Abstract
Objectives
To evaluate the effect of addition of copper nanoparticles at different concentrations into an etch-and-rinse adhesive (ER) on antimicrobial activity, Knoop microhardness (KHN), in vitro and in situ degree of conversion (DC), as well as the immediate (IM) and 2-year (2Y) resin-dentine bond strength (μTBS) and nanoleakage (NL).
Methods
Seven experimental ER adhesives were formulated according to the amount of copper nanoparticles incorporated into the adhesives (0 [control], 0.0075 to 1 wt.%). We tested the antimicrobial activity of adhesives against Streptococcus mutans using agar diffusion assay after IM and 2Y. The Knoop microhardness and in vitro DC were tested after IM and 2Y. The adhesives were applied to flat occlusal dentine surfaces after acid etching. After resin build-ups, specimens were longitudinally sectioned to obtain beam-like resin-dentine specimens (0.8 mm 2 ), which were used for evaluation of μTBS and nanoleakage at the IM and 2Y periods. In situ DC was evaluated at the IM period in these beam-like specimens. Data were submitted to appropriate statistical analyses (α = 0.05).
Results
The addition of copper nanoparticles provided antimicrobial activity to the adhesives only in the IM evaluation and slightly reduced the KHN, the in vitro and in situ DC (copper concentrations of 1 wt.%). However, KHN increase for all concentrations after 2Y. After 2Y, no significant reductions of μTBS (0.06 to 1% wt.%) and increases of nanoleakage were observed for copper containing adhesives compared to the control group.
Conclusion
Copper nanoparticles addition up to 0.5 wt.% may provide antimicrobial properties to ER adhesives and prevent the degradation of the adhesive interface, without reducing the mechanical properties of the formulations.
1
Introduction
Failure of composite restorations by secondary caries is considered the major reason for restoration replacement, which results in millions of dental care dollars spent annually . The higher accumulation of dental biofilm around composite resin restorations than other materials or intact enamel are likely responsible for the faster degradation of these restorations . Furthermore, composite resin undergoes volumetric contraction during polymerization, which have a great impact on their sealing ability. This can result in the formation of gaps between the adhesive resin and the dental structure .
In the oral environment, water, enzymes, occlusal stresses (generated during mastication and especially during parafunctional activities), temperature and microorganisms derived from plaque biofilms challenge the resin-dentine interface . S. mutans and other bacterial species can then bond to this suitable biofilm and, in the presence of gaps and weakened interface, may invade the inner area of teeth and allow for the development of caries adjacent to tooth-restorative margins . There seems to be an association between failure of the resin-dentine interface and increased levels of cariogenic bacteria at the perimeter of resin composite restorations . Production of acids and esterases may also accelerate the degradation of the hybrid layer, being an important factor in the premature failure of moderate-to-large composite restorations .
Although biofilm cannot be eliminated , one can reduce the pathogenicity of biofilm and make the restorative interface less prone to caries adjacent to restorations . This can be achieved by developing dental materials with antibacterial properties to reduce biofilm formation at the tooth-restoration margins, without compromise the mechanical properties of the adhesive formation .
Among the most promising agents with antibacterial properties are metallic nanoparticles, which exhibit increased chemical activity and biocide effectiveness . The antibacterial activity of copper and silver nanoparticles has been extensively investigated. Copper nanoparticles showed higher antibacterial activity compared with silver nanoparticles against Escherichia coli, Bacillus subtilis and Staphylococcus aureus . In addition to superior antimicrobial activity, copper is cheaper than silver, easily available and the synthesis of copper nanoparticles is cost effective . An additional advantage of copper nanoparticles is that they are easily oxidizable in air or aqueous media producing copper oxide nanoparticles. Copper oxide has an effective bactericidal action and can be easily blended with polymers or macromolecules producing stable polymers in regards to chemical and physical properties .
In dentistry, silver nanoparticles incorporation in resin cement and dental adhesives showed high antimicrobial activity and also improved mechanical and adhesive properties . Incorporation of copper was also evaluated in several dental materials, such as glass ionomer , dental adhesive and even dental implant . In dental adhesives, only two recent studies used copper nanoparticles to test the effectiveness of these materials against S. mutans , However, none of these studies evaluated the mechanical properties of the adhesive, and hence the effect of different copper concentrations on them. In terms of bond strength, only Sabatini et al. evaluated the immediate strength of the adhesive interface, and no study so far addressed the effects of copper addition on the long-term resin-dentine interface. Additionally, the literature still lacks information about the optimal copper concentration that can be added to an adhesive system to express its antimicrobial activity without jeopardizing other mechanical properties.
Therefore, we designed this in vitro study to investigate the effect of addition of copper nanoparticles at different concentrations into a simplified etch-and-rinse adhesive system on the antimicrobial activity, Knoop microhardness, in vitro and in situ degree of conversion, as well as the immediate and 2-year resin-dentine bond strength and nanoleakage.
2
Materials and methods
2.1
Formulation of the experimental adhesives and characterization of the copper nano-powder by FE-SEM and EDX
We formulated experimental adhesives using the simplified etch-and-rinse adhesive system (Ambar [AM], FGM Prod. Odont. Ltda, Joinville, SC, Brazil). All adhesives were formulated by adding different concentrations of copper nanoparticles (99.9% pure, SkySpring Nanomaterials, Inc., Houston, TX, USA; www.ssnano.com ) (wt%): 0% (control, commercial material), 0.0075%, 0.015%, 0.06%, 0.1%, 0.5% and 1.0%.
The copper nanoparticles used for production of the experimental adhesives were characterized by field emission scanning electron microscope (FE-SEM) and energy dispersive X-ray (EDX) analysis. The incorporation to the adhesive solution was done in a dark room with a motorized stirrer.
2.2
Antimicrobial activity
After isolating a metallic matrix (5.8 mm diameter, 1.0 mm thick) with a very thin layer of petroleum jelly, we dispensed the adhesive until completely fill the mold. All visible air bubbles trapped in the adhesives specimens were carefully removed with a microbrush (Microbrush International, Grafton, WI, USA). An air stream was applied for solvent evaporation for 40 s at a distance of 10 cm.
Under a plastic matrix strip, the adhesive specimens were light-cured for 40 s with a LED light source at 1200 mW/cm 2 (Radii-cal, SDI, Baywater, Victoria, Australia), in close contact with each disc-like specimen. After polymerization, the specimens were removed from the mold, and polished with 600-grit SiC paper in order to remove the adhesive excesses and the oxygen-inhibition layer. Five adhesive discs of each group were made.
All specimens were stored in a dark vial for 24 h and each side of the specimen was disinfected by ultraviolet light with a 30 min exposure. They were placed on brain heart infusion (BHI) agar plates (Kasvi Ltda, Curitiba, PR, Brasil) containing a freshly prepared lawn of S. mutans (ATCC 25175). S. mutans was cultured microaerophilically in brain heart infusion broth (Difco Laboratories, Detroit, MI, USA) for 72 h at 37 °C. Then, 100 μL of the bacterial suspension was swabbed onto BHI to create the lawn and the BHI agar plates were stored in an anaerobic jar. The inhibition zones (mm) were measured after 96 h with a digital caliper to the nearest 0.1 mm (Absolute Digimatic, Mitutoyo, Tokyo, Japan).
2.3
Knoop microhardness
Five adhesive discs of each group were produced as described for microbiological test. After preparation, specimens were stored in a dark vial for 24 h or after 2 year of water storage before microhardness measurement. Specimens were then taken to a HMV-2 microhardness tester (Shimadzu; Tokyo, Japan) equipped with a Knoop indenter. Five measurements were performed of each specimen with a load of 10 g for 15 s. The first measurement was performed in the center of the adhesive disc. The other four measurements were performed 100 μm and 200 μm to the left and right of the first one. Values obtained for the same specimen were averaged for statistical purposes .
2.4
In vitro degree of conversion
Ten new adhesive discs of each group were produced as described for microbiological tests. Specimens were stored in wet environment for 24 h at 37 °C prior to performing the DC readings. The DC measurements were performed in a micro-Raman spectrometer (Bruker Optik GmbH, Ettlingen, Baden-Württemberg, Germany).
The micro-Raman spectrometer was first calibrated for zero and then for coefficient values using a silicon specimen. Specimens were analyzed using the following micro-Raman parameters: 20-mW Neon laser with 532-nm wavelength, spatial resolution of ≈3 μm, spectral resolution ≈5 cm −1 , accumulation time of 20 s with 6 co-additions, and magnification of 20X (Olympus UK, London, UK) to beam diameter of ≈1 μm . The spectra were taken at three different sites for each specimen and the values averaged for statistical purposes. Spectra of uncured adhesives were taken as reference. Post-processing of spectra was performed using the dedicated Opus Spectroscopy Software version 6.5 (Bruker Optik GmbH, Ettlingen, Baden- Württemberg, Germany).
The ratio of double-bond content of monomer to polymer in the adhesive was calculated according to the following formula: DC (%) = (1–R cured /R uncured ) x 100, where R is the ratio of aliphatic and aromatic peak areas at 1639 cm −1 and 1609 cm −1 in cured and uncured adhesives.
2.5
Teeth preparation and bonding procedures
The University Ethics Committee approved this part of the experiment under protocol number 1.065.446. Thirty-five caries-free extracted human third molars, collected from patients with age ranging from 18 to 35 years old, were randomly distributed among 7 groups by lottery. The teeth were collected after the patient’s informed consent. Teeth were disinfected in 0.5% chloramine, stored in distilled water and used within 3 months after extraction. A flat dentine surface was exposed on each tooth after wet grinding the occlusal enamel with 180-grit SiC paper. The enamel-free, exposed dentine surfaces were further polished with 600-grit silicon-carbide paper for 60 s to standardize the smear layer.
The adhesives were applied as per manufacturers’ instructions. The dentine surfaces were acid etched with 37% phosphoric acid for 15 s, water rinsed for 15 s and dried with absorbent paper keeping the dentine surface slightly wet. Two adhesive coats were vigorously applied by rubbing the adhesive for 20 s (10 s each coat). Then, gently air stream of 10 s was applied for solvent evaporation and the adhesive layer was light cured with a LED light curing unit for 10 s at 1200 W/cm 2 (Radii-cal, SDI, Bayswater, Victoria, Australia). Resin composite blocks (Opallis, FGM) were buildup on the bonded surfaces in 3 increments of 1.0 mm thick each and each one was individually light activated for 40 s. A single operator carried out all bonding procedures in an environment with controlled temperature and humidity. Five teeth were used for each experimental group.
After storage of the bonded teeth in distilled water at 37 °C for 24 h, they were longitudinally sectioned in both “x” and “y” directions across the bonded interface with a diamond saw in a cutting machine (IsoMet 1000; Buehler, Lake Bluff, USA), under water cooling at 300 rpm to obtain resin-dentine beam-like specimens with a cross-sectional area of approximately 0.8 mm 2 .
The number of premature failures (PF) per tooth during specimen preparation was recorded. The cross-sectional area of each beam-like specimen was measured with the digital caliper to the nearest 0.01 mm and recorded for subsequent calculation of the microtensile bond strength (μTBS) values (Absolute Digimatic, Mitutoyo, Tokyo, Japan). The resin-dentine beam-like specimens originated from the same tooth were randomly divided and assigned to be tested immediately or after 2-year of storage in distilled water at 37 °C. The storage solution was not changed and its pH was monitored monthly. The resin-dentine beam-like specimens from each tooth were then divided as follow:
- •
Two beam-like specimens at each storage period were used for nanoleakage evaluation.
- •
Two beam-like specimens were used to measure the immediate in situ degree of conversion.
- •
Two beam-like specimens at each storage period were used for identification of the presence of copper in the hybrid layer.
- •
The remaining beam-like specimens were submitted to μTBS test at each storage period.
2.6
Microtensile bond strength testing
Each beam-like specimens was attached to a modified device for microtensile bond strength test with cyanoacrylate resin (Super Bonder, Loctite, São Paulo, SP, Brazil) and subjected to a tensile force in a universal testing machine (Kratos, São Paulo, SP, Brazil) at 0.5 mm/min. The failure mode was evaluated at 40x (HMV-2, Shimadzu, Tokyo, Japan) and classified as cohesive in dentine (failure exclusive within cohesive dentine − CD); cohesive in resin (failure exclusive within cohesive resin − CR); adhesive (failure at resin/dentine interface − A), or mixed (failure at resin/dentine interface that included cohesive failure of the neighboring substrates, M). The number of premature failures (PF) was recorded and it was not included in the average mean bond strength.
2.7
Nanoleakage evaluation
All resin-dentine beam-like specimens selected for this test were coated with two layers of nail varnish applied up to within 1 mm of the bonded interfaces. The resin-dentine beam-like specimens were immersed in 50 wt% ammoniacal silver nitrate solution in total darkness for 24 h, rinsed thoroughly in distilled water, and immersed in photo developing solution for 8 h under a fluorescent light to reduce silver ions into metallic silver grains within voids along the bonded interface.
Specimens were mounted on aluminium stubs, polished with 1000-, 1500-, 2000- and 2500-grit SiC paper and 1 and 0.25 μm diamond paste (Buehler Ltd., Lake Bluff, IL, USA). Then, they were ultrasonically cleaned, air dried and gold sputter coated (MED 010, Balzers Union, Balzers, Liechtenstein). The interfaces were observed in a scanning electron microscope in the backscattered mode at 15 kV (VEGA 3 TESCAN, Shimadzu, Tokyo, Japan).
In a way to standardize image acquisition, three pictures were taken of each beam-like specimen. The first picture was taken in the center of the resin-dentine beam-like specimens. The other two pictures were taken 0.3 mm to the left and right of the first one. As two resin-dentine beam-like specimens per tooth were evaluated and a total of five teeth were used for each experimental condition, a total of 30 images were evaluated per group . A technician who was blinded to the experimental conditions under evaluation took them all. The relative percentage of nanoleakage within the adhesive and hybrid layer areas was measured in all pictures using the public domain Image J software, a Java-based image processing software package developed at the National Institutes of Health (NIH) .
Before performing the nanoleakage test a pilot test was conducted to evaluate if the presence of copper in the adhesive could impair the visualization of silver nitrate uptake. For this purpose, we performed scanning electron microscopy (SEM) images of resin-dentine interfaces of all groups without immersion in silver nitrate. Even in adhesive interfaces with the highest copper concentration (1%), copper nanoparticles were not observed using the same parameters described above. And thus, the results of nanoleakage test reflect the amount of silver uptake into unpolymerized areas and/or nanospaces not infiltrated by the resin adhesive but not the presence of copper in the hybrid layer.
2.8
In situ degree of conversion within adhesive/hybrid layers
All resin-dentine beams selected for this test were wet polished using 1500; 2000; 2500 and 4000-grit SiC paper for 30 s each. The specimens were ultrasonically cleaned for 10 min and positioned into micro-Raman equipment. The DC measurements were performed in a micro-Raman spectrometer (Bruker Optik GmbH, Ettlingen, Baden-Württemberg, Germany). The micro-Raman spectrometer was first calibrated for zero and then for coefficient values using a silicon specimen. Specimens were analyzed using the following micro-Raman parameters: 20-mW Neon laser with 532-nm wavelength, spatial resolution of ≈3 μm, spectral resolution ≈5 cm −1 , accumulation time of 30 s with 6 co-additions, and magnification of 100X (Olympus UK, London, UK) to beam diameter of ≈1 μm . The spectra were taken at the resin-dentine interface, in the middle of the hybrid layer within the intertubular dentine, at three different sites for each specimen and the values averaged for statistical purposes. Spectra of uncured adhesives were taken as reference. Post-processing of spectra was performed using the dedicated Opus Spectroscopy Software version 6.5 (Bruker Optik GmbH, Ettlingen, Baden-Württemberg, Germany). The ratio of double-bond content of monomer to polymer in the adhesive was calculated according to the formula described earlier in the materials and methods section in the item in vitro degree of conversion.
2.9
Identification of copper within adhesive itself and in the adhesive/hybrid layers by EDX
For the first analysis, three adhesive discs of each group were produced as described for the microbiological test. After preparation, specimens were stored in a dark vial for 24 h and vertically sectioned in the middle with a diamond saw in a cutting machine (IsoMet 1000; Buehler, Lake Bluff, USA), under water cooling (at 300 rpm). Then, 05 areas of this cross-sectional interface were evaluated under EDX.
In the second part, two beam-like specimens at each storage period previously obtained during specimens sectioning (item 2.5) were used for identification of the presence of copper in the hybrid layer. The analysis of the copper content in each selected beam was performed by field emission scanning electron microscope (FE-SEM) (MIRA 3 TESCAN, Shimadzu, Tokyo, Japan) coupled with an energy-dispersive X-ray spectrometer (EDX). The bonding area was observed and the analysis was focused to the middle of the hybrid layer. One site, randomly determined, per beam-like specimens was examined.
2.10
Statistical analysis
Data for microbiological test (mm), in vitro degree of conversion and in situ degree of conversion (%) were subjected to a one-way ANOVA. Data for microhardness (KNH) was subjected to a two-way ANOVA. For μTBS and nanoleakage, the experimental unit was the tooth with half being tested immediately and the other half after 2 years of water storage. The μTBS and nanoleakage values of all beam-like specimens from the same hemi-tooth were averaged for statistical purposes. The μTBS (MPa) and nanoleakage (%) data of each adhesive were subjected to two-way repeated measures ANOVA. The repeated measure was the tooth at the two-evaluation period. Tukey’s post hoc test was used for pair-wise comparisons (α = 0.05) using the Statistica for Windows software (StatSoft, Tulsa, OK, USA).
2
Materials and methods
2.1
Formulation of the experimental adhesives and characterization of the copper nano-powder by FE-SEM and EDX
We formulated experimental adhesives using the simplified etch-and-rinse adhesive system (Ambar [AM], FGM Prod. Odont. Ltda, Joinville, SC, Brazil). All adhesives were formulated by adding different concentrations of copper nanoparticles (99.9% pure, SkySpring Nanomaterials, Inc., Houston, TX, USA; www.ssnano.com ) (wt%): 0% (control, commercial material), 0.0075%, 0.015%, 0.06%, 0.1%, 0.5% and 1.0%.
The copper nanoparticles used for production of the experimental adhesives were characterized by field emission scanning electron microscope (FE-SEM) and energy dispersive X-ray (EDX) analysis. The incorporation to the adhesive solution was done in a dark room with a motorized stirrer.
2.2
Antimicrobial activity
After isolating a metallic matrix (5.8 mm diameter, 1.0 mm thick) with a very thin layer of petroleum jelly, we dispensed the adhesive until completely fill the mold. All visible air bubbles trapped in the adhesives specimens were carefully removed with a microbrush (Microbrush International, Grafton, WI, USA). An air stream was applied for solvent evaporation for 40 s at a distance of 10 cm.
Under a plastic matrix strip, the adhesive specimens were light-cured for 40 s with a LED light source at 1200 mW/cm 2 (Radii-cal, SDI, Baywater, Victoria, Australia), in close contact with each disc-like specimen. After polymerization, the specimens were removed from the mold, and polished with 600-grit SiC paper in order to remove the adhesive excesses and the oxygen-inhibition layer. Five adhesive discs of each group were made.
All specimens were stored in a dark vial for 24 h and each side of the specimen was disinfected by ultraviolet light with a 30 min exposure. They were placed on brain heart infusion (BHI) agar plates (Kasvi Ltda, Curitiba, PR, Brasil) containing a freshly prepared lawn of S. mutans (ATCC 25175). S. mutans was cultured microaerophilically in brain heart infusion broth (Difco Laboratories, Detroit, MI, USA) for 72 h at 37 °C. Then, 100 μL of the bacterial suspension was swabbed onto BHI to create the lawn and the BHI agar plates were stored in an anaerobic jar. The inhibition zones (mm) were measured after 96 h with a digital caliper to the nearest 0.1 mm (Absolute Digimatic, Mitutoyo, Tokyo, Japan).
2.3
Knoop microhardness
Five adhesive discs of each group were produced as described for microbiological test. After preparation, specimens were stored in a dark vial for 24 h or after 2 year of water storage before microhardness measurement. Specimens were then taken to a HMV-2 microhardness tester (Shimadzu; Tokyo, Japan) equipped with a Knoop indenter. Five measurements were performed of each specimen with a load of 10 g for 15 s. The first measurement was performed in the center of the adhesive disc. The other four measurements were performed 100 μm and 200 μm to the left and right of the first one. Values obtained for the same specimen were averaged for statistical purposes .
2.4
In vitro degree of conversion
Ten new adhesive discs of each group were produced as described for microbiological tests. Specimens were stored in wet environment for 24 h at 37 °C prior to performing the DC readings. The DC measurements were performed in a micro-Raman spectrometer (Bruker Optik GmbH, Ettlingen, Baden-Württemberg, Germany).
The micro-Raman spectrometer was first calibrated for zero and then for coefficient values using a silicon specimen. Specimens were analyzed using the following micro-Raman parameters: 20-mW Neon laser with 532-nm wavelength, spatial resolution of ≈3 μm, spectral resolution ≈5 cm −1 , accumulation time of 20 s with 6 co-additions, and magnification of 20X (Olympus UK, London, UK) to beam diameter of ≈1 μm . The spectra were taken at three different sites for each specimen and the values averaged for statistical purposes. Spectra of uncured adhesives were taken as reference. Post-processing of spectra was performed using the dedicated Opus Spectroscopy Software version 6.5 (Bruker Optik GmbH, Ettlingen, Baden- Württemberg, Germany).
The ratio of double-bond content of monomer to polymer in the adhesive was calculated according to the following formula: DC (%) = (1–R cured /R uncured ) x 100, where R is the ratio of aliphatic and aromatic peak areas at 1639 cm −1 and 1609 cm −1 in cured and uncured adhesives.
2.5
Teeth preparation and bonding procedures
The University Ethics Committee approved this part of the experiment under protocol number 1.065.446. Thirty-five caries-free extracted human third molars, collected from patients with age ranging from 18 to 35 years old, were randomly distributed among 7 groups by lottery. The teeth were collected after the patient’s informed consent. Teeth were disinfected in 0.5% chloramine, stored in distilled water and used within 3 months after extraction. A flat dentine surface was exposed on each tooth after wet grinding the occlusal enamel with 180-grit SiC paper. The enamel-free, exposed dentine surfaces were further polished with 600-grit silicon-carbide paper for 60 s to standardize the smear layer.
The adhesives were applied as per manufacturers’ instructions. The dentine surfaces were acid etched with 37% phosphoric acid for 15 s, water rinsed for 15 s and dried with absorbent paper keeping the dentine surface slightly wet. Two adhesive coats were vigorously applied by rubbing the adhesive for 20 s (10 s each coat). Then, gently air stream of 10 s was applied for solvent evaporation and the adhesive layer was light cured with a LED light curing unit for 10 s at 1200 W/cm 2 (Radii-cal, SDI, Bayswater, Victoria, Australia). Resin composite blocks (Opallis, FGM) were buildup on the bonded surfaces in 3 increments of 1.0 mm thick each and each one was individually light activated for 40 s. A single operator carried out all bonding procedures in an environment with controlled temperature and humidity. Five teeth were used for each experimental group.
After storage of the bonded teeth in distilled water at 37 °C for 24 h, they were longitudinally sectioned in both “x” and “y” directions across the bonded interface with a diamond saw in a cutting machine (IsoMet 1000; Buehler, Lake Bluff, USA), under water cooling at 300 rpm to obtain resin-dentine beam-like specimens with a cross-sectional area of approximately 0.8 mm 2 .
The number of premature failures (PF) per tooth during specimen preparation was recorded. The cross-sectional area of each beam-like specimen was measured with the digital caliper to the nearest 0.01 mm and recorded for subsequent calculation of the microtensile bond strength (μTBS) values (Absolute Digimatic, Mitutoyo, Tokyo, Japan). The resin-dentine beam-like specimens originated from the same tooth were randomly divided and assigned to be tested immediately or after 2-year of storage in distilled water at 37 °C. The storage solution was not changed and its pH was monitored monthly. The resin-dentine beam-like specimens from each tooth were then divided as follow:
- •
Two beam-like specimens at each storage period were used for nanoleakage evaluation.
- •
Two beam-like specimens were used to measure the immediate in situ degree of conversion.
- •
Two beam-like specimens at each storage period were used for identification of the presence of copper in the hybrid layer.
- •
The remaining beam-like specimens were submitted to μTBS test at each storage period.
2.6
Microtensile bond strength testing
Each beam-like specimens was attached to a modified device for microtensile bond strength test with cyanoacrylate resin (Super Bonder, Loctite, São Paulo, SP, Brazil) and subjected to a tensile force in a universal testing machine (Kratos, São Paulo, SP, Brazil) at 0.5 mm/min. The failure mode was evaluated at 40x (HMV-2, Shimadzu, Tokyo, Japan) and classified as cohesive in dentine (failure exclusive within cohesive dentine − CD); cohesive in resin (failure exclusive within cohesive resin − CR); adhesive (failure at resin/dentine interface − A), or mixed (failure at resin/dentine interface that included cohesive failure of the neighboring substrates, M). The number of premature failures (PF) was recorded and it was not included in the average mean bond strength.
2.7
Nanoleakage evaluation
All resin-dentine beam-like specimens selected for this test were coated with two layers of nail varnish applied up to within 1 mm of the bonded interfaces. The resin-dentine beam-like specimens were immersed in 50 wt% ammoniacal silver nitrate solution in total darkness for 24 h, rinsed thoroughly in distilled water, and immersed in photo developing solution for 8 h under a fluorescent light to reduce silver ions into metallic silver grains within voids along the bonded interface.
Specimens were mounted on aluminium stubs, polished with 1000-, 1500-, 2000- and 2500-grit SiC paper and 1 and 0.25 μm diamond paste (Buehler Ltd., Lake Bluff, IL, USA). Then, they were ultrasonically cleaned, air dried and gold sputter coated (MED 010, Balzers Union, Balzers, Liechtenstein). The interfaces were observed in a scanning electron microscope in the backscattered mode at 15 kV (VEGA 3 TESCAN, Shimadzu, Tokyo, Japan).
In a way to standardize image acquisition, three pictures were taken of each beam-like specimen. The first picture was taken in the center of the resin-dentine beam-like specimens. The other two pictures were taken 0.3 mm to the left and right of the first one. As two resin-dentine beam-like specimens per tooth were evaluated and a total of five teeth were used for each experimental condition, a total of 30 images were evaluated per group . A technician who was blinded to the experimental conditions under evaluation took them all. The relative percentage of nanoleakage within the adhesive and hybrid layer areas was measured in all pictures using the public domain Image J software, a Java-based image processing software package developed at the National Institutes of Health (NIH) .
Before performing the nanoleakage test a pilot test was conducted to evaluate if the presence of copper in the adhesive could impair the visualization of silver nitrate uptake. For this purpose, we performed scanning electron microscopy (SEM) images of resin-dentine interfaces of all groups without immersion in silver nitrate. Even in adhesive interfaces with the highest copper concentration (1%), copper nanoparticles were not observed using the same parameters described above. And thus, the results of nanoleakage test reflect the amount of silver uptake into unpolymerized areas and/or nanospaces not infiltrated by the resin adhesive but not the presence of copper in the hybrid layer.
2.8
In situ degree of conversion within adhesive/hybrid layers
All resin-dentine beams selected for this test were wet polished using 1500; 2000; 2500 and 4000-grit SiC paper for 30 s each. The specimens were ultrasonically cleaned for 10 min and positioned into micro-Raman equipment. The DC measurements were performed in a micro-Raman spectrometer (Bruker Optik GmbH, Ettlingen, Baden-Württemberg, Germany). The micro-Raman spectrometer was first calibrated for zero and then for coefficient values using a silicon specimen. Specimens were analyzed using the following micro-Raman parameters: 20-mW Neon laser with 532-nm wavelength, spatial resolution of ≈3 μm, spectral resolution ≈5 cm −1 , accumulation time of 30 s with 6 co-additions, and magnification of 100X (Olympus UK, London, UK) to beam diameter of ≈1 μm . The spectra were taken at the resin-dentine interface, in the middle of the hybrid layer within the intertubular dentine, at three different sites for each specimen and the values averaged for statistical purposes. Spectra of uncured adhesives were taken as reference. Post-processing of spectra was performed using the dedicated Opus Spectroscopy Software version 6.5 (Bruker Optik GmbH, Ettlingen, Baden-Württemberg, Germany). The ratio of double-bond content of monomer to polymer in the adhesive was calculated according to the formula described earlier in the materials and methods section in the item in vitro degree of conversion.
2.9
Identification of copper within adhesive itself and in the adhesive/hybrid layers by EDX
For the first analysis, three adhesive discs of each group were produced as described for the microbiological test. After preparation, specimens were stored in a dark vial for 24 h and vertically sectioned in the middle with a diamond saw in a cutting machine (IsoMet 1000; Buehler, Lake Bluff, USA), under water cooling (at 300 rpm). Then, 05 areas of this cross-sectional interface were evaluated under EDX.
In the second part, two beam-like specimens at each storage period previously obtained during specimens sectioning (item 2.5) were used for identification of the presence of copper in the hybrid layer. The analysis of the copper content in each selected beam was performed by field emission scanning electron microscope (FE-SEM) (MIRA 3 TESCAN, Shimadzu, Tokyo, Japan) coupled with an energy-dispersive X-ray spectrometer (EDX). The bonding area was observed and the analysis was focused to the middle of the hybrid layer. One site, randomly determined, per beam-like specimens was examined.
2.10
Statistical analysis
Data for microbiological test (mm), in vitro degree of conversion and in situ degree of conversion (%) were subjected to a one-way ANOVA. Data for microhardness (KNH) was subjected to a two-way ANOVA. For μTBS and nanoleakage, the experimental unit was the tooth with half being tested immediately and the other half after 2 years of water storage. The μTBS and nanoleakage values of all beam-like specimens from the same hemi-tooth were averaged for statistical purposes. The μTBS (MPa) and nanoleakage (%) data of each adhesive were subjected to two-way repeated measures ANOVA. The repeated measure was the tooth at the two-evaluation period. Tukey’s post hoc test was used for pair-wise comparisons (α = 0.05) using the Statistica for Windows software (StatSoft, Tulsa, OK, USA).
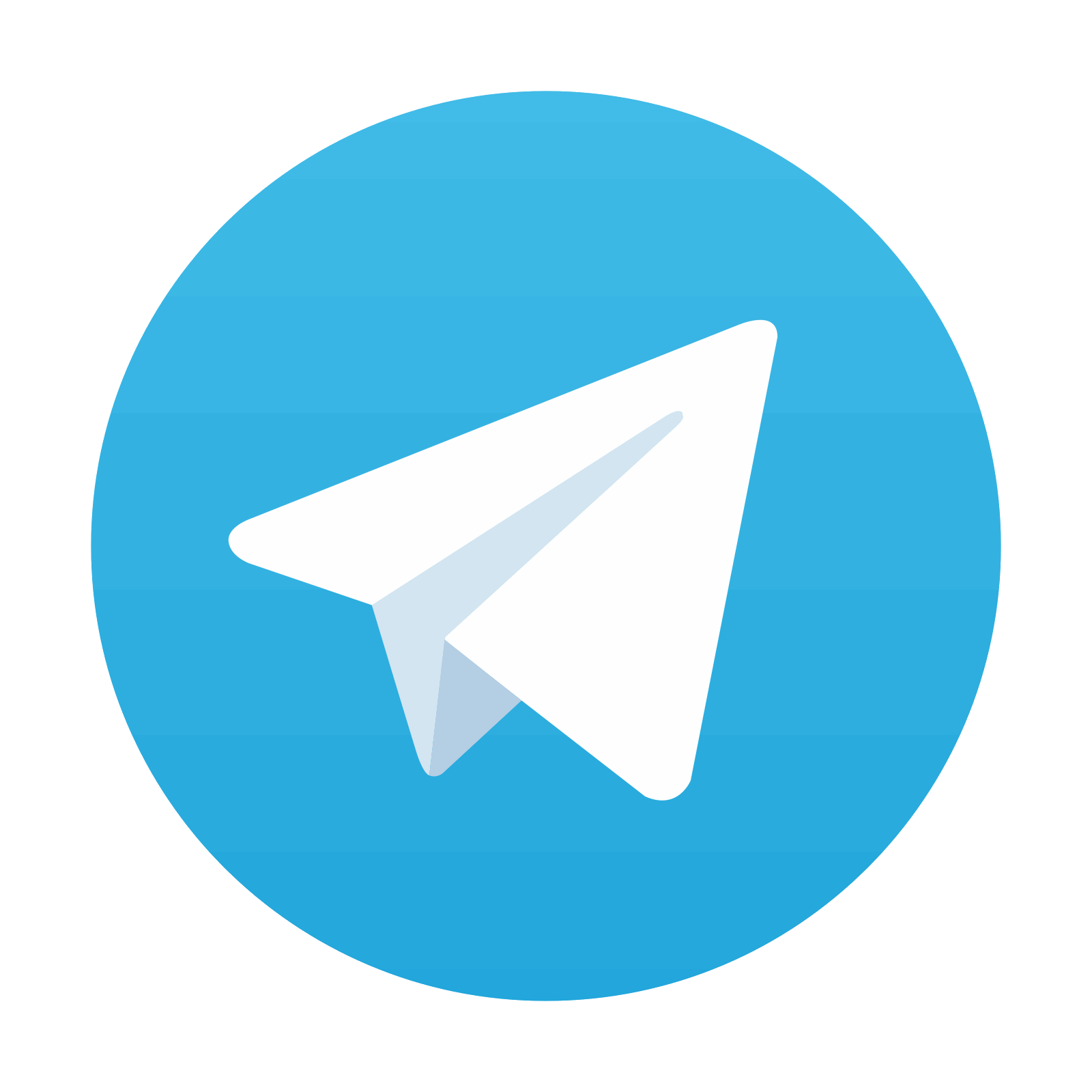
Stay updated, free dental videos. Join our Telegram channel

VIDEdental - Online dental courses
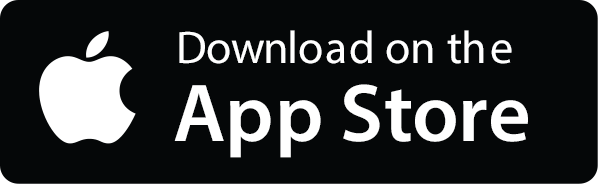
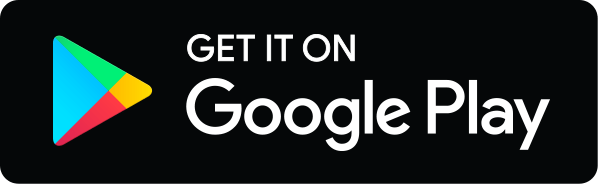