or a low thermal mismatch (Δ α = +1.4 ppm/°C with VM9 and Δ α = +0.3 ppm/°C with Lava Ceram). A summary of the thermal, physical and mechanical properties of the core ceramic and the porcelains used are listed in Table 1 . The porcelains were applied by a single operator according to the manufacturer’s instructions using a dentin and an enamel shade (D3M2 and EL1 for VM9, lot 19469; DA3 and EA1 for Lava Ceram, lot 8790D) as done routinely in practice. After the enamel firing, the thickness of the crowns were checked with a specimeter and grinded with a low-speed superfine diamond bur under water lubrication down to 2.1 mm (cusp) and 1.7 mm (buccal surface) if necessary. At the buccal cusp the core/veneer ratio was 0.5.
The final firing was a glaze firing (without glaze) as described in Table 2 . At the end of the glaze firing the crowns were cooled from final sintering temperature following a fast or a slow cooling program. Fast cooling consisted of the immediate opening of the oven’s door (Vacumat 4000, VITA Zahnfabrik) after the end of the firing schedule, exposing the crowns to contact with convective ambient air (∼23 °C). After the end of the firing schedule in the slow cooling program, the door of the oven opened only 10%, opening completely as soon as the temperature inside the oven reached 200 °C. A K-type thermocouple was used to determine the cooling rates according to the fast or slow cooling programs at the same position inside the oven the crowns were sintered. Between the maximum sintering temperature and 500 °C, cooling rates of 600 °C/min and 30 °C/min were recorded for fast and slow cooling protocols, respectively. Eight crowns from each subgroup were fired together during the last firing and designated either to the compressive test or to the cyclic fatigue experiment.
Porcelain | Start temp. (°C) | Heating rate (°C/min) | Final temp. (°C) | Holding time (min) | Cooling |
---|---|---|---|---|---|
VM9 | 500 | 80 | 900 | 1 | Fast or slow |
Lava Ceram | 480 | 45 | 820 | 1 | Fast or slow |
2.2
Compression test and cyclic fatigue test
Two types of abutment were produced to support the crowns and to fix them in position in the appropriate testing devices. For the compression test the crowns were luted over a bronze alloy abutment made from impressions of the original preparation. For the fatigue testing, resin composite (Grandio, VOCO, Germany) abutments were fabricated and stored in distilled water for 30 days prior to the cementation of the crowns with a glass ionomer cement (Fuji Cem, GC, Japan). After luting, the specimens were stored in distilled water for 1 week prior to testing.
For the compression test, the abutments were mounted in a metal plate fixed to the lower axis of a universal testing machine (Instron Model 4240). A 1 mm-thick rubber band was used between the crown and the flat surface of the upper axis. The crowns were loaded at the buccal cusp using a 5 kN load cell at a 0.75 mm/min cross-head speed until fracture.
The crowns subjected to cyclic fatigue were mounted in an eight-chamber dual-axis chewing simulator (SD Mechatronik GmbH, Feldkirchen, Germany). An evaluation of the reproducibility of contact forces and force profiles of this chewing simulator can be found elsewhere . Twenty kilogram weights were mounted on vertical bars (independent for each test chamber) driven by computer controlled step motors. Since the maximum load operated by this machine is 80 kg, only 4 chambers were used simultaneously. Steatite balls ( = 6.25 mm) were used as antagonist and changed for every new tested crown. The antagonist loading was composed by a three-step motion, starting with a 1 cm descendent movement at 20 mm/s until the steatite ball touched the buccal cusp of the crown. After the contact, the crown was loaded (so no impact occured) and the antagonist was moved 0.7 mm in direction to the occlusal sulcus at 20 mm/s to simulate the sliding path of teeth during function. The last part of the cycle was a direct return movement of the antagonist to the initial position also at 20 mm/s. The final cycling frequency was 1.5 Hz. A schematic of the loading cycle is shown in Fig. 1 . During testing the crowns were immersed in distilled water at room temperature. Since small chippings at the edges of the wear facets usually developed at low cycling for all specimens, a pilot study was used to determine the criteria for defining the failure by chipping. Chipping was defined as the loss of veneer structure greater than 20% of the area of the buccal surface of the crown. The crowns under test were inspected visually for chippings every ∼2500 cycles by drying with air spray after removing the water from the chamber.

2.3
Determination of Weibull parameters
An uniform distribution of material defects results in a strength variability which can be statistically treated using the Weibull approach, describing the failure probability P f .
P f = 1 − exp − σ σ 0 m
where σ 0 is the characteristic strength at a failure probability of 63.2% ( P f = 63.2%) and m is the Weibull modulus, respectively. The strength data was evaluated according to these two parameter cumulative Weibull distribution by plotting the failure probability P f versus fracture strength σ c
ln 1 ( 1 − P f ( σ ) ) = m ⋅ ln σ − m ⋅ ln σ 0
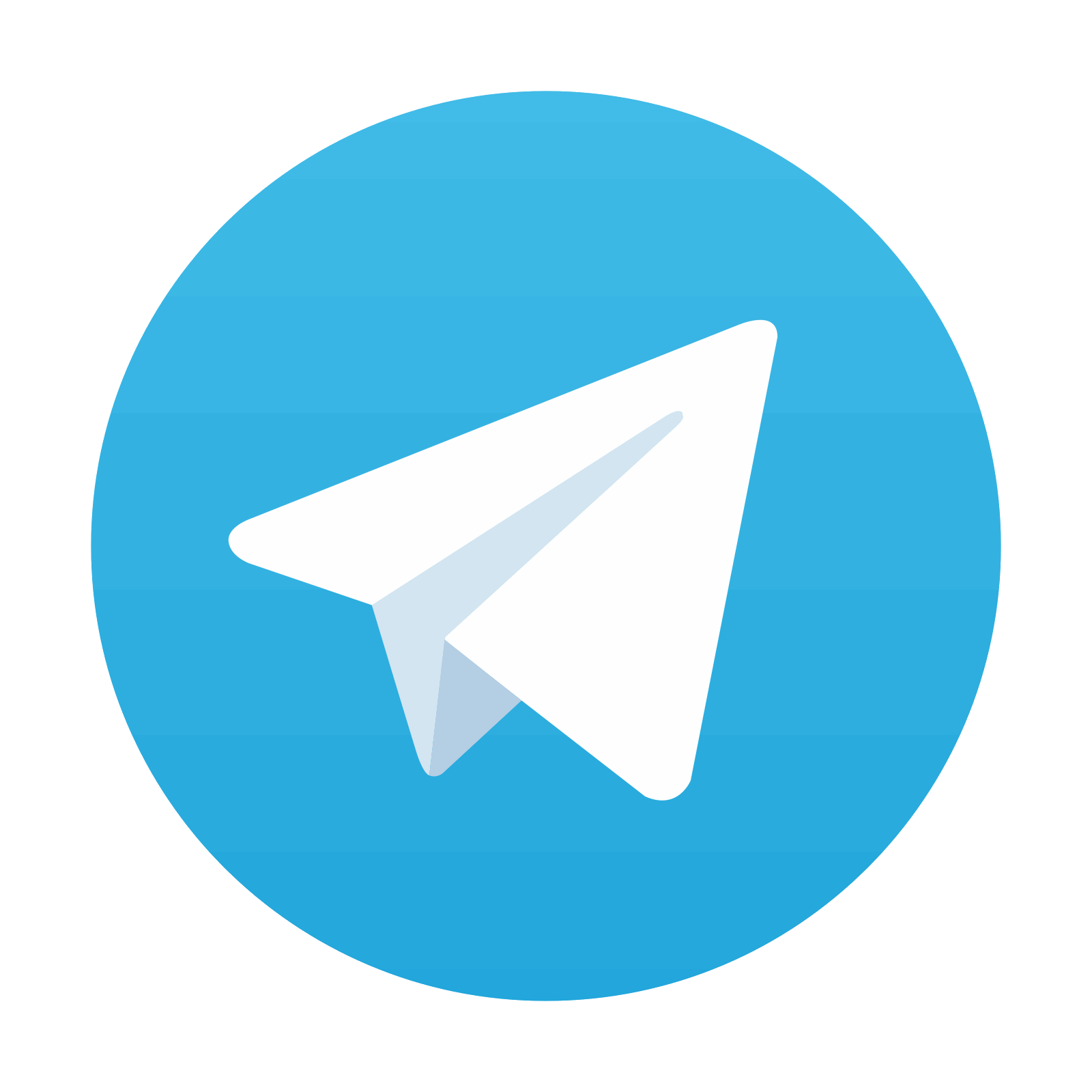
Stay updated, free dental videos. Join our Telegram channel

VIDEdental - Online dental courses
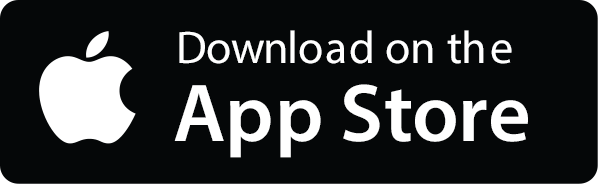
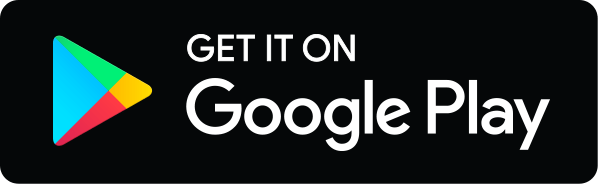