17
The Restorative Dentist and Orthodontist: Orthodontic Implications of Dental Caries, Tooth Fracture, Exposed Dental Pulp, and Esthetic Improvements
Neslihan Arhun1, Ayca Arman‐Özçırpıcı2, Sevi Burçak Çehreli3,4, Kamran Gülşahı5, and Ömur Polat Özsoy6
1 Department of Restorative Dentistry, Faculty of Dentistry, Baskent University, Ankara, Turkey
2 Department of Orthodontics, Faculty of Dentistry, Baskent University, Ankara, Turkey
3 Department of Pedodontics, Faculty of Dentistry, European University of Lefke, Lefke, Northern Cyprus
4 Pediatric Dentist, Specialist, Ankara, Turkey
5 Department of Endodontics, Faculty of Dentistry, Baskent University, Ankara, Turkey
6 Private Practice, Orthodontist, Ankara, Turkey
Primum non nocere.
(Hipoccrates, c.460–c.377 BCE)
An increase in desire for improved facial esthetics and dental appearance is the key motivating factor for orthodontic treatment in every population. These appearance‐conscious patients and/or their families request esthetic treatment plans that usually require a comprehensive interactive approach. Coordinated orthodontic, endodontic, and restorative treatments, with careful consideration of patients’ and their families’ expectations and requests, are critical for successful outcomes and patient satisfaction (Vitale et al., 2004). Clearly, it is the orthodontist’s responsibility to take a proactive role in forming close cooperation between the disciplines to reach the ultimate goal of achieving healthy esthetics and function, while at the same time limiting undesirable consequences and risks of mechanotherapeutics.
Optimized treatment outcomes necessitate close collaboration between the disciplines to evaluate, diagnose, and resolve problems at the following stages of the treatment life cycle:
- Pretreatment evaluation of prognostic factors and the early stages of the orthodontic treatment.
- Orthodontic treatment.
- Emergency orthodontic treatment in trauma cases.
- Immediate postorthodontic period.
- Restorative and/or esthetic touch‐ups.
This chapter reviews and summarizes the need for and benefit of interactions between orthodontists, endodontists, pediatric dentists, and/or restorative dentistry specialists according to these stages of orthodontic treatment.
Pretreatment evaluation and early stages of the orthodontic treatment
Successful orthodontic therapy relies on the accurate assessment of the pretreatment dental and gingival health status with the aid of other dental disciplines. The assessment should include a determination of systemic and/or local factors and obtaining a detailed history of previous trauma to the oral tissues. The initial clinical examination should precede basic radiographic evaluation, which will help the clinician to decide on precautions to minimize the undesirable effects of orthodontic mechanics. It should not be forgotten that every patient is unique and demands and merits a unique treatment plan customized for his or her specific needs.
Initial caries risk assessment: Can the dental team tailor an interdisciplinary approach to end up with a healthy smile?
The orthodontist should take a proactive role throughout the treatment, not only from his or her own perspective, but also from that of the other disciplines, making sure to achieve a healthy, functional, and esthetic smile. The possibility of an increased incidence of caries (i.e. the number of new cavitated or incipient lesions) during a certain time period like orthodontic treatment, or the likelihood of a change in the size or activity of lesions already present, is determined by caries‐risk assessment (CRA; American Academy of Pediatric Dentistry, 2021). Being able to forecast whether new carious lesions may appear in the future will help orthodontists make better decisions, especially when it comes to preventive interventions. It will also help the clinician construct personalized treatment and preventive strategies that are tailored to the needs of each patient. Furthermore, CRA approaches could be employed as an effective health education tool to improve patients‘, parents’, and caregivers’ attitudes and actions toward maintaining excellent oral hygiene and nutritional habits (Bratthall and Petersson, 2005). This is especially important for patients who are receiving orthodontic treatment. Various CRA models have been presented over the years (Twetman and Fontana, 2009). These models vary in many aspects, from the age group to which they can be applied to the risk and protective factors that they include.
The CRA tools basically work on a reasoning‐based model (CAT, CAMBRA, and ADA model) or an algorithm‐driven model (Cariogram, NUS‐CRA, and PreViser). In the first group the clinician takes a brief history and matches that with intraoral findings, then correlates this knowledge with the CRA model’s criteria. In the algorithm‐driven model, computer‐assisted work is done.
Caries Risk Assessment Tool (CAT)
The CAT, from the American Academy of Pediatric Dentistry (AAPD) for patients ≥6 years old, is utilized by answering the following with yes or no:
- Risk factors (social/biological): poverty, low health literacy, frequent exposure (>3 times/day) to between‐meal sugar‐containing snacks, being a recent immigrant, a patient with special healthcare needs.
- Protective factors: optimally fluoridated drinking, fluoridated toothpaste, topical toothpaste, topical fluoride, the patient has dental home/regular dental care.
- Clinical findings: ≥1 interproximal caries lesions, active noncavitated (white spot) caries lesions or enamel defects, low salivary flow, defective restorations, patient wears an intraoral appliance.
Caries Risk Management by Risk Assessment (CAMBRA)
The CAMBRA philosophy was developed in California and has been used for 14 years in the teaching clinics of the School of Dentistry at the University of California San Francisco (UCSF). The items used in the caries risk assessment for patients aged 6 years through adult were grouped into the following:
- Disease indicators (clinical observations): aproximal caries on the radiograph, past caries experience –3 years – and white spot lesions.
- Biological risk factors (expanded to pathological factors): visible plaque, snack habits, saliva deficiencies, reactional drug use.
- Protective factors: fluoridated toothpaste/mouthrinse use, fluoridated community.
Accordingly, low, moderate, high, and extreme risk levels are assigned. Extreme is defined as a combination of high risk and hyposalivation, as determined by visual inspection, medical history, and, if in question, salivary flow rate testing.
American Dental Association (ADA)
- Contributing conditions:
- Fluoride exposure.
- Sugary foods or drinks.
- Caries experience of mother, caregiver, and/or other siblings (no carious lesions in last 24 months, carious lesions in last 7–23 months, carious lesions in last 6 months).
- Dental home: receiving regular dental care in a dental office.
- General health conditions:
- Special healthcare needs (developmental, physical, medical, or mental disabilities that prevent or limit the performance of adequate oral healthcare by patient or caregivers).
- Chemo/radiation therapy.
- Eating disorders.
- Medications that reduce salivary flow.
- Drug/alcohol abuse.
- Clinical considerations:
- Cavitated or noncavitated carious lesions or restorations, visually or radiographically evident (last 36 months considered).
- Teeth missing due to caries (in past 36 months).
- Visible plaque.
- Unusual tooth morphology that compromises oral hygiene.
- Interproximal restorations – 1 or more.
- Exposed root surfaces present.
- Restorations with overhangs and/or open margins; open contacts with food impaction.
- Dental/orthodontic appliances (fixed or removable).
- Severe dry mouth (xerostomia).
Cariogram
Cariogram is an interactive computer program, first developed by Bratthall in 1996 and further refined in 1997 for better understanding of the multifactorial nature of dental caries. It illustrates the “caries risk” and possible interactions between caries‐related factors (Bratthall 1996). The patient is examined and data are obtained for various parameters that are directly associated to caries, as bacteria, nutrition, and susceptibility. The computer program assigns a score to each factor/variable based on a specified scale. It generates a pie diagram with bacteria in the red sector, diet in the dark blue sector, and susceptibility‐related factors in the light blue sector, according to its built‐in formula. Furthermore, some situations are depicted as a yellow sector. The four sectors divide their shares, leaving a green sector that reflects the possibility of preventing caries. The software is available on both Android and IOS platforms for purchasing (Singh et al., 2018: 26–31).
National University of Singapore Caries Risk Assessment Tool (NUS‐CRAT)
Similar to the Cariogram, the NUS‐CRAT classifies children’s caries risk into five risk levels and is expressed as “chance (%) to avoid caries.” NUS‐CRAT considers 11 risk factors (6 items in the community‐screening version) and the risk level is program generated based on a predetermined algorithm. The evaluated risk factors include ethnicity, family socioeconomic status, infant feeding history, diet, fluoride, dental attendance, oral hygiene, past caries, systemic health, and Mutans streptococci and Lactobacilli (Christian et al., 2019).
Effectiveness of caries risk assessment models
A recent systematic review concluded that the evidence for the effectiveness of existing CRA models in assessing and predicting caries lesions is limited (Cagetti et al., 2018); even though Cariogram was used in a few high‐quality studies in children and adults, there is insufficient evidence to conclude that the method is effective in caries assessment and prediction. Other CRA models, such as CAT, CAMBRA, NUS‐CRAT, and PreViser, may be effective in clinical settings; however, there is limited scientific evidence to date. Christian et al. (2019) systemically compared several CRA models including CAMBRA, Cariogram, AAPD, and a few more for patients aged <6 years. Due to the results of this review, only the NUS‐CRAT received a high degree of evidence for criterion validity and responsiveness, as well as individual sensitivity and specificity values of at least 75% and 85%, respectively. The limited evidence for CRA tools was mentioned by Twetwan (2016), who underlined that there is no clearly superior method for predicting future caries and no evidence to support the use of one model, program, or technology over any other. On the other hand, the article concluded that multivariate models display better accuracy than the use of single predictors and this is especially true for preschool children. Twetman (2016) emphasized the fact that there is a great need for methods that more accurately reflect the activity in the biofilm.
With all this in mind, the authors recommend the following in order to increase CRA tool effectiveness (Cagetti et al., 2018):
- A caries risk assessment should be carried out at the patient’s first dental visit and reassessments should be done during treatment.
- The risk category should be linked to appropriate preventive care, with recall intervals based on individual need.
- In preschool children CRA tools including socioeconomic and sociodemographic information, baseline caries, and etiological factors resulted in moderate/good accuracy, with sensitivity >80% and specificity >70% (Mejàre et al., 2014). In general, similar models were less accurate in school children and adolescents, who are the vast majority of orthodontic patients.
Indicators of past dental caries experience remain the strongest predictors of risk of dental caries among school children and older adult populations. In addition, dental caries in the primary teeth are a strong predictor of future dental caries experience (Swedish Council on Technology Assessment in Health Care, 2008). If an orthodontic patient has an active lesion or treated carious teeth, this patient should receive professional preventive measures along with orthodontic therapy.
When the limited evidence regarding the effectiveness of CRA is evaluated, the adoption of CRA tools can be viewed as a tool for patient health education, as it identifies the risk variables implicated in illness onset (Cagetti et al., 2018). These tools are particularly important in patients undergoing orthodontic treatment, as the nature of the treatment may increase caries susceptibility.
In addition to evaluating the child’s status, the orthodontist should also evaluate the family, which plays a great role in children’s oral health. Families, as the smallest social unit with which children interact, play a critical role in shaping a child’s oral health behavior (Fisher‐Owens et al., 2007; Abbasoglu and Kuvvetli, 2021). The psychosocial factors of a parent are major determinants of children’s oral health behavior. These factors influence a child’s oral health via parent–child relationships and are also a reflection of mother–father relationships in a child’s behavior. For example, the cognitive qualities of the mother, such as knowledge of healthy oral behaviors, are significantly related to a child’s proclivity to establish oral hygiene routines. A recent survey conducted on 8446 families revealed that parents with higher education levels have better oral health knowledge and benefit from preventive measures such as pit and fissure sealants. Furthermore, children of higher‐educated parents have better dental hygiene routines (Chen et al., 2020). Eating a lot of sweets, using the wrong brushing method, starting brushing at later ages (after the first year of life), and not brushing regularly are major risk factors for early childhood caries. Emphasizing oral health knowledge to parents and guardians, using proper brushing methods, and limiting frequent sweets intake are all important factors in the prevention of dental caries (Sun et al., 2017).
Nonrestorative or minimally invasive approaches and fighting cavities for good
The notion of active surveillance (prevention therapies and close monitoring) of enamel lesions is based on the idea that treatment of disease is only necessary if it progresses, and that caries can be stopped without therapy.
Minimal‐intervention dentistry is a holistic approach to caries care that combines caries lesion control with minimal surgical intervention. Tissue preservation is the major goal, which includes early caries identification and nonoperative therapy, as well as minimally invasive restorative methods (Frencken et al., 2012; Machiulskiene et al., 2020). The following are short descriptions of noninvasive or minimally invasive approaches that can be performed easily during orthodontic treatment. One should keep in mind that for clinical success, all of these techniques should be combined with good oral hygiene and proper diet, as well as sugar intake restriction.
Nonrestorative/nonoperative cavity treatment
This approach makes the cavitated caries lesions accessible to tooth cleaning by removal of overhanging enamel margins (Machiulskiene et al., 2020).
Silver diamine application
Silver diamine fluoride (SDF; 38%) is a clear liquid that contains a high concentration of fluoride ions (44,800 ppm) and combines the antibacterial effects of silver with the remineralizing effects of fluoride (Crystal and Niederman, 2019). SDF is employed as a promoter of the arrest and remineralization of active carious lesions, but it stains teeth black. The clinician should inform the patient that this discoloration is permanent (Horst et al. 2016). SDF is also effective for arresting active dentinal lesions and for teeth with exposed root surfaces that cause dentinal hypersensitivity, and may also be beneficial for permanent molar teeth with molar–incisor hypomineralization (MIH), since plugging the dentinal tubules may lessen sensitivity. As for safety, one drop of SDF contains 2.24 mg of fluoride (a typical dose of 5% fluoride varnish contains 11.3 mg fluoride). Thus, it is considered that biannual application of dental fluorides should not be a risk in children (Seifo et al., 2020; Figure 17.1).

Figure 17.1 Silver diamine fluoride application on the distal surface of the second primary molar.
Atraumatic restorative treatment
Atraumatic restorative treatment is a caries management approach that uses hand instruments for opening caries cavities and removing decomposed carious dentine, followed by restoration with a high‐viscosity glass ionomer. The technique can be tooth saving in small children and class I lesions.
Ultraconservative caries therapy
Ultraconservative caries therapy describes the approach of bonded and sealed restorations placed directly over frank cavitated carious lesions extending into dentine (Machiulskiene et al., 2020).
Evaluation of the prognostic factors of the existing dentition
The decision of whether or not to extract tooth/teeth is an important step in designing concrete orthodontic treatment procedures. If the orthodontist judges a case as one requiring extraction, the next step will be the decision regarding which tooth/teeth to extract (Yagi et al., 2009). There may be multiple options and choosing one of the potential teeth requires consideration of parameters that might require a consultation with specialists from other disciplines. For example, a history of root canal treatment or the presence of periapical lesions, restorations, and carious teeth complicates the decision about the site of extraction. In such cases, the decision should be the one that optimizes the orthodontic treatment prognosis with less invasive operative intervention (Figure 17.2).
Teeth with deep or extremely deep caries: Restorative strategies
Facing teeth with deep caries lesions presents challenge to every orthodontist, leading to hesitation about incorporating this uncertain prognosis into the game plan. Cariology experts (Innes et al., 2016) and the European Society of Endodontology (ESE; European Society of Endodontology developed by:, Duncan et al., 2019) reached a consensus and clarified a distinction between deep and extremely deep caries. The ESE defined deep caries as “Caries reaching the inner quarter of dentine, but with a zone of hard or firm dentine between the caries and the pulp with a risk of pulp exposure during operative treatment” and extremely deep caries as “the carious demineralized dentine penetrating the entire thickness of the dentine, without a radiopaque zone separating the lesion from the pulp with microorganism penetrating into the critical zone of tertiary dentine including the pulp” (Bjørndal 2018). However, the diagnosis of the exact extent of the disease is challenging for many experienced orthodontists, restorative dentistry specialists, and endodontists, compromising choice of the most appropriate treatment strategy. Evaluation of the radiographic image is inevitably not sufficient to make an exact diagnosis of the proximity of the lesion to the pulp (Bjørndal et al., 2019). Likewise, the relation between clinical symptoms and the histopathological state of the pulp has presented contradictory findings where asymptomatic teeth may have presented pulpal involvement (Iwamoto et al., 2006; Iqbal et al., 2007).
As our biological conceptualization of pulpal response to caries has evolved, management strategies have shifted from traditional invasive techniques to contemporary philosophies that aim at arresting caries progression, remineralization of caries‐affected dentine by bioactive materials, and maintenance of the pulp’s developmental, defensive, and proprioceptive functions in the light of minimally invasive dentistry (Smith, 2002; Edwards et al., 2021).
The decision making for a deep or extremely deep lesion mostly relies on comprehensive evaluation of the patient with a detailed history of signs and symptoms, and appropriate radiographic and clinical assessment (Allison et al., 2020). Even so, identification of reversible and irreversible pulpitis clinically has been shown to be both erratic and subjective (Villa‐Chávez et al., 2013; Jespersen et al., 2014). Thus, a new classification system to judge the true repair potential of the pulp is proposed by Wolters et al. (2017). This new classification system eliminates the terms “reversible” and “irreversible,” suggesting that the inflammatory process is a continuum from initial to mild, moderate, and severe inflammation. With the advent of conservative treatment options, bacterial invasion of the pulp may be prevented and severe pulpal inflammation, necrosis, root canal treatment, and even extraction may be avoided or at least postponed.
The initial step of conservative treatment of deep and extremely deep caries lesions begins from the carious tissue removal stage. The International Caries Consensus Collaboration reached a consensus that complete excavation of carious tissue to hard dentine is overtreatment and favored selective or stepwise removal techniques (Schwendicke et al., 2016).
Stepwise caries removal technique involves nonselective removal of carious tissue in two sessions. In the first session, all carious dentin is removed, including the most necrotic and contaminated dentin on the pulp wall, leaving some soft tissue nearest to the pulp, with temporary sealing for 2–6 months to give the pulp the chance to remineralize and reduce the risk of pulpal exposure by stimulating the deposition of tertiary dentin (Maltz et al., 2012). In the second session the cavity is reopened, the softened remaining carious tissue is removed, and the final restoration is performed. However, the need for a revisit, possible failure of the temporary restoration, and the possibility of iatrogenic pulpal exposure in the second visit are the basic pitfalls of the stepwise technique (Maltz et al., 2018). To overcome these pitfalls, and as a result of debates about the temporization and reopening of the restoration, a selective caries removal technique is proposed. This technique is less invasive and utilizes nonselective removal of carious tissue from the surrounding cavity walls, allowing remineralization of the affected dentin in the pulpal wall, and restoring the tooth definitively (Maltz et al., 2018; Casagrande et al., 2017). The ultimate goal of both techniques is to reduce the bacterial load by removing the infected dentin to preserve pulp vitality (Reeves and Stanley, 1966). Thus, the dentin–pulp complex can continue reactionary and reparative dentinogenesis to reduce the likelihood of further injury (Duncan et al., 2019; European Society of Endodontology developed by:, Duncan et al., 2019).

Figure 17.2 (a–j) Heavily restored four first permanent molars were extracted instead of premolars due to poor prognosis of the molar teeth in order to correct the Class II malocclusion. Crosses represent missing teeth. (k) The lower third molars erupted and replaced the second molars.
In 2019, ESE updated the definition of indirect pulp capping to “application of a biomaterial onto a thin dentine barrier in a one‐stage carious‐tissue removal technique generally to hard dentine” (European Society of Endodontology developed by:, Duncan et al., 2019). The emphasis on application of a biomaterial is the backbone of vital pulp therapy, which refers to avoiding monomer and bacterial ingress stimulating dentin formation. In fact, the previous version was a “procedure in which a pulp is covered with a protective dressing or cement placed over a thin partition of remaining dentine” (European Society of Endodontology, 2006).
Following stepwise or selective caries removal, a bioactive material should be placed before definitive restoration. Calcium silicate cements – mineral trioxide aggregate (MTA) or Biodentine™ (Septodont, New Castle, DE, USA) or glass ionomer cement (GIC) may be placed in this therapeutic approach. The “tricalcium silicate‐based dentin replacement and repair material” Biodentine (Malkondu et al., 2014) shows promising results on biocompatibility as well as bioactivity‐inducing proliferation of pulp stem cells (Luo et al., 2014; Laurent et al., 2012) and mineralization (Loison‐Robert et al., 2018). Evidence is currently equivocal between the use of GIC and calcium silicate cements or conventional MTA, as at present there is an absence of long‐term studies (Hashem et al., 2019). However, the superiority of calcium silicate cements over calcium hydroxide liners is proven (Petrou et al., 2014).
A recent systematic review and meta‐analysis reported selective caries removal to be superior than the stepwise technique (Barros et al., 2020). Furthermore, a Cochrane systematic review also reported reduced risk of pulp exposure (Ricketts et al., 2013). Bjørndal et al. (2017), in a randomized clinical trial, pointed out that the stepwise carious removal group had a significantly higher proportion of pulps with sustained vitality without apical radiolucency versus nonselective carious removal of deep carious lesions in adult teeth at five‐year follow‐up.
Pulpotomy, the removal of all the coronal pulp tissue, has been recommended as an alternative treatment to pulp capping where appropriate (Asgary and Eghbal, 2010; Simon et al., 2013). The histologically based idea beneath this philosophy is that the limited inflammation in the coronal part of the pulp tissue may be removed without interfering with the roots (Ricucci et al., 2014). This technique is routinely accomplished in pediatric dentistry to allow the radicular process and apexogenesis, and may be a promising approach for mature teeth (Simon et al., 2013; Taha et al., 2017). In the future, practical issues surrounding coronal pulpotomy will also need investigation, including the lack of response to pulp sensibility testing and the likelihood of pulp canal obliteration, which will compromise potential reentry.
Immature permanent teeth with pulp necrosis and apical periodontitis
The American Association of Endodontics Clinical Considerations for Endodontic Regenerative Procedures (REPs) identifies three goals for the success of REPs: symptom relief and observation of bone healing; increased root wall thickness and/or a desirable, though not necessary, root length increase; and finally a positive response to cold test and electric pulp tests. However, it is impossible to achieve all these goals at once, and often only one of them is achieved (Chen et al., 2012; Natera and Mukherjee, 2018). REPs were accelerated in the early 2000s with the publication of two remarkable case studies (Iwaya et al., 2001; Banchs and Trope, 2004) and nowadays REPs are becoming the preferred treatment option for immature permanent teeth with pulp necrosis and apical periodontitis (Garcia‐Godoy and Murray, 2012; Kim et al., 2012; Lin et al., 2014; Natera and Mukherjee, 2018).
It is important to consider that complete closure of the root apex occurs approximately three years after a tooth erupts in the oral cavity, and the loss of pulp vitality during this time stops further root development. Immature teeth diagnosed with pulp necrosis have been conventionally treated with apexification methods using long‐term calcium hydroxide therapy (Cvek, 1992) or immediate placement of an MTA apical plug (Witherspoon et al., 2008). Although these treatments usually end with resolution of the disease symptoms, they provide little or no benefit for continued root growth (Bose et al., 2009). The root closure process includes root elongation, apical closure, and further thickening of the root. REPs have been described as biological methods created to stimulate apical maturation and thicken dentinal walls (Murray et al., 2007).
In orthodontic dental treatment, reshaping or resorption of the apical part of the root during the movement of the tooth is effective (Hamilton and Gutmann, 1999). However, there is no study that has negative views on orthodontic treatment in patients undergoing REP. In a case report followed for four years, it was shown that a tooth treated with REP responded to orthodontic treatment in a similar way to teeth that were not treated with REP. Therefore, REP probably does not have a negative effect on orthodontic treatment (Natera and Mukherjee, 2018; Figure 17.3).
Survival of hypomineralized teeth (molar–incisor hypomineralization)
Molar–incisor hypomineralization (MIH) is defined as demarcated, qualitative developmental enamel defects of systemic origin in at least one permanent molar with or without incisors being affected (Weerheijm et al., 2003; Weerheijm 2004). Research revealed that the demarcated defects that are present on molars and incisors with MIH have also been present on other teeth such as primary molars. This phenomenon is called hypomineralized second primary molars (HSPM). Thus, HSPM can be considered a predictor for MIH (Elfrink et al., 2015).
MIH is considered a worldwide clinical problem with a global prevalence of 14.2%, ranging from 0.5% to 40.2% according to different studies (Kusku et al., 2008; Padavala and Sukumaran, 2018). The condition is associated with significantly increased dental treatment needs, especially in severe cases. Giuca et al. (2020) states that children with MIH undergo dental treatment nearly 10 times more than unaffected children. Restorative treatment is initiated with preventive measures and ends with extractions in severe cases. The preventive measures include casein phosphopeptide–amorphous calcium phosphate (CPP‐ACP), glass ionomer sealants, topical application of fluoride varnish, tricalcium phosphates, and more recently, SDF application.

Figure 17.3 A 14‐year‐old female patient with deep/extremely deep caries lesions on molar and premolar teeth. Regenerative endodontic treatment of the lower left second molar tooth. (a) A periapical radiograph depicting periapical radiolucency associated with tooth # 37 with an immature apex. (b) Placement of white mineral trioxide aggregate below the cementoenamel junction and a coronal seal with composite. (c) Thirteen‐month follow‐up periapical radiograph of the tooth shows a decrease in the size of the periapical radiolucency, periapical healing, and root maturation.

Figure 17.4 (a) Molar–incisor hypomineralization (MIH) treated with silver diamine and glass ionomer. (b) MIH treated with stainless steel crown and resin composite. (c) Untreated MIH.
The remineralization function of the active molecule, CPP‐ACP crème, has been well documented in the literature in the treatment of hypomineralized white spot lesions (WSL), with calcium and phosphate levels recovering to nearly that of normal, mature enamel at the three‐year mark (Baroni and Marchionni, 2011). The product is also effective in treating hypersensitivity in MIH. In a clinical study conducted on 40 patients aged from 8 to 13 years old who had a molar with MIH hypersensitivity, the use of CPP‐ACP resulted in a significant improvement in dental sensitivity (Pasini et al., 2018). Recently, Kumar et al. (2022) compared daily single application of CPP‐ACP cream and professional application of fluoride varnishes every three months on enamel slabs prepared from MIH‐affected teeth in situ. The intergroup comparison revealed no significant differences between the two groups at either baseline or six months after intervention. The usage of this innovative product is also discussed in the “Immediate postorthodontic period” section of this chapter.
Even if clinicians initiate preventive measures along with early detection of the phenomenon, in moderate to severely affected cases they still may face many complications like enamel breakdown, caries occurrence, restoration failure, need for endodontic treatment, or the need for extraction. The permanent first molars (PFMs) severely affected with MIH are vulnerable to pulpal inflammation even in the very early stages of their presence in the mouth, which in turn complicates the endodontic treatment due to open apices (Lygidakis et al., 2010; Rodd et al., 2007; Figure 17.4).
Another problem with MIH‐affected PFMs is that these teeth are hypersensitive and treatments can be painful due to difficulties in anaesthetizing. As a consequence of repeated painful treatments, children with severe MIH have been reported to display more behavior management problems and anxiety during dental treatments (Jälevik and Klingberg, 2002). As a basic rule, PFMs should only be extracted if their preservation until the third decade of life seems unlikely (Schopf, 2008). The challenging and never‐ending treatments of severely MIH cases are thereby good candidates for this rule. Thus, clinicians may prefer extraction over treatment in cases with poor prognosis.
Poor long‐term prognosis of MIH‐affected PFMs can be expected (Fayle, 2003; Jälevik and Klingberg, 2002) for molars with a severe and large‐scale hypomineralization area that needs comprehensive restorative treatment, fast posteruptive breakdown and porosities reaching into the dentin, uncontrollable periodontal and/or endodontic problems, or repeatedly failed rehabilitations where patient cooperation is also lacking.
Orthodontic alignment in the postextraction period (i.e. without spontaneous alignment) is another issue in cases with MIH. Thilander and Skagius (1970) reported that the best timing for the extractions of PFMs was at 8–10 years of age. Kirschneck and Proff (2016) reported that the appropriate timing for molar extraction – between 8 and 11.5 years of (dental) age – is critical for the efficacy of orthodontic extraction therapy in MIH. Early referral of children with MIH to an orthodontist, as well as an orthodontic evaluation in the early stages of mixed dentition, is therefore recommended to allow for proper scheduling of orthodontic extraction therapy and space closure in patients who would benefit from this treatment strategy.
One retrospective cohort study had evaluated extraction of MIH‐affected PFMs and subsequent spontaneous alignment of the second molars (Jälevik and Möller, 2007). Overall 27 children aged 5.6–12.7 years at the time of extraction were evaluated. A median of 2.6 molars were extracted in each participant (overall number was 70 extracted molars). Seven participants (26%) had common malocclusions like crowding or crossbites; 79% of the extractions took place in a quadrant where a third molar was present; in three participants all third molars were missing, while in two participants two third molars were missing. The eruption of the second molars followed after a mean period of six years after extraction. In the maxilla 55% and in the mandible 47% of second molars aligned spontaneously and did not require orthodontic intervention. The authors stated that their study shows favorable spontaneous development of the permanent dentition after first molar extraction in the majority of cases. Unfortunately, that valuable study lacks information on influencing factors for alignment (like stage of second molar development or vertical skeletal dimension).
The other issue with MIH is coexisting dental anomalies, mainly hypodontia. A study by Walshaw et al. (2020) inspected children with MIH and reported that in total 29% of the patients had a dental abnormality. Coexisting hypodontia was found in 12% of cases, with lower second premolars being the most affected teeth. Concurrent ectopic first permanent molars were seen in 8% of cases, while infraocclusion of one or more primary molars was found in 9%. There was abnormal morphology in 9% of the teeth, including macrodont and microdont teeth.
Thus, the “take‐home message” for the clinician should be that MIH‐affected PFMs should be evaluated carefully early in the mixed dentition by a pediatric dentist and an orthodontist. It is wise to remember that severe, even moderate cases may cause complications resulting in tooth loss. On the other hand, the clinical management of mild cases can be undertaken successfully with a deep knowledge of appropriate preventive materials.
Amelogenesis imperfecta
Amelogenesis imperfecta (AI) is an uncommon developmental defect of tooth enamel that occurs at a rate ranging from 1 in 4000 to 1 in 14,000 in Western cultures (Witkop, 1988; Kida et al., 2002). AI enamel is excessively thin, brittle, pitted, and/or discolored, causing considerable feeding problems and discomfort in individuals. It is also linked to bad social results, humiliation, and poor esthetics (Crawford et al., 2007).
AI is a hereditary condition characterized by mutations in genes AMEL, ENAM, MMP20, KLK4, and FAM83H, and characterized by a wide variety of phenotypes (Gadhia et al., 2012). The type of AI determines whether heredity is autosomal dominant, autosomal recessive, or X‐linked. Although there are many kinds of AI, the main abnormality in each is hypoplasia, hypomaturation, or hypocalcification. The hypoplastic kind of enamel is thin, pitted, or fissured. The hypocalcified enamel is powdery, varies in color, and erodes easily.
Coexisting dental anomalies may include delayed tooth eruption, impacted teeth, congenitally missing teeth, open bite, pulpal calcifications, taurodontism, and root malformations, which may lead to resorption during orthodontic treatment (Witkop, 1988; Arkutu et al., 2012). Arkutu et al. (2012) described all the features of AI that may compromise orthodontic approaches in their review.
Like MIH, AI also needs an interdisciplinary approach for its treatment. Tight contact between the interdisciplinary team is required for treatment planning in the immediate, transitory, and long‐term stages (Figure 17.5).
The major problem of weak and delicate enamel may be overcome with alternative approaches. If possible, choosing removable approaches would be in the best interest of the patient (Arkutu et al., 2012). Traditional banded appliances are out of date, yet they may be utilized to solve some of the adhesion problems. With an AI patient, the orthodontist will face clear difficulties in attachment and bracket bonding. Because they are less reliant on micromechanical adhesion phenomena, GIC‐based adhesives are expected to increase appliance retention and aid in the mitigation of future enamel demineralization. In AI, the use of sodium hypochlorite to eliminate excess protein around the enamel crystals and enhance etch quality has been documented (Sarıoğlu et al., 2006). However, since this study was done on primary teeth, the evidence for enhanced bracket retention with these approaches as well as increasing etch times in AI remains weak (Venezie et al., 1994; Hiraishi et al., 2008).
As for the problem of impacted teeth, even in the lack of clear supported data, preventive measures such as deciduous canine removal and space construction should be used (Parkin et al., 2009a). In addition, as for the taurodont and malformed roots, an endodontist should keep an eye on AI patients throughout the orthodontic treatment.

Figure 17.5 (a–k) Orthognathic surgical and restorative treatment of a patient with severe Class III open bite malocclusion and amelogenesis imperfecta. Successful bonding is a challenge in patients with amelogenesis imperfecta.
Endodontically treated teeth
Successful root canal treatment relies on adequate removal of microorganisms and prevention of recolonization or reinfection through the placement of a root canal filling that obliterates the canal space and a restoration with a good coronal seal (Briggs and Scott, 1997). The benefits of root canal treatment are retention of the natural tooth in the dental arch and facilitation of the restoration to conserve the remaining crown and root structures, preservation of the alveolar bone and accompanying papillae, and maintenance of pressure and tension perception by paradental mechanoreceptors. However, such a retained tooth may be at risk of future root fracture and development of caries or periodontal disease after orthodontic treatment (Torabinejad et al., 2007). Epidemiological radiographic surveys have revealed a relatively low frequency of high‐quality root canal fillings, ranging from 14% to 65% (Eriksen et al., 2002). Epidemiological studies of root canal–treated teeth in different groups performed outside of a university‐sponsored clinic produced more adverse treatment results, with rates of highly insufficient root canal fillings and apical periodontitis (between 40 and 65%) related to endodontically treated teeth (Gillen et al., 2011).
Endodontic outcomes are difficult to predict, since nonendodontic factors such as the quality of the subsequent restoration and the remaining tooth structure contribute to the true prognosis. Whenever the decision has to be made about whether to perform endodontic treatment or to extract a tooth, orthodontists should consult with endodontists, taking into account pre‐, intra‐, and postoperative factors, such as the patient’s age, socioeconomic class, vital or necrotic pulp, and presence of periapical infections (Travassos et al., 2003). It is important to realize that the presence of periapical lesions, increased age, and irregular canal anatomies, especially in molars, may compromise the endodontic outcome. Moreover, procedural errors during canal instrumentation, rinsing with irrigation solutions, and medicaments and fillings may play a crucial role in long‐term prognosis. These factors should be evaluated based on good clinical judgment along with input from endodontists, so that the patient is provided with an optimized treatment plan that is at the same time cost‐effective.
The time at which a tooth may be moved after completion of endodontic therapy is the most frequently asked question and presents uncertainties in orthodontic and endodontic clinical practice. Endodontically treated teeth can be moved orthodontically as readily as teeth with vital pulp. But the effects of orthodontic treatment on endodontically treated teeth depend on a variety of factors such as the applied force, the seal of the canal, any previous history of trauma, and the patient’s clinical symptoms (Consolaro and Consolaro, 2013; Consolaro et al., 2020). If teeth require root canal treatment during orthodontic movement, it is recommended that the root canals be cleaned, shaped, and an interim dressing of calcium hydroxide be placed. Canal obturation is accomplished after orthodontic treatment (Hamilton and Gutmann, 1999). If orthodontic treatment might take too long to finish, a gutta‐percha filling should be placed in treatment, because the calcium hydroxide filling may make the tooth prone to fracture during this period.
Congenitally missing and peg‐shaped laterals
Tooth agenesis (hypodontia) is the most commonly observed craniofacial developmental anomaly, which involves absence of at least one permanent tooth, excluding third molars, with a prevalence of 3.5–10% in the permanent dentition and exhibiting variable prevalence in different races or ethnic groups, with a modest preference for females (Krassnig and Fickl, 2011; Khalaf et al., 2014; Arte et al., 2017). The most frequent permanent missing teeth are the second premolars and maxillary lateral incisors (Rizos and Spyropoulos, 2004; Al‐Ani et al., 2017). This form of hypodontia can be bilateral or unilateral; such a condition can occur with a contralateral small or peg‐shaped lateral incisor, adding complexity to the restorative options (Mirabella et al., 2012). Bilateral agenesis of maxillary permanent lateral incisors occurs more often than unilateral agenesis (Polder et al., 2004).
Studies have shown that different dental and craniofacial characteristics accompany agenesis. Bimaxillary retrognathism, a Class III skeletal pattern, and reduced vertical and transverse dental arch dimensions may be skeletal companions (Vucic et al., 2016). Microdontia resulting in spacing and displacement of adjacent teeth (Hobkirk et al., 2010), taurodontism (Goodman et al., 1994), ectopic eruption (Peck et al., 1998), delayed eruption (Dhanrajani, 2002), ankylosis, and infraocclusion may be seen on a dento‐alveolar level. In addition, hypodontia patients may display reduced alveolar bone development, which may be restricted to a particular region or generalized to the whole alveolar process. These patients may have an older look as a sequelae of more prominent mandible and lips. Also, the freeway space may be increased, compromising esthetics and function.
Hypodontia can be classified as syndromic or nonsyndromic. Syndromes such as ectodermal dysplasia, Van der Woude syndrome, or Down syndrome and craniofacial microsomia are more commonly associated with severe hypodontia and can be either sporadic or familial, whereas in nonsyndromic conditions such as cleft lip and palate, hypodontia is more commonly seen in the permanent dentition and ranges in severity (Cobourne, 2007; Pagán‐Collazo et al., 2014).
Familial hypodontia is complex and multifactorial, influenced by a combination of gene function, environmental interaction, and developmental stage (Lammi et al., 2004). Although congenital absence of teeth typically has a complex and poorly defined genetic background, since more than 200 genes are involved in tooth formation (De Coster et al., 2009), many affected individuals have a family history of developmental dental anomalies (Hobkirk et al., 1995). Genetic transmission of hypodontia has demonstrated variable penetrance, with reported polygenic, epigenetic, and environmental influences (Parkin et al., 2009b). Mutations in transcription factors (Javed et al., 2014) MSX1, PAX9, and AXIN 2 have been identified in families with an autosomal dominant oligodontia (Tallón‐Walton et al., 2014). These mutations have been implicated in arresting tooth development at the bud stage (Mitsui et al., 2014).
Environmental factors that lead to arrested tooth development may include factors that cause failure of tooth bud cell proliferation from the dental lamina (Al‐Ani et al., 2017). This may be due to infection (e.g. rubella, osteomyelitis), trauma in the dental region such as fractures, surgical procedures on the jaw and extraction of the preceding primary tooth, drugs (e.g. thalidomide), or chemotherapy or radiotherapy at a young age (Al‐Ani et al., 2017).
The peg‐shaped lateral phenotype has a strong genetic etiology, and peg‐shaped laterals have been associated with tooth agenesis (Arte et al., 2001). The prevalence of peg‐shaped laterals has been reported to be 1.8% (Hua et al., 2013). The prevalence of bilateral and unilateral peg‐shaped lateral incisors is the same, and the left side is more common than the right side (Hua et al., 2013). Peg‐shaped lateral prevalence has been shown to be 7.5% in nonsyndromic cleft patients (Sæle et al., 2017).
Severe hypodontia with excessive spacing and compromised esthetics have been reported to cause functional and psychosocial burdens (Robertson and Mohlin, 2000), such as bullying in school, which led to depression, loneliness, anxiety, low self‐esteem, and underachievement, especially during the sensitive adolescent period (Kavadia et al., 2011; Johal et al. 2021). Meaney et al. (2012) reported that adolescents become more aware of their esthetics as they grow up, and their primary motivation for treatment is esthetics.
A logical hierarchy of decisions should be followed to create a stable occlusion, periodontal health, and acceptable dental and facial esthetics after diagnosing agenesis (Cocconi, 2020). To design a custom treatment plan, it is of fundamental importance to analyze key factors such as the patient’s age, type of sagittal malocclusion, presence or absence of crowding, profile type, shape and color of the canine, smile line, arch length, tooth size discrepancy, ridge thickness, existing occlusion, patient’s expectation from treatment, and possible cooperation level of the patient (Silveira et al., 2016; Hsu and Lee, 2019).
In fact, the decision requires an interdisciplinary treatment plan that mostly involves restorative touch‐ups, which will be discussed in the last section of this chapter under “Esthetic improvements.”
Interactive collaboration during orthodontic treatment
Orthodontic treatment will succeed when its advantages outweigh any adverse sequelae. To prevent, minimize, and manage the iatrogenic effects of orthodontic mechanics, the clinician should examine both the hard and soft oral tissues that may be affected by the orthodontic treatment (Shaw et al., 1991), just like at the treatment planning stage before treatment initiation.
Cariogenic potential and white spot lesions
After the decision about the final treatment plan, before the full bond‐up appointment, the clinician should take preventive measures against caries. Dental caries in the enamel is unique among other infective diseases in the human body, as enamel is both acellular and avascular; thus, enamel cannot heal itself by a cellular repair mechanism (Zero, 1999). In orthodontic patients, caries starts as decalcification areas adjacent to fixed orthodontic appliances. Several studies showed that orthodontic attachments caused an increase in occurrence of cariogenic Streptococcus mutans, and the number of bacteria was found to be four times higher compared with the number at the beginning of treatment (Ahn et al., 2007; Rosenbloom and Tinanoff, 1991). These acidogenic bacteria produce byproducts of organic acids in the presence of fermentable carbohydrates, further lowering the pH of the plaque. As the pH drops below the threshold for remineralization (pH = 4.5), enamel demineralization/decalcification occurs. The first clinical evidence of this demineralization is visualized as a WSL, which has been defined as “sub‐surface enamel porosity from carious demineralization” that represents itself as “a milky white opacity” caused by the changes in enamel translucency and light scattering (Bishara and Ostby, 2008).
WSL development is a very rapid process and they may be apparent within four weeks after the orthodontic bond‐up appointment (Ogaard et al., 1988). Their prevalence is reported to vary from 4.9% (Gorelick et al., 1982) to 84% (Mizrahi, 1982) of tooth surfaces. More than 50% of subjects may experience an increase in the number of WSLs with fixed orthodontic appliance therapy (Artun and Brobakken, 1986). Boersma et al. (2005) observed that 97% of all their subjects and on average 30% of the buccal surfaces in a person were affected. Again, on average, 40% of the surfaces in males and 22% in females showed WSLs. Briefly, any tooth in the mouth can be affected, with the common ones being the maxillary lateral incisors, maxillary canines, and mandibular premolars. Diagnosis, prevention, and treatment of WSLs are all crucial to prevent tooth decay as well as to minimize the tooth discoloration that could compromise the smile esthetics.
The acquired biofilm (the pellicle) in the intact enamel surface participates in dynamic physico‐chemical equilibrium with the oral fluids (Zero, 1999; Ten Cate and van Loveren, 1999). When the pH of the oral fluids goes below the physiological norm, calcium and phosphate ions diffuse from the hydroxyapatite mineral in the enamel to the pellicle and into the oral cavity (demineralization). When the pH of the oral fluids rises to the norm, the calcium and phosphate ions in the supersaturated saliva are transmitted through the pellicle into the enamel following the laws of chemical equilibrium (remineralization). Within this cycle, destructive demineralization and restorative remineralization occur either simultaneously or alternately. If this cycle is not prevented and demineralization predominates, WSLs will be the result and, once they are established, it is extremely difficult, or sometimes impossible, to achieve complete remineralization.
Excess of bonding material around the attachment base may exacerbate the problem by creating retentive areas where the acidogenic bacteria can adhere and cause demineralization. Complicated appliance designs with loops, auxiliary archwires, springs, coils, and some Class II correctors create noncleanable areas even in highly motivated patients. In most cases, the lesions are small and restricted to forming narrow white bands around the bracket bases or in the areas between the brackets and the gingival margin (Ogaard, 2008). The demineralized enamel is porous and absorbs stains from the daily diet, representing esthetic challenges on the labial surfaces of the affected tooth. In some patients, who are noncompliant with oral hygiene and fluoride regimens, lesion development may be extensive and require premature debonding.
Cariogenic challenge between different orthodontic treatment modalities
On a basic level, the types of orthodontic treatment can be subgrouped into fixed orthodontic treatment and removable orthodontic treatment. In contemporary orthodontics, due to the esthetic demand, the variety of orthodontic appliances has increased, from lingual appliances to a diversity of fixed techniques and clear aligners as a type of a removable appliance. Even though these appliances provide comfort in social life due to less or no visibility than conventional methods, their effects on plaque accumulation and oral flora need detailed investigation.
Significant changes occur in the microbial plaque mass at the metal surfaces and the adjacent tooth surface as early as one week after the insertion of fixed orthodontic appliances (Reichardt et. al., 2019). During orthodontic treatment, the areas that show the highest risk for demineralization are maxillary incisors and mandibular canines and premolars (Hu and Featherstone, 2005).
The use of elastomeric ligatures is frequently blamed for the increase of bacterial plaque around the brackets during fixed orthodontic treatment. Therefore, it is logical to assume that orthodontic brackets that do not need any type of a ligation, namely self‐ligation brackets (SLB), can reduce the amount of bacterial plaque, and thus improve overall oral health. There are several studies that investigated the effects of SLB on oral health and the results of these studies are contradictory (Lucchese et al., 2018). One study showed an increase of S. mutans and Lactobacillus spp. at three months with the use of SLB compared to controls (Baka et al., 2013). Another study showed fewer S. mutans with SLB compared to conventional brackets (Perinetti et al., 2004a). These conflicting results may indicate that the variation in the number of cariogenic bacteria may be a result of the individual’s behavior in terms of oral hygiene measures, and not the type of bracket used.
Lingual orthodontics is also a type of fixed orthodontic treatment technique. Food and plaque deposits on the lingual gingival margin of the teeth are more difficult to remove with standard oral hygiene procedures with respect to the labial side (Lombardo et al., 2013). Bilgin Yener and Polat Ozsoy (2020) measured the amount of biofilm formation on labial and lingual brackets using a quantitative image analysis. Their results showed that the amount of biofilm on debonded brackets was higher than in the labial bracket group. According to some retrospective (Sinclair et al., 1986; Artun, 1987) and prospective studies (Caniklioğlu and Öztürk, 2005; Stamm et al., 2005), wider lingual brackets cause a reduced interbracket distance and make oral hygiene procedures very difficult, leading to an increased risk for plaque accumulation and gingival problems. Therefore, the use of adjunct cleaning aids such as sonic electric toothbrushes, interproximal brushes, chlorhexidine mouthwashes, fluoride mouthwashes, and regular professional cleaning should be recommended for patients with lingual fixed appliances. However, despite the evidence concerning the increased number of cariogenic bacteria in lingual orthodontics, Wiechmann et. al. (2015) showed that the incidence of WSLs was much less in lingual treatment compared to labial treatment. The reason behind this reduction could be the difference in saliva wetting between lingual and labial treatment. Moreover, lingual WSLs do not compromise smile esthetics due to their location on the lingual side of the teeth (Figure 17.6).

Figure 17.6 Caries lesion arising from the lingual bracket on the palatal surface of the first molar.

Figure 17.7 White spot lesions developed during orthodontic treatment.
Since the introduction of commercial aligners in 1998, treatment with orthodontic clear aligners is gaining more and more interest due to its esthetics and ease of use. Since it is a removable type of appliance, patients do not need to carry out extreme oral hygiene measures. However, when the aligners are worn the flow of saliva is limited, the self‐cleansing activities of orofacial soft tissues is interrupted, and the development of plaque is frequently seen under the aligners. Moreover, if patients consume acidic beverages without removing these appliances, WSLs are likely to develop. Traditionally they have been used as retention appliances for years and bacterial adhesion studies showed that the colonies of S. mutans and Lactobacillus spp. start to increase two months after the treatment (Türköz et al., 2012). In a prospective cohort study, Sifakakis et. al. (2018) investigated the salivary levels of cariogenic bacteria S. mutans, Lactobacillus acidophilus, and S. sanguinis among adolescents treated orthodontically with thermoplastic aligners (0.75 mm polyethylenterephthalat‐glycol copolyester [PET‐G] thermoplastic sheets) or self‐ligating fixed appliances. Their results showed no differences in the salivary levels of S. mutans and L. acidophilus between adolescent patients treated for one month with thermoplastic aligners or self‐ligating appliances; however, lower salivary levels of S. sanguinis were found in patients treated with thermoplastic aligners.
Azeem and Ul Hamid (2017) evaluated the incidence of WSLs in patients who had clear aligner treatment using the quantitative light‐induced fluorescence (QLF) method. After a mean of 18 months of orthodontic treatment, the overall WSL incidence was found to be 2.85%, which is a remarkably reduced level compared to that seen after conventional fixed appliances. In addition to this, Albhaisi et. al. (2020) showed that the WSLs that were seen after clear aligner treatment were shallower but larger in area. Remineralization of shallow lesions is more responsive to WSL treatment. The literature needs more research to draw conclusions, but we can say that in patients with adequate oral hygiene habits, clear aligners are superior to fixed appliance techniques in terms of the number of cariogenic bacteria and WSL formation (Figure 17.7).
Evidence‐based strategies against cariogenic activity during orthodontic treatment
The most important prophylactic measure against WSLs is implementation of a good dietary and oral hygiene regimen along with proper tooth brushing with a fluoridated dentifrice. The remineralization process is greatly enhanced by the low levels of fluoride in the saliva and plaque (Ten Cate and van Loveren, 1999). By controlling biofilm accumulation with a fluoride‐containing dentifrice, the lesions can not only be arrested but also be partially repaired (Lima et al., 2008). Dentifrices typically contain sodium fluoride, monofluorophosphate, stannous fluoride, amine fluoride, or a combination of these compounds, but the maximum concentration allowed in the European Union is 0.15%. Ogaard et al. (2004) demonstrated a dose–response effect and a fluoride concentration above 0.1% in dentifrices is recommended for orthodontic patients, because of their increased risk of WSLs. Considering the existing evidence related to caries and fluorosis risks, low‐fluoride (500–550 ppm) dentifrices could be recommended for low caries–risk young children who are at risk of developing dental fluorosis in the permanent maxillary central incisors (less than 3 years of age), especially those living in fluoridated communities. In all other cases, dentifrices containing at least 1000 ppm fluoride should be used (Buzalaf, 2018).
Stannous fluoride, which interferes with the adsorption of plaque bacteria to the enamel surface, offers beneficial effects not only against caries but also against plaque‐induced gingival diseases during orthodontic treatment (Ogaard et al., 1980; Boyd and Chun, 1994). Tin atoms in stannous products block the passage of sucrose into bacterial cells, inhibiting acid production. Ogaard et al. (2006) demonstrated an inhibiting effect of a combined stannous fluoride/amine fluoride toothpaste/mouthrinse against both decalcification and gingival bleeding in a prospective, randomized clinical study. Titanium tetrafluoride solutions, through formation of a retentive, titanium‐rich, glaze‐like surface coating on treated enamel surfaces, inhibit caries lesion development in patients wearing fixed appliances. At a low pH, titanium binds with an oxygen atom of a phosphate group to form Ti‐O‐Ti‐O chains on the tooth surface and this covalently bound titanium covers the tooth surface by formation of a strong complex. This surface coating resists cariogenic challenges even under extreme alkaline and acidic conditions (Büyükyılmaz et al., 1994).
The effect of fluoride is limited when the pH level drops to lower than the solubility product of pure fluoroapatite (pH = 4.5). When this pH is exceeded, the liquid phase of the plaque will be undersaturated with hydroxyapatite and fluoroapatite, making remineralization no longer possible (Larsen, 1990). A dose response to fluoride may not be apparent in this stage and more fluoride may not produce a better clinical effect (Ogaard, 2001). Briefly, if the patient is noncompliant with the implemented hygiene and dietary precautions, the use of a fluoridated dentifrice alone may not be effective in preventing the development of WSLs (O’Reilly and Featherstone, 1987). Supplemental professional sources of fluoride and other antimicrobial agents in the forms of varnishes, solutions, or gels are often recommended. Fluoridated mouthrinses containing 0.05% sodium fluoride used daily have been shown to significantly reduce lesion formation beneath bands. These mouthrinses may be combined with antimicrobial agents such as chlorhexidine (CHX), octenidine dihydrochloride, benzydamine, triclosan, or zinc to improve their cariostatic effect (Kocak et al., 2009). CHX varnishes for long‐term use have been introduced (Ogaard et al., 2001), but are not recommended because of the metallic taste and frequent discoloration they cause to the teeth and tongue.
An in‐office application of a high concentration of fluoride in the form of a varnish or sealant may be beneficial in noncompliant patients. This exposure to fluoride is limited to office‐only application, because of the temporary discoloration of the teeth and the gingival tissues, and, at the same time, increasing expense to the patient and/or chairtime to the clinician (Bishara and Ostby, 2008). With topical fluoride application, a calcium‐fluoride like material (CaF2) builds up in plaque, on the tooth surface (enamel/dentin), or in incipient carious lesions, which acts as a reservoir for fluoride release when the pH is lowered (Ogaard, 1990). Vivaldi‐Rodrigues et al. (2006) found that application of the fluoride varnish resulted in a 44.3% reduction in enamel demineralization in orthodontic patients. The Cochrane systematic reviews have not been able to reach a consensus on any topical preparation or schedule that would provide the greatest benefit for preventing enamel demineralization (Benson et al., 2004, 2019; Derks et al., 2004; Chadwick et al., 2005). In fact, Benson and coworkers recommended daily fluoride mouth rinsing (0.05% NaF) similar to Zachrisson more than 30 years ago (Benson et al., 2004). However, in a more recent Cochrane systematic review it was found that evidence is insufficient to recommend the use of fluoride‐containing materials for attached braces to the teeth or intraoral fluoride‐releasing devices (Benson et al. 2019).
Probiotic approach
The oral microbiome is an essential component of the human microbiome, and it refers to the microorganisms that live in the human oral cavity (Dewhirst et al., 2010). The oral cavity, containing over 700 species, is the second most complex microbiota in the human body, after the colon (Wade, 2013; Palmer, 2014). Bacteria, fungus, viruses, archaea, and protozoa are all part of the oral microbiome. According to the Human Oral Microbiome Database (www.homd.org), roughly 54% have been grown and named, 14% have been farmed but unidentified, and 32% are only known as uncultivated phylotypes. A growing number of studies have shown that the oral microbiota is important in the pathophysiology and development of numerous oral and systemic disorders (Dewhirst et al., 2010; Zhang et al., 2018).
Probiotic organisms are thought to act primarily through the following mechanisms: competition with potential pathogens for nutrients or adhesion sites; killing or inhibiting pathogen growth via the production of bacteriocins or other products; improvement of intestinal barrier integrity and upregulation of mucin production; modulation of cell proliferation and apoptosis; and stimulation and modulation of the mucosal immune system (Teughels et al., 2011). However, probiotics have unique properties in the oral cavity. Oral probiotics should be able to adhere to and colonize oral tissue, particularly hard, nonshedding surfaces, and eventually form a component of the biofilm (Meurman and Stamatova, 2007; Teughels et al., 2011). In addition, they should not digest carbohydrates; otherwise, pH will drop and caries will develop (Teughels et al., 2011).
Probiotic techniques have been examined to cure caries, mostly by interfering with cariogenic pathogen oral colonization. Näse et al. (2001) were the first to test L. rhamnosus GG for caries‐inhibiting capacity in vivo and discovered that the test group had reduced dental cavities and lower S. mutans counts. Bifidobacterium (Çağlar et al., 2005), L. reuteri Bifidobacterium animalis (Cildir et al., 2009), L. paracasei, and L. casei, in addition to L. rhamnosus, have been shown to reduce the quantity of cariogenic bacteria and thereby prevent dental caries. Clinical research conducted in patients undergoing orthodontic treatment showed that daily kefir consumption and use of probiotic toothpaste decreased salivary microbial colonization (Alp and Baka, 2018). In contrast, results of other studies showed that the probiotic bacteria had no significant impact on caries prevalence in tested groups (Taipale et al., 2012; Villavicencio et al., 2018). Nagarajappa et al. (2015) stated that the use of probiotics can reduce S. Mutans colony‐forming unit (CFU) counts, which may have a preventive effect on the development of dental caries; however, the lack of long‐term follow‐up periods makes it impossible to suggest that the effect continues after stopping the bacteriotherapy, or its influence on the prevalence/ incidence of dental caries. This makes the clinician think about sustainability. In addition, it is known that even early administration (i.e. in the first year of life) of probiotics does not represent permanent colonization in the oral cavity (Taipale et al., 2012).
A meta‐analysis of 50 randomized control studies found that present data are insufficient to recommend probiotics for dental caries management (Gruner et al., 2016). The same meta‐analysis stated that probiotic treatment was found to have a favorable effect on gingival inflammatory markers, which is an important issue during orthodontic treatment. Additionally, a number of studies support probiotic therapy to prevent or treat gingivitis and periodontitis. Specifically, there are promising results with L. reuteri on gingivitis and periodontitis (Krasse et al., 2006; Iniesta et al., 2012) and with L. brevis on aggressive periodontitis (Shah et al., 2013). In contrast, a more recent systematic review concluded that probiotic administration does not seem to have an effect on gingival inflammation and enamel decalcification development in patients under treatment with fixed orthodontic appliances. That review pointed out that extrapolating data is very complex due to lack of standards among clinical studies. The authors underlined that more randomized control studies are needed, especially on finding the effective probiotic strains (no effect on inflammation and demineralization; Hadj‐Hmou et al., 2020). In any case, it is crucial to note that dental oral diseases have a complex etiology, which means that lowering cariogenic bacterial levels might help, but does not guarantee disease control. Furthermore, identification of the correct dose, treatment time, and optimal vehicles for a probiotic approach are still under research worldwide.
Other than probiotics, synbiotics have been under research for the last few years. The word “synbiotic” refers to products that contain both probiotics and prebiotics. Following medicine and the food industry, prebiotics are being coupled with probiotics in dentistry to boost the latter’s capacity to “outgrow” infections (Antoniadou and Varzakas, 2021). Only five combinations have been tried to date, and they are all in the preclinical stage (Tester and Al‐Ghazzewi, 2011; Kojima et al., 2016).
Orthodontic treatment commonly alters the possibility of biofilm formation, thus every effort is worth it to change the bacterial community. On the other hand, dental disease is defined as a noncommunicable, bacteria‐related lifestyle disease, so a good diet with less sugar is very valuable in fighting the disease (Pitts et al., 2021; Twetman, 2018).
Novel bracket adhesive protocols
Tooth‐conserving and time‐saving adhesive methods of retaining orthodontic attachments are replacing traditional methods and procedures. However, despite these advances, caries risk under and in the vicinity of multibonded appliances is of concern (Ogaard, 1989). GICs and resin‐modified glass ionomer (RMGI) cements were introduced as orthodontic bonding adhesives to take advantage of some of their sustained fluoride‐release capabilities (Swift, 1989; McCourt et al., 1990), but their bond strength was found to be equivocal while the concentration of fluoride released decreased with time (Miguel et al., 1995; Miller et al., 1996; Bishara et al., 2007). Nowadays, bioactive adhesive systems with antibacterial effects or intensive remineralization ability are considered to be beneficial.
Microleakage, an important postrestorative complication, is defined as the seeping and leaking of fluids and bacteria in the tooth–restoration interface (Gladwin and Bagby, 2004). The concept of microleakage has been underestimated by orthodontists. Formation of WSL at and under the adhesive–enamel interface is often due to microleakage that may be of clinical importance (Arhun et al., 2006a; Arıkan et al., 2006). Expansion and contraction occur when the teeth are subjected to temperature changes by the ingestion of hot or cold foods. The linear thermal coefficients of expansion of enamel and ceramic or metal brackets and the adhesive systems do not match closely. Metal brackets repeatedly contract and expand more than ceramic brackets, enamel, or the adhesive system, producing microgaps between the bracket and the adhesive system, resulting in leakage of oral fluids and bacteria beneath the brackets. However, if microleakage cannot be prevented, inactivation of bacteria will be a direct strategy to prevent development of WSLs. Antimicrobial adhesive systems may be beneficial in such situations, ensuring antimicrobial effect at the site of therapeutic importance, which is the enamel surface under the bracket in this circumstance (Arhun et al., 2006a).
MDPB (12‐methacryloyloxydodecyl‐pyridinium bromide) is incorporated in various restorative materials since it allows immobilization of the antibacterial component by polymerization, halts the deterioration in the mechanical properties of cured resins, and improves inhibitory effects against bacterial growth on surfaces (Imazato, 2009). The use of argon laser for curing composite resins used for orthodontic attachments has been tried as it saves time and alters the enamel structure, rendering it less susceptible to demineralization. Combining laser irradiation with fluoride treatment can have a synergistic effect on acid resistance, thus preventing the formation of WSLs (Hicks et al., 1995).
When the brackets are bonded to the enamel using an adhesive resin, there is often an amount of flash around the enamel–bracket interface that needs to be cleaned before the resin is light cured. Even in the hands of a very careful clinician, the resultant enamel–bracket interface will expose some rough composite surface that may become a critical site for plaque accumulation (Sukontapatipar et al., 2001; Gwinnett and Ceen, 1979). The accumulation of dental plaque around brackets may cause enamel demineralization and possibly WSLs. Attempts have been made to minimize the amount of flash around the brackets to provide a smooth enamel–bracket interface, and one of the latest developments is the creation of a special type of adhesive system that eliminates the removal of flash. In 2015, the APC™ Flash‐Free (FF) Adhesive Coated Appliance System (3M, Maplewood, MN, USA) came onto the market that attempts to eliminate the need for flash removal around the brackets. The brackets incorporate an amount of prepasted adhesive with a nonwoven polypropylene matrix soaked with resin under the individually packed brackets. The nonwoven matrix acts like a sponge during bonding and the excess resin is soaked back into the base before the setting of the resin. Independent studies have investigated the manufacturer’s claims regarding two main strengths of this adhesive system: adequate bond strength and smooth enamel–resin interface. Foersch et al. (2016) investigated the resin margin interface in brackets bonded with the APC FF system. The stereomicroscopic images revealed remarkably few excess composite remains around flash‐free bonded brackets, with an average amount of visible resin between 0.16 and 0.08 mm measured in the bracket periphery. Furthermore, the filleted edge that is formed by the resin microscopically seemed to form a smooth and well‐locking shape in this type of bracket bonding.
Short‐term effects of FF brackets on bacterial plaque, microbial formation, and WSLs were evaluated in a comprehensive in vivo study that was conducted by Yetkiner et al. (2019). The main difference between the conventional bracket and the flash‐free bracket was found to be the variety of bacterial content. The counts of Aggregatibacter actinomycotemcomitans, Porphyromonas gingivalis, and Streptococcus oralis were much lower in the FF group compared to the conventional APC bracket group. Therefore, even though the amount of plaque and number of WSLs were similar for the first three months of the treatment, fewer pathogenic bacteria were found around FF brackets. In another in vivo study, ElSherifa et al. (2020) evaluated enamel roughness and color after debonding APC FF brackets, APC Plus brackets without a flash‐free system, and a conventional adhesive system. Atomic force microscope results revealed less roughness in the FF group and color evaluation with the spectrophotometer showed that the adhesive around FF brackets showed less discoloration compared to the other groups (ElSherifa et al., 2020). When the enamel–resin interface is smooth, less plaque accumulation will be seen and microleakage will also be less. Therefore, the clinician is advised to use advanced bonding materials to obtain a smooth marginal interface between enamel and resin.
Other than recently developed adhesive materials, GICs, especially RMGICs, are still an alternative for orthodontic bonding and banding procedures. In a recent review, Fricker (2022) mentioned the possible advantages of GICs in patients with a high caries risk. GICs have the trait of long‐term fluoride release, which is characterized by an initial high peak that quickly declines to level off over a period of months (Ngo and Opsahl‐Vital, 2014). As fluoride reserves run low, GICs can be recharged with fluoride using fluoride‐containing oral care products such as topical fluoride gels, fluoride‐containing toothpastes, and mouthrinses to keep the fluoride release going (Benington et al., 2001). The bond strengths of both chemically cured and resin‐modified GICs improve over time; however, RMGICs reach maximum tensile strength faster than chemically cured materials (McCarthy and Hondrum, 1994). The mean shear bond strength of RMGIC is consistently lower than that of composite resin, according to in vitro investigations. However, higher adhesion is not necessarily desirable, as excessive bond strengths may cause iatrogenic injury during bracket debonding (Wiltshire and Noble, 2010). When debonding composite resin from hypomineralized enamel, such as WSLs, adhesive failure occurs more frequently at the enamel–resin contact than at the attachment–resin interface of mineralized enamel. When attachments are removed, the risk of injury to the hypomineralized enamel increases (Cochrane et al., 2017).
The clinician can choose the correct material for bonding by considering the properties of the enamel (i.e. AI, hypomineralization, etc.) and caries risk of the patient.
Pulpal reactions to orthodontic forces
The dental pulp is a specialized type of loose connective tissue, rich in cells such as odontoblasts, fibroblasts, and cells of the immune, nervous, and vascular systems (Leone et al., 2009). While performing complex functions such as having a nervous and vascular supply for vitality, carrying sensory impulses, and formation of protective secondary dentin, it is responsive to peripheral impacts, such as orthodontic forces. Morphological and structural changes occur in dental pulps subjected to such forces (Anstendig and Kronman, 1972; Delivanis and Gauer, 1980; Popp et al., 1992; Kayhan et al., 2000). Although reports on these changes present conflicting data, most show degenerative changes that may have harmful effects on tooth vitality.
The pulpal reactions to the orthodontic force include pulp changes like cell damage, inflammation and wound healing, and changes that may lead to permanent damage to the pulp (von Böhl et al., 2012). Circulatory disturbances, vascular compression, and formation of secondary dentin after orthodontic force application have been reported (Stenvik and Mjör, 1970; Anstendig and Kronman, 1972). Radiorespirometric techniques, which measure the tissue respiration rate, indicated depression of the oxygen utilization system within the pulp cells after the application of orthodontic forces (Hamersky et al., 1980; Unsterseher et al., 1987). An average of 27% reduction in pulpal respiration has been seen after three days of orthodontic force application (Unsterseher et al., 1987) and this might compromise the long‐term vitality of the tooth (Popp et al., 1992). Hypoxia in pulp cells due to reduced blood flow may negatively enhance mitochondrial function and proliferative activity (Amemiya et al., 2003). Significant elevation of aspartate aminotransferase (AST) activity, an enzyme that is released into the extracellular environment following cell death, has been demonstrated after one week of intrusive force application (Veberiene et al., 2009). Likewise, Perinetti et al. (2004b) observed elevated AST activity in orthodontically treated teeth and found these levels comparable to reversible pulpitis. It has been hypothesized that increased AST levels could be the result of circular disruption, reduction in oxygen levels, and pulp tissue apoptosis. Furthermore, an increased response to electrical pulp testing was seen, which is positively correlated to anatomical features such as pulpal weight and root surface area (Veberiene et al., 2009).
Several authors have detected the formation of calcified deposits in the dental pulps of teeth undergoing orthodontic treatment (Stenvik and Mjör, 1970; Delivanis and Gauer, 1980; Popp et al., 1992). However, Stenvik and Mjör (1970) concluded that these changes were not different from those in untreated teeth. Loss of tooth vitality during orthodontic treatment has also been reported, especially in previously traumatized teeth. Histologically, cell death can be seen as either cellular necrosis and/or apoptosis. Even though previous studies called the changes in teeth undergoing orthodontic treatment necrosis, the term apoptosis is more suitable for nontraumatized teeth. Rana et al. (2001) investigated apoptotic changes with orthodontic mechanics in detail and could observe the maximum rate after three days of force application. In the long term, dental pulp damage due to orthodontic treatment varied from 2% to 17% for root canal obliteration and from 1% to 14% for pulpal necrosis in adolescents (von Böhl et al, 2012). In a systemic study by Weissheimer et al. (2021), there was shown to be low evidence in the current literature regarding the loss of pulp vitality during the application of orthodontic forces. However, a history of dental trauma may be considered a risk factor for the loss of pulp vitality during orthodontic treatment (Javed et al., 2015).
Several other factors are also involved in pulpal reactions to orthodontic force, the main one being the type of tooth movement. Intrusion, the movement that has the greatest impact on the root apex, is capable of occluding the pulpal blood supply (Vandevska‐Radunovic et al., 1994; Sano et al., 2002). Moreover, Kvinnsland et al. (1989) and Nixon et al. (1993) reported a substantial increase in pulpal blood flow and in the number of capillaries after application of extrusive orthodontic forces. Mostafa et al. (1991) observed vacuolization and severe odontoblastic degeneration in dental pulps undergoing orthodontic extrusion for seven days. McDonald and Pitt Ford (1994) found decreased human blood flow after continuous light tipping forces, using laser Doppler flowmetry. Since orthodontic treatment involves complex three‐dimensional (3D) tooth movements, it is not always possible to avoid movements that are relatively more detrimental.
Since the first rule of orthodontic mechanics is to avoid poorly controlled and jiggling forces (Krishnan and Davidovitch, 2009), the magnitude of the applied force is another important factor. Controlled intraoral orthodontic mechanics do not require heavy forces for tooth movement, but orthopedic treatment requiring forces over 500 g may have deleterious effects on the dental pulp. In a histological study, Küçükkeleş and Okar (1994) reported degeneration of vessel walls in teeth undergoing rapid palatal expansion (RPE) treatment. However, contrary to the results of previous studies, a histomorphometric evaluation by Kayhan et al. (2000) has shown no pulpal differences between control teeth and teeth undergoing RPE. In a systematic review, the authors aimed to identify the optimal safe force levels for the pulp, but the literature showed inconclusive evidence regarding the force level that would cause the minimally detrimental pulp tissue reaction in humans (von Böhl et al., 2012).
The degree of pulpal reaction to orthodontic forces also depends on individual factors such as the age of the patient and the size of the apical foramen (Butcher and Taylor, 1951; Stenvik and Mjör, 1970). Hamersky et al. (1980) reported positive correlations between age and the rate of tissue respiratory depression. Therefore, the maturity of a tooth is an important aspect in preserving tooth vitality during orthodontic treatment, and clinicians should be cautious while moving teeth with closed apices.
As pulpal tissue is susceptible to thermal stress, temperature‐generating orthodontic procedures such as stripping, thermal debonding, and residual adhesive removal after debonding require extra attention. The heat generated during rotary instrumentation may result in irreversible trauma to the pulp. Among the different modalities used for instrumentation, such as a handheld stripper, rotary disks, and an air‐rotor stripping that utilizes diamond burs, the handheld stripper, which does not need any rotary instrument, has been found to be the safest method (Baysal et al., 2007). Thermal debonding using a carbon dioxide laser (Ma et al., 1997) is considered safest for debonding orthodontic brackets. Clean‐up of residual resin using high‐speed handpieces may cause vascular injuries and pulp necrosis. Zach and Cohen (1965) stated that a temperature rise of more than 5.5 °C will result in pulpal inflammation and an increase of 11 °C resulted in necrosis. Uysal et al. (2005) reported that clean‐up of residual resin should be performed with adequate water cooling to avoid temperature changes exceeding the critical value. Eminkahyagil et al. (2006) concluded that tungsten carbide burs used at low speed may be the method of choice for adhesive clean‐up.
Technological advancements in laser dentistry may be useful in the future for avoiding severe pulpal reactions. In a study performed on rats, it has been shown that the use of low‐level laser therapy (LLLT) during tooth movement results in an increase in vascularization and faster repair of pulpal tissue. LLLT has been shown to induce stem cell activity by increasing cell migration, proliferation, and viability, activating protein expression, and inducing the differentiation of progenitor cells (De Villiers et al., 2011). Low‐level laser irradiation promotes the proliferation of mesenchymal and cardiac stem cells in culture (Zaccara et al., 2015). The therapeutic effects of LLLT, such as pain relief, acceleration of tissue healing, repair and neovascularization, increased fibroblast and collagen fiber proliferation, and decrease in the immune response, may provide an insight for optimization of orthodontic treatment (Abi‐Ramia et al., 2010). The preferred dose of LLLT is 600–700 nm when a visible light spectrum is used (Tuby et al., 2007). According to the study by Zaccara et al. (2015), the parameters of LLLT (30 mW power, 660 nm wavelength, and 1.0 J/cm2 energy density) promoted cell proliferation. Laser therapy exerts a dose‐dependent effect on biological responses and seems to have a cumulative effect at each new dose applied (Li et al., 2010).
Despite all these efforts to minimize adverse pulp reactions, it seems almost impossible to avoid hyperemia or reversible pulpitis during the early stages of orthodontic tooth movement. As shown by Popp et al. (1992), the signs of hyperemia diminish as blood flow returns to normal in 72 hours. These mild pulpitis reactions can be detected 2 hours after bonding/banding. Moderate pain caused by pulp hyperemia can last up to at least seven days (Polat and Karaman, 2005). Mild pain can be noted after each appointment due to the change in archwires, but pulp vitality is unaffected in most of these cases.
Differential diagnosis between symptoms of tooth movement and an inflamed tooth is very difficult, especially if there is a history of trauma. A patient with severe pain or pain that lasts for more than 10 days or who has darkening of the crown should consult a restorative specialist, preferably an endodontist. Radiographic evidence of an apical radiolucency may necessitate endodontic therapy (Hamilton and Gutmann, 1999), although orthodontic movement can be continued afterward.
During orthodontic treatment, the color of teeth may darken to gray or brown as a sign of discoloration, necrosis, or devitalization of the pulp. It is a rare phenomenon, but it does present an esthetic problem, especially when seen in an anterior area. It can also be seen during an orthognathic procedure in which an approach is made near the root apex, or direct resection is done, when pulp necrosis and discoloration and pulp diseases can develop (Kim, 2017; Figure 17.8).
Root resorption
Orthodontically induced external (apical) root resorption
Alveolar bone resorption is the basis of orthodontic tooth movement. Bone resorption occurs in a usual healthy periodontal environment, while the highly mineralized cementum is more resistant to the resorption process, because of its fluoride content and vascular nature. Moreover, the cemental surface is covered by a pre‐cemental layer that is resistant to resorption (Reitan, 1974). The inflammatory process, incident and essential for tooth movement, is also a reason for orthodontically induced root resorption (OIRR). When orthodontic forces are applied over a sustained period of time, hyalinization of the compressed periodontal ligament (PDL) may develop rapidly. The defensive leukocytes that migrate out of PDL capillaries include osteoclast progenitors that promptly coalesce to form multinucleated cells, capable of resorbing mineralized tissues (Krishnan, 2017). External apical root resorption (EARR) is initiated when the protective layer of cementoblasts, interfacing with the hyalinized PDL, undergoes apoptosis and enables odontoclasts to resorb cementum and dentine. Initially, a protective layer of cementoid is removed that leaves a raw cemental surface open to odontoclastic attack (Brudvik and Rygh, 1993, 1994a, 1994b). It is usually seen in the apical region. There is a decrease in the hardness and elastic modulus of the cementum from the cervical to the apical region, making the apical areas prone to resorption (Chutimanutskul et al., 2006). When the forces are relieved, repair commences from the periphery, with fibroblast and cementoblast‐like cells synthesizing collagenous fibrillar material and a new PDL. Most of the resorbed lacunae are repaired with secondary cementum (Reitan, 1985). Resorbed acellular cementum is always repaired with cellular cementum (Feller et al., 2016). According to Owman‐Moll and Kurol (1998), 6–7 weeks after the termination of force, the amount of repair rises to 82%.
Root resorption caused by orthodontic therapy occurs in two locations: superficial surface resorption and in the apical third of the root (Figure 17.9). The increased incidence can be explained in two ways (Huang et al., 2005):
- First, the fulcrum of the tooth is more coronal to the apical third of the root and, due to the differences in the direction of the PDL fibers, forces are more traumatic to the apical region than to the coronal.
- Second, the middle and gingival thirds of the root are covered with acellular cementum, whereas the apical third is covered with cellular cementum, containing cells and blood vessels that have been damaged by heavy forces (Rygh, 1977).
Predisposing factors for root resorption can be divided into general factors and local factors (Krishnan, 2017). Among the general factors, the age at the start of orthodontic treatment, sex, ethnicity, systemic diseases and medications, and genetics can be mentioned. Among the local factors, tooth structure and root morphology are the main factors. Treatment and appliance type, amount of tooth displacement, type of tooth movement, duration, degree and amount of orthodontic force, anterior vertical intermaxillary elastics, and application of orthopedic forces were also assumed to be related to root resorption. Extraction treatment was found to be related to an increased rate of EARR, possibly because of the increased apical displacements and the close distance of the apex to the anterior cortical bone area (Sondeijeker et al., 2020; Yassir et al, 2021). However, a major factor for the occurrence of root resorption is individual susceptibility, which is not predictable (Rygh, 1977). The susceptibility to root resorption differs among individuals, as well as within the same individual at different periods of time. Hormones, body type, and the metabolic rate trigger signals that initiate fluctuations in osteoclastic and osteoblastic activities. In certain systemic diseases such as allergy and asthma, there is increased susceptibility to resorption (Davidovitch, 1996; Davidovitch et al., 2000; McNab et al., 1999). The maxillary and mandibular incisors, maxillary first molars, maxillary first and second premolars, and maxillary canines are the teeth that are more prone to root resorption. One reason for the high prevalence of root resorption among incisors may be their thin root morphology, but it is more likely due to the excessive movement during orthodontic treatment – that is, round tripping – and contact with the cortical plates of alveolar bone, particularly during the use of intraslot torque, which generates very heavy forces (De Angelis and Davidovitch, 2009). If there was pretreatment root resorption, the rate might increase from 4% to 77% during treatment (Goldson and Henrikson, 1975). Previously traumatized teeth presented an important risk for OIRR (Linge and Linge, 1991), but Malmgren et al. (1982) concluded that resorption rates do not increase in the presence of trauma if a normal PDL environment is maintained (as a result of applying appropriate mechanics). Although Wickwire et al. (1974) found a greater incidence of resorption during orthodontic treatment in 53 endodontically treated teeth, Spurrier et al. (1990) and Remington et al. (1989) found lower resorption rates in such teeth due to their increased dentin hardness and density after the orthodontic treatment (Figure 17.10). Retreatments were not found to negatively influence the risk of EARR (Sondeijeker et al., 2020).

Figure 17.8 A maxillary incisor that showed discoloration two weeks after bimaxillary orthognathic surgery. Orthodontic force levels were reduced, hoping that regeneration might occur, but the brownish color did not change in eight months. Therefore, at the end of the orthodontic treatment nonvital bleaching was applied.

Figure 17.9 Orthodontically induced apical root resorption in the apical third of the root. (a) At the end of fixed appliance treatment. (b) At one‐year follow‐up. (c) Periapical radiograph at 14‐month follow‐up. Resorption has not progressed. The tooth has been splinted with a flexible wire retainer due to slight mobility.
Fixed appliances have more detrimental effects on teeth than removable appliances (Linge and Linge, 1983). Specific movements like intrusion and torque are more associated with root resorption than the other types of movement. Resorption is also observed in teeth subjected to faulty mechanics in the early stages of treatment, such as in uncontrolled tipping of maxillary incisors in order to reduce excessive overjet (De Angelis and Davidovitch, 2009). According to a systematic review, orthodontic treatment with clear aligners might not avoid root resorption, but both the incidence and severity of resorption could be lower compared with results reported for treatment with fixed appliances (Fang et al., 2019). Pamukçu et al. (2020) found no differences in root resorption rates between nonextraction lingual treatment and labial treatment; however, currently there are no data regarding extraction treatment. The relationship between the length of treatment time and root resorption has been positively correlated by a majority of the studies (Brezniak and Wasserstein 2002; Sameshima and Asgarifar, 2001).

Figure 17.10 Root resorption due to orthodontic treatment. (a, b) The talon cusp of the left central incisor was ground down during orthodontic treatment. Note that only this tooth did not demonstrate apical resorption, as it was nonvital due to pulp/dentin exposure, which was not diagnosed during the grinding procedure. (c, d) Treatment of chronic apical periodontitis with root canal treatment and apical resection. (e) Patient was retreated orthodontically. (f) After the second orthodontic treatment, the left central incisor showed no resorption and was also asymptomatic without any mobility.
The presence of root resorption can be established using conventional radiographic methods, subtraction radiography, cone beam computed tomography (CBCT), scanning electron microscopy, and histopathology (Nanekrungsan et al., 2012). The periapical method, especially with the paralleling technique, seems to be the best conventional method, with minimal distortion and superimposition of anatomical structures. The periapical and panoramic methods provide two‐dimensional (2D) evaluation, but CBCT is the only method that provides 3D evaluation. Dental CBCT offers a lower radiation dose, fewer artefacts, and faster image projection than a conventional CT (Dudic et al., 2009), but is not recommended for this purpose because of the high radiation dose (Sondeijker et al, 2020). Histopathological examination and scanning electron microscopy are clearly not methods that are clinically applicable. Root resorption may also be diagnosed with the help of dentinal proteins such as dentin matrix protein 1 and dentin phosphophoryn, which are released into the gingival crevicular fluid during active root resorption (Mah and Prasad, 2004).
The clinician’s role in minimizing root resorption
The strategies to minimize external root resorption should include identification of systemic and local risk factors at the stage of treatment planning, limitation of treatment duration, the use of light intermittent forces, and biannual radiographic monitoring in order to detect any possible root resorption at the earliest stage (Lim et al., 2011).
Treatment plans for patients with predisposing factors should ensure that teeth will not be moved unnecessarily and into contact with cortical plates of alveolar bone, in order to avoid any additional resorption. The timing of the first radiograph to detect the signs of EARR was proposed as 6–12 months after the initiation of fixed orthodontic treatment, especially in patients with premolar extractions (Sondeijeker et al., 2020). When a sign of EARR is detected, the clinician has to decide whether to continue or to stop orthodontic treatment. If the decision will be for the continuation of the treatment, then the treatment goals can be re‐evaluated and modified, if needed. If any sign of resorption is seen in the radiographs, the application of orthodontic force can be interrupted for at least three months to allow for repair (Reitan, 1964; Levander and Malmgren, 1988; Sondeijeker et al., 2020). After the rest period, caution is required and a follow‐up radiograph after six months might be indicated, but, more importantly, the appliances should be adjusted to deliver the appropriate mechanical forces, and excluding the affected teeth from the appliances may also be an option. If the decision will be for termination of the treatment, especially in cases of generalized resorption, the clinician may be relieved to hear that iatrogenic root resorption usually does not progress once orthodontic force is discontinued.
A tooth with severe resorption and increased mobility can be splinted to the neighboring teeth. The retention appliances should be passive and regular retention check‐ups should be made. The patient should be informed about the situation and advised to be careful in the use of these teeth. During the retention period, the clinician should prevent occlusal trauma to the resorbed teeth through modification in the retainers (Dougherty, 1968). Root canal treatment is usually not needed, but rarely when the pulpal nerve is affected the patient might experience some pain. In rare cases, resorption continues despite termination of orthodontic treatment and does not stop until splinting and endodontic therapy are performed (Gholston and Mattison, 1983). For severe, active lesions, endodontic treatment should be performed with a calcium hydroxide paste, but if the resorption has ceased, the canal can be filled with gutta‐percha. A crown/root ratio of 1 : 1 is sufficient for maintaining the tooth. If the root length is shorter than this, the tooth is likely to be unable to bear the functional loads of mastication. The literature presents some case series and reports, but none of the resorbed teeth was lost during a follow‐up period of 5–25 years (Sondeijeker et al., 2020). However, the patient should be informed that the affected teeth may be lost early due to periodontitis. Although vitality is rarely affected, endodontic treatment should be performed if the crown/root ratio is less than 1 : 1. A search for pharmacological agents capable of repairing root resorption revealed that bisphosphonates (Liu et al., 2004; Sedghizadeh et al., 2021), echistatin (Talic et al., 2006), and low doses of corticosteroids (1 mg/kg; Ashcraft et al., 1992; Demir and Arici, 2021) have positive effects, whereas high doses (15 mg/kg) have a reverse effect.
Invasive cervical root resorption
Invasive cervical resorption (ICR) is a relatively rare and aggressive form of external root resorption that begins with cemental damage just below the epithelial attachment, leading to progressive destruction of tooth structure (Gülşahı, 2014). It usually affects single teeth, but in rare cases multiple teeth are affected (Coyle et al., 2006). Cervical location is the characteristic feature, and resorption of coronal dentin and enamel creates a pinkish color as the vascular tissues become visible (Heithersay, 2004; Figure 17.11). The lesions are characterized by highly vascular fibrous tissue with osteoclasts arising from the PDL (Smidt et al., 2007). Since microorganisms are rarely found, microbial involvement in these lesions is doubtful (Heithersay, 1999; Trope, 2002). A study of 222 patients who displayed ICR showed that orthodontic treatment, trauma, intracoronal bleaching, and surgery involving the cementoenamel junction were the major predisposing factors. In that study, 21.2% of patients were reported to have a history of orthodontic treatment. Diabetes has also been suggested as a predisposing factor, as factors like inflammation and oxidative stress, the activation of clastic cells, and the promotion of angiogenesis are linked to the hypoxic cellular tissue environment (Galler et al., 2021). Regarding the relation to orthodontic treatment as a risk factor for cervical resorption, there are conflicting results in the current literature. In a recent systematic review (Khalaf et al., 2022), two randomized control trials (RCTs) and one cohort study were analyzed to investigate whether orthodontic treatment could be considered as a risk factor for cervical resorption. The RCTs (Dudic et al., 2017; Giannopoulou et al., 2016) showed that both a loss of cervical volume and an increase in the formation of cervical resorption were found in teeth that underwent orthodontic force application. However, in the cohort study by Thönen et al. (2013), 175 patients, who had completed their treatments about eight years before, were recalled for their final orthodontic check and cervical root resorption was found in only one patient after a CBCT evaluation. So orthodontic treatment has the potential to stimulate cervical root resorption, but the literature is still lacking high‐quality studies to conclude a direct relation, instead of an idiopathic cause.

Figure 17.11 (a, b) Invasive cervical resorption after orthodontic treatment, demonstrating a pinkish color. (c) The patient was referred to an experienced endodontic specialist for treatment of invasive cervical resorption by elimination of the actively resorbing tissue and restoration of the defect with a suitable filling material (mineral trioxide aggregate). However, conventional root canal treatment was carried out by a general practitioner and the prognosis remained poor.
ICR is painless and rarely symptomatic. The resorption process starts below the gingival margin and extends apically and coronally along the root dentin (Smidt et al., 2007). The lesion is often separated from the pulp by the predentin layer, which is the last to resorb (Heithersay, 2004). A clinical feature is a pink coronal discoloration close to the gingival margin. It can go unnoticed for months to years and only become evident on routine radiography. Conventional radiographs taken from different angles can identify the involved surfaces, but a 3D visualization of the exact size and location of the lesion requires a small‐field CBCT scan (Kim et al., 2003; Gulsahi et al., 2007; Gulsahi, 2014). CBCT is very useful in diagnosis and treatment planning of ICR. In addition, it is often used alongside conventional imaging to address the extent of the lesion and the prognosis of the affected tooth. Two important features of ICR differentiate it from internal root resorption. One is the absence of pulpal signs and the other is the radiographic appearance of the pulp canal size (Gulabivala and Searson, 1995; Smidt et al., 2007). In ICR the canal space is unchanged, and the radiolucency extends coronally and apically in the dentin (Figure 17.12).
ICR is quite aggressive; therefore, the orthodontist should refer the patient as soon as the lesion is diagnosed. The treatment should be performed by experienced specialists to prevent tooth loss. The rationale for treatment of ICR is elimination of the actively resorbing tissue and restoration of the defect with a suitable filling material (Figure 17.13). Both nonsurgical and surgical treatment approaches can be used. Nonsurgical regimens involve topical application of a 90% aqueous solution of trichloroacetic acid for the induction of coagulation necrosis to the lesion area, curettage, endodontic treatment if pulpal perforation is present, and GIC restoration. The use of calcium hydroxide for neutralization of the lesion, elimination of microorganisms, and inactivation of toxic products is also recommended (Frank, 1981; Estrela and Holland, 2003). If the margin of the lesion is below the cervical line, orthodontic extrusion can be performed. Extrusive orthodontic forces can be applied for 4–6 weeks and during the extrusion crown shortening can be performed if premature contact occurs. Fiberotomy can be performed for maintenance of the extruded tooth (Smidt et al., 2007). The cases with cervical resorption do not usually show ankylosis, but if the healing occurred with replacement resorption with ankylosis, the orthodontic extrusion will be likely to fail (Luso and Luder, 2012; Figure 17.14).

Figure 17.12 The 37‐year‐old patient’s main complaint was rotation of her upper right canine and inadequate restoration space for # 35 (a, c). The internal resorption in # 21 was misdiagnosed at the beginning of the treatment (c). After one year of orthodontic treatment with fixed appliances, the rotations were corrected and adequate space for proper restoration of # 35 was obtained (b, d). At the end of the orthodontic treatment, the internal resorption of # 21 was diagnosed and it was decided to follow the patient to understand the prognosis. Follow‐up periapical radiographs were taken after two years (e) and four years (f), and cone beam computed tomography scans were obtained six years after completion of the treatment (g).
Surgical treatment involves periodontal flap surgery, curettage, and restoration of the defect. Restorative materials that have been used previously include amalgam, composite resin, and GIC (Lustmann and Ehrlich, 1974; Frank, 1981; Frank and Blakland, 1987). Currently, MTA is recommended to restore the resorptive cementum in cervical resorption cases (Frank and Torabinejad, 1998
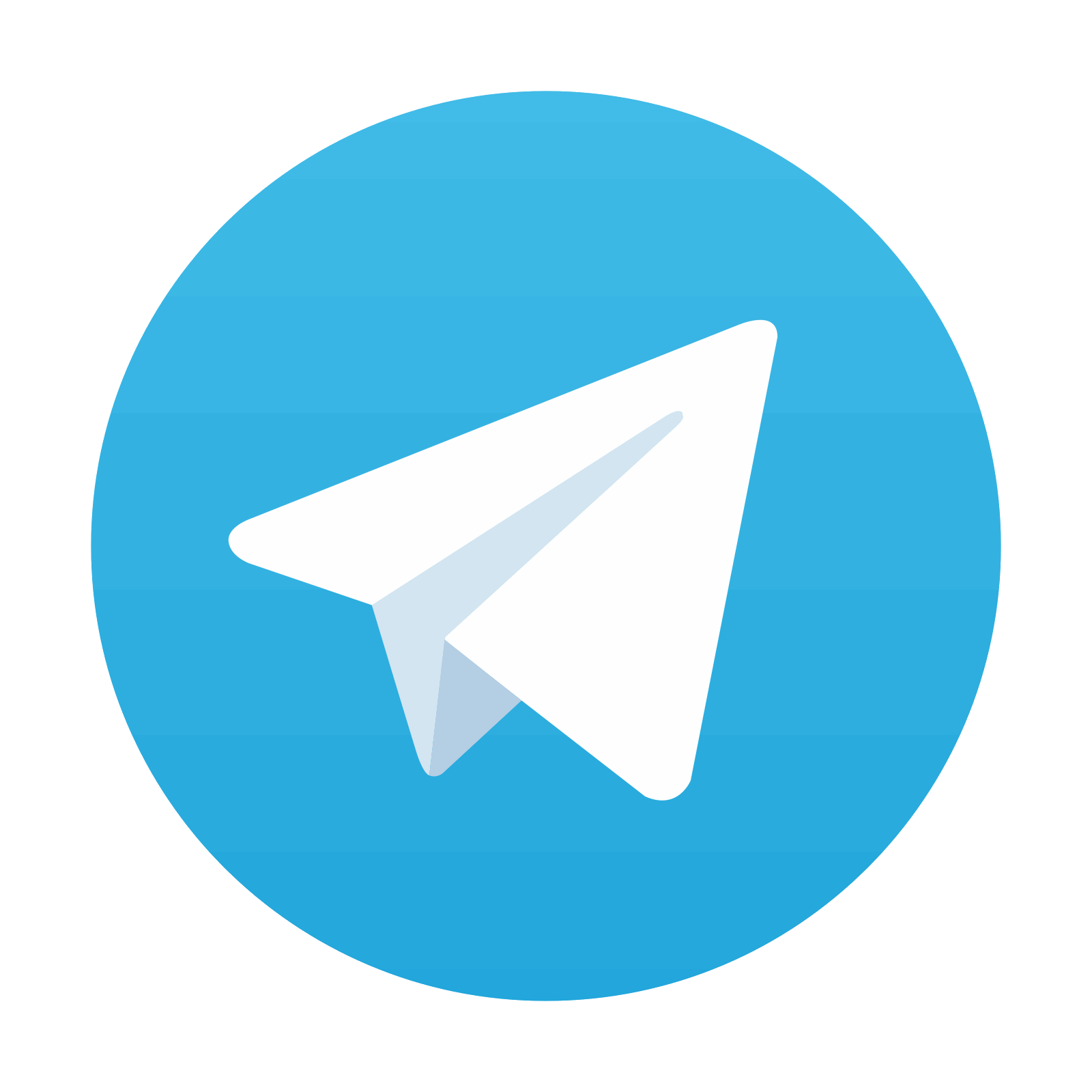
Stay updated, free dental videos. Join our Telegram channel

VIDEdental - Online dental courses
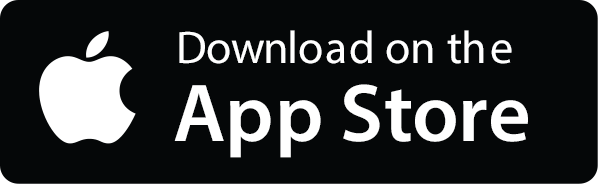
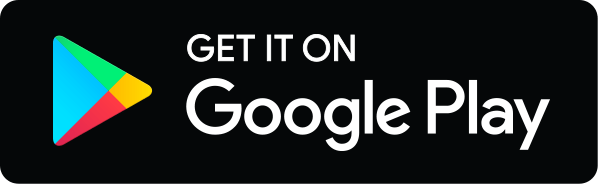