Abstract
Objectives
To measure the ‘repair’ strength of various combinations of composite using four manufacturers’ adhesive systems, to compare the bond strengths with the cohesive strength of the original, unrepaired products and to assess whether the chemical nature of the resin matrix influenced the repair strength.
Methods
Specimens were prepared of three composite materials Durafill, Heraeus Kulzer; P90 (Silorane) 3M ESPE; Z250 (3M ESPE) and aged in water at 60 °C for 1 month. One surface of each specimen was faced with 80-grit silicone carbide paper, one of four adhesives placed (Ecusit, DMG; Clearfil Repair, Kuraray; P90 System Adhesive; Single Bond 2, 3 M ESPE) and ‘repair’ composite added of the same type as above, such that all combinations of original and repair composite and adhesive were used. ‘Stick’ samples, approximately 6 mm × 0.8 mm × 4 mm were prepared from each repair specimen, a neck created at the junction of original and repair composites and the hour-glass sample tested in tension at 1 mm/min. The microtensile bond strength of the repair was calculated and the mode of failure (adhesive; cohesive in the original composite; cohesive in the repair composite) recorded.
Results
There was no significant difference between the cohesive strengths of Filtek P90 and Filtek Z250 (both ≈106 MPa); both were significantly stronger than Durafill (67.0 MPa). For bonding to Durafill the bond strengths ranged from 17.6 MPa to 50.9 MPa; for bonding to P90, the bond strengths ranged from 5.0 MPa to 54.2 MPa; for bonding to Z250, the bond strengths ranged from 17.2 MPa to 75.4 MPa. Clearfil Repair appeared to provide the most consistently high bond strengths, followed by the P90 System Adhesive, Single Bond 2 and Ecusit. Overall, the majority of failures (74%) was adhesive.
Significance
It appears that bonding of new dimethacrylate-based composite to old dimethacrylate-based composite can be a viable clinical procedure. However, if the original composite is silorane-based (e.g., P90), then using the silane-based adhesive may be the best repair option, and similarly if it is planned to effect a repair with a silorane-based composite, using a silane-based adhesive may give the best outcome. The null hypotheses are thus rejected.
1
Introduction
Resin-based composite (‘composite’) restorations have a finite lifetime, depending on a range of factors . The traditional approach to a failed composite restoration is removal and replacement, however each time this is done, the cavity becomes larger, the tooth weaker, more complex restorations may result and pulpal symptoms may ensue . It has been estimated that half a general practitioner’s time is spent on ‘replacement’ dentistry, with the consequent high cost in time and money .
Consistent with the philosophy of ‘minimum intervention’ operative dentistry, defective restorations should be first evaluated for the possibility of repair, rather than being routinely replaced . There is now accumulating evidence that repair of composite can be a viable, long-term, clinical procedure . However, a recent questionnaire to 20 composite manufacturers revealed that all recommended repair of their materials, but more than half (65%) did not supply any repair instructions. The remainder (35%) gave guidance on repair using the same type and brand of composite as the original, i.e., that manufacturer’s own brand. The dilemma for the clinician, however, is that the type and brand of original composite may not be known, and now that composite monomers other than dimethacrylates are being used which require matching bonding agents (e.g., ‘acid’ resins in the polyacid-modified composites; siloranes), the repair task becomes less straightforward. It is also apparent that different repair techniques are being taught in different dental schools around the world .
Adhesive technology in dentistry is a complex area , and since 2003, several reports have been published on the repair bond strength of composite materials . The main area of interest in such papers was in the surface treatment of the original composite, including mechanical roughening, acid ‘etching’, silanating and applying various resin-based adhesives, and recently excellent reviews have been published . Most authors ‘repaired’ the original composite with the same composite, and all composites used were dimethacrylate-based. However, it should be noted that the bond strengths do not necessarily correlate with microleakage, at least in the laboratory . Regarding bond strength and clinical data, one recent review showed that in non-carious cervical lesions, ‘aged’ bond strength values and medium-term retention rates show a reasonable correlation. However, no data appear to exist on the correlation between bond strength of repaired resin composites and clinical performance.
The aim of this study was to evaluate the ‘repair’ strength of various combinations of composite, including a silorane-based material, using four manufacturers’ adhesive systems, and to compare the bond strengths with the cohesive strength of the original, unrepaired products. The null hypotheses were (a) the type of adhesive used did not influence the repair strength, (b) the chemical nature of the resin matrix did not influence the repair strength.
2
Materials and methods
Twelve disc-shaped specimens, 12 mm diameter × 3 mm thick, were prepared for each composite material ( Table 1 ) in a plastics mold. The mold was positioned on a glass microscope slide, the composite was injected, a second microscope slide pressed firmly onto the composite to remove excess, the composite photocured for 40 s (XL3000; 3M, St Paul, MN; minimum intensity 600 mW cm −2 ) and stored in water at 60 °C.
Name Batch number, shade |
Manufacturer | Composition |
---|---|---|
Durafill VS Original: 010028, A3.5 Repair: 010213, A2 |
Heraeus Kulzer GmbH, Hanau, Germany | Urethane dimethacrylate, 52% w/w silicon dioxide , splinter polymer. Total filler 75% (w/w) |
Filtek P90 (Silorane) Original: N122784, A2 Repair: N115352, C2 |
3M ESPE, St Paul, MN | Silane treated quartz 60–70%, yttrium trifluoride 5–15% Bis-3,4-epoxycyclohexylethyl-phenyl-methylsilane 5–15% 3,4-Epoxycyclohexylcyclo-polymethylsiloxane 5–15% |
Filtek Z250 Original: 9TC, A3.5 Repair: N099907, A2 |
3M ESPE, St Paul, MN | Silane treated ceramic 75–85%, bisphenol a polyethylene glycol diether dimethacrylates 5–10%, diurethane dimethacrylate 5–10%, bisphenol diglycidyl ether dimethacrylate (BISMA) 1–10%, triethylene glycol dimethacrylate (TEGDMA) <5% |
After 1 month the specimens of each material were removed from the water, the upper face ground unidirectionally with 80-grit carborundum paper and divided randomly into four groups of three specimens, one group being assigned to each of the repair adhesives ( Table 2 ). For each group of three, one specimen was randomly assigned to each of the repair composites ( Table 1 ).
Name Batch number |
Manufacturer | Composition |
---|---|---|
Adper Single Bond 2 | 3M ESPE, St Paul, MN | |
Scotchbond Etch 4CL | Phosphoric acid 35% | |
Adhesive N104096 | Ethyl alcohol 25–35%, bisphenol a diglycidyl ether dimethacrylate 10–20%, silane treated silica (nanofiller) 10–20%, 2-hydroxyethyl methacrylate 5–15%, glycerol 1,3-dimethacrylate 5–10%, copolymer of acrylic and itaconic acids 5–10%, diurethane dimethacrylates 1–5% | |
Clearfil Repair | Kuraray Medical Inc, Okayama, Japan | |
K-Etchant Gel 00450B | Phosphoric acid 40–60%, colloidal silica, water | |
SE Bond Primer 00887A | 2-Hydroxyethyl methacrylate 20–30%, 10-methacryloyloxydecyl dihydrogen phosphate, hydrophilic aliphatic dimethacrylates, dl -camphorquinone, water | |
Porcelain Bond Activator 00234A | 3-Trimethoxysilylpropyl methacrylate 40–60%, hydrophobic aromatic dimethacrylates | |
SE Bond Bond 01306A | 2-Hydroxyethyl methacrylate 25–35%, bisphenol A diglycidylmethacrylate, 10-methacryloyloxydecyl dihydrogen phosphate, hydrophobic aliphatic dimethacrylates, colloidal silica, dl -camphorquinone | |
Ecusit Composite Repair 628845 | DMG GmbH, Hamburg, Germany | Aliphatic, aromatic dimethacrylate, carboxylic methacrylate |
P90 System Adhesive Self-etch Primer: N109581 Bond: N098774 |
3M ESPE AG, Seefeld, Germany | 2-Hydroxyethyl methacrylate (HEMA) 15–25%, bisphenol A diglycidyl ether dimethacrylate (BISGMA) 15–25%, water 10–15, ethanol 10–15%, phosphoric acid-methacryloxy-hexyl esters mixture 5–15%, silane treated silica 8–12%, 1,6-hexanediol dimethacrylates 5–10%, copolymer of acrylic and itaconic acids < 5, (dimethylamino) ethyl methacrylate <5%, dl -camphorquinone <3%, phosphine oxide <3% |
The specimen of each sub-group was placed in the base of a 6-mm deep × 12-mm internal diameter plastics mold with the 80-grit abraded surface uppermost, the abraded surface treated with one of the adhesives ( Table 2 ) according to the manufacturer’s instructions ( Table 3 ) and the remaining 3-mm deep cavity in the mold filled with one of the repair composites ( Table 1 ). The composite was photocured as above as for the original composite.
Adhesive | Procedure |
---|---|
Adper Single Bond 2 | Apply Scotchbond etchant 15 s; wash 10 s; blow dry; apply Adhesive 15 s with agitation; air thin 5 s; photocure 15 s; apply composite |
Clearfil Repair | Apply K-Etchant Gel 5 s; wash; dry; mix 1 drop of SE Bond Primer with 1 drop Porcelain Bond Activator; mix; apply 5 s; dry; apply SE Bond Bond; blow thin; photocure; apply composite |
Ecusit Composite Repair | Apply Ecusit; work into surface 30 s; air thin; photocure 20 s; apply composite |
P90 System Adhesive | Apply Self-etch Primer 15 s; blow thin; photocure 10 s; apply Bond; blow thin; photocure 10 s; apply composite |
The specimens were placed in tap water at 37 °C for 24 h, removed and fixed by the end to a 12-mm diameter acrylic stub for mounting in a diamond disc cutting device (Isomet; Beuhler; Lake Bluff, IL). Four arcs of the cylindrical specimen were removed to leave a square configuration of face approximately 10 mm × 10 mm, followed by a cut half way across the face to full specimen depth, followed by seven to eight evenly spaced cuts to full depth at right angles to the initial cut. The resulting 14–16 ‘sticks’ of material, approximately 6 mm × 0.8 mm × 4 mm, were fractured away from the mounting base. An approximately 1-mm wide semicircular ‘neck’ was created on both sides of the stick at the level of the original and repair composite interface using a 1.2-mm diameter high speed diamond bur with water cooling. The thickness of the specimens was measured to 0.01 mm at the level of the neck, the hour-glass specimens attached to a sliding jig ( Figs. 1 and 2 ) with cyanoacrylate adhesive and tested in tension at a cross head speed of 1 mm/min. After fracture, the failure load (N) was recorded, the mode of failure assessed at between 30 and 45 magnification under a binocular light microscope (ML9300, Meiji, Japan) and the width of the fracture site measured to 0.01 mm. Specimens that failed prior to testing were assigned a nominal failure load of 1 N.


One disc-shaped specimen, 12 mm diameter × 6 mm thick, was prepared for each composite material in a plastic mold using the procedure described above, photocured from both sides for 40 s and stored in water at 60 °C for 24 h. 14–16 ‘sticks’ of each material, with a semicircular ‘neck’ at the middle, were prepared as above for microtensile cohesive strength testing.
The data were entered into a statistical package (SPSS for Windows; SPSS Inc, Chicago, IL) and the fracture surface area (width at fracture × thickness at fracture, sq mm) and failure stress (failure load/fracture surface area, N/sq mm = MPa) calculated for each specimen. For each group of specimens, the mean failure stress was calculated. The 36 groups were divided into three groups of 12 depending on the original composite (Durafill, P90 or Z250), and the various means within each group of 12 compared using 1-way analysis of variance and LSD t -tests.
2
Materials and methods
Twelve disc-shaped specimens, 12 mm diameter × 3 mm thick, were prepared for each composite material ( Table 1 ) in a plastics mold. The mold was positioned on a glass microscope slide, the composite was injected, a second microscope slide pressed firmly onto the composite to remove excess, the composite photocured for 40 s (XL3000; 3M, St Paul, MN; minimum intensity 600 mW cm −2 ) and stored in water at 60 °C.
Name Batch number, shade |
Manufacturer | Composition |
---|---|---|
Durafill VS Original: 010028, A3.5 Repair: 010213, A2 |
Heraeus Kulzer GmbH, Hanau, Germany | Urethane dimethacrylate, 52% w/w silicon dioxide , splinter polymer. Total filler 75% (w/w) |
Filtek P90 (Silorane) Original: N122784, A2 Repair: N115352, C2 |
3M ESPE, St Paul, MN | Silane treated quartz 60–70%, yttrium trifluoride 5–15% Bis-3,4-epoxycyclohexylethyl-phenyl-methylsilane 5–15% 3,4-Epoxycyclohexylcyclo-polymethylsiloxane 5–15% |
Filtek Z250 Original: 9TC, A3.5 Repair: N099907, A2 |
3M ESPE, St Paul, MN | Silane treated ceramic 75–85%, bisphenol a polyethylene glycol diether dimethacrylates 5–10%, diurethane dimethacrylate 5–10%, bisphenol diglycidyl ether dimethacrylate (BISMA) 1–10%, triethylene glycol dimethacrylate (TEGDMA) <5% |
After 1 month the specimens of each material were removed from the water, the upper face ground unidirectionally with 80-grit carborundum paper and divided randomly into four groups of three specimens, one group being assigned to each of the repair adhesives ( Table 2 ). For each group of three, one specimen was randomly assigned to each of the repair composites ( Table 1 ).
Name Batch number |
Manufacturer | Composition |
---|---|---|
Adper Single Bond 2 | 3M ESPE, St Paul, MN | |
Scotchbond Etch 4CL | Phosphoric acid 35% | |
Adhesive N104096 | Ethyl alcohol 25–35%, bisphenol a diglycidyl ether dimethacrylate 10–20%, silane treated silica (nanofiller) 10–20%, 2-hydroxyethyl methacrylate 5–15%, glycerol 1,3-dimethacrylate 5–10%, copolymer of acrylic and itaconic acids 5–10%, diurethane dimethacrylates 1–5% | |
Clearfil Repair | Kuraray Medical Inc, Okayama, Japan | |
K-Etchant Gel 00450B | Phosphoric acid 40–60%, colloidal silica, water | |
SE Bond Primer 00887A | 2-Hydroxyethyl methacrylate 20–30%, 10-methacryloyloxydecyl dihydrogen phosphate, hydrophilic aliphatic dimethacrylates, dl -camphorquinone, water | |
Porcelain Bond Activator 00234A | 3-Trimethoxysilylpropyl methacrylate 40–60%, hydrophobic aromatic dimethacrylates | |
SE Bond Bond 01306A | 2-Hydroxyethyl methacrylate 25–35%, bisphenol A diglycidylmethacrylate, 10-methacryloyloxydecyl dihydrogen phosphate, hydrophobic aliphatic dimethacrylates, colloidal silica, dl -camphorquinone | |
Ecusit Composite Repair 628845 | DMG GmbH, Hamburg, Germany | Aliphatic, aromatic dimethacrylate, carboxylic methacrylate |
P90 System Adhesive Self-etch Primer: N109581 Bond: N098774 |
3M ESPE AG, Seefeld, Germany | 2-Hydroxyethyl methacrylate (HEMA) 15–25%, bisphenol A diglycidyl ether dimethacrylate (BISGMA) 15–25%, water 10–15, ethanol 10–15%, phosphoric acid-methacryloxy-hexyl esters mixture 5–15%, silane treated silica 8–12%, 1,6-hexanediol dimethacrylates 5–10%, copolymer of acrylic and itaconic acids < 5, (dimethylamino) ethyl methacrylate <5%, dl -camphorquinone <3%, phosphine oxide <3% |
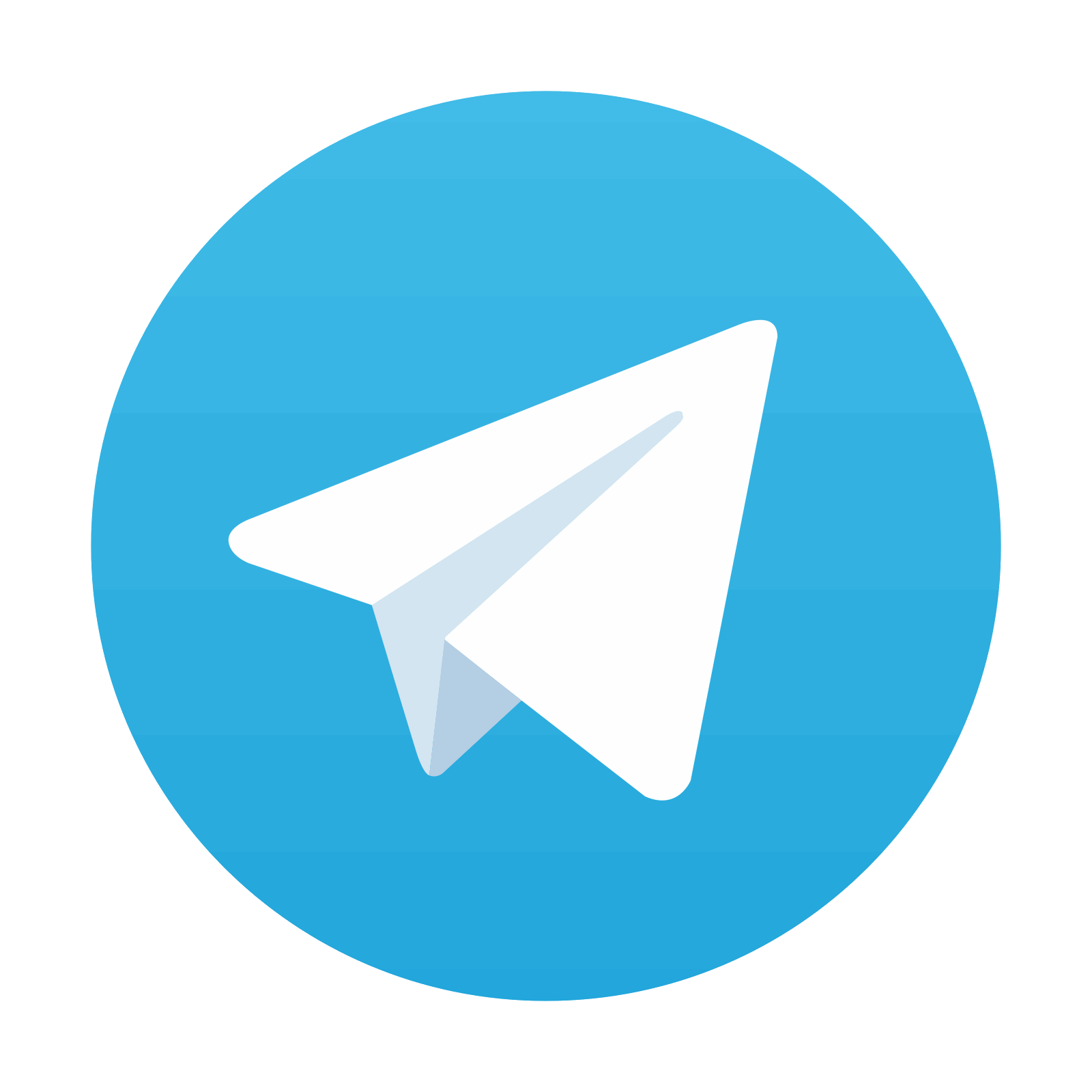
Stay updated, free dental videos. Join our Telegram channel

VIDEdental - Online dental courses
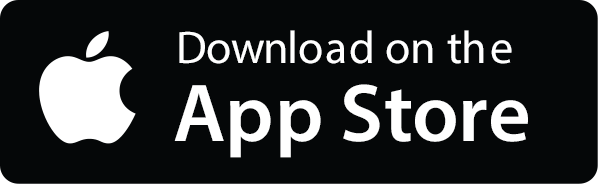
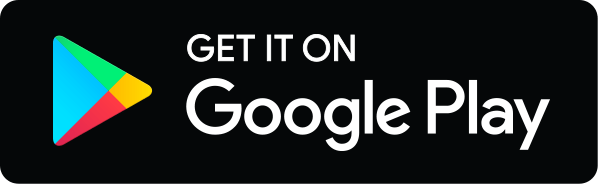
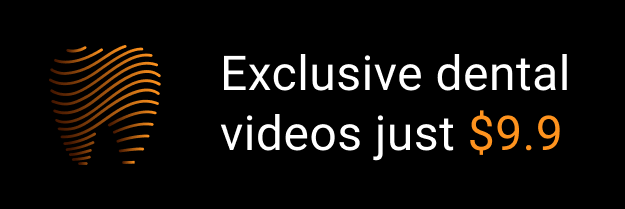