Abstract
This study was conducted to evaluate the healing of critical-size surgical defects after implantation of porous nano-hydroxyapatite/polyamide composite (nHA/PA) blocks based on a bilateral mandible model using adult New Zealand white rabbits. 15 rabbits were divided randomly into three groups according to the observation period: 4, 12 and 24 weeks. The defects on one side were implanted with nHA/PA blocks and the contralateral defects were kept empty as blank controls. A combination of macroscopic, radiographic, histological and histomorphometric studies were performed up to 24 weeks postoperatively and compared with normal healing. Large amounts of callus and active osteoblasts were found in the pore structure after 4 weeks of implantation, and the defects were completely occupied by neo-bone with density comparable with that of host bone at 24 weeks. Significant difference was found between nHA/PA groups and blank controls regarding X-ray opacity over the whole period and bone parameters at 4 weeks postoperation ( P < 0.05). The porous nHA/PA composite promotes bone formation over the extension of the defect, particularly in the early stage. Porous nHA/PA offers interesting potential for maxillofacial reconstructive procedures in load-free areas.
The ideal bone-graft substitute should be an osteogenic, biocompatible, bioresorbable, easy to use material with specific properties that provide structural support and deliver a prescribed dosage of drugs for a stipulated time . It is rare to find all these features in one material and composite materials seem to be the most suitable for clinical applications.
Hydroxyapatite (HA) has a composition and structure very close to natural bone mineral. HA can promote the formation of bone-like apatite on its surface , and is used in various biomedical fields. Polymers combined with HA can promote osteoblast adhesion, migration, differentiation and proliferation , which have potential applications in bone repair and regeneration. Polyamide (PA) has good biocompatibility with various human cells and tissues, probably because of its similarity to collagen protein in chemical structure and active groups . PA also has excellent mechanical and corrosion-resisting properties resulting from the strong hydrogen bonds between the amide groups in PA macromolecules . Particularly, as a polar polymer with high polarity, PA has a relatively high affinity to, and may form hydrogen bonds with, nano-sized apatite . The biocompatibility and bioactivity of HA/PA composites has been confirmed in previous in vitro and in vivo studies .
Bioactive materials with interconnected porosity in their structure have added advantages in hard tissue prosthesis . The porous structure of bioactive materials supports tissue in/on growth and supplements implant stability by biological fixation. The disadvantages of porous materials result from their low fracture strength and fatigue resistance. These materials could be used successfully in load-free situations or as coating on metal substrates .
In the present investigation, nano-hydroxyapatite/polyamide composite (nHA/PA) with high interconnecting porosity (65–75%) was tested as a bone substitute material in rabbit mandible defects. The biocompatibility of dense nHA/PA composite is excellent . Porous nHA/PA was expected to promote bone repair in load-free maxillofacial defects. The purpose of this experiment was to evaluate the biocompatibility and osteogenesis of porous nHA/PA66 in vivo using a rabbit bilateral mandible defect (critical-size) model . The relevant part of the mandible was collected 4, 12 and 24 weeks after surgery, to investigate bone healing by macroscopic, radiographic and histologic analysis.
Materials and methods
Nanocomposite characterization
The rectangular nHA/PA blocks (14 mm × 9 mm × 3 mm) were of high interconnecting porosity (65–75%); the pore size ranged from 300 to 500 μm ( Fig. 1 ). The compressive strength for the block specimens was 3–7 MPa, similar to that of natural cancellous bone (2–15 MPa) . The porous struts were initially pasteurized using distilled water and subsequently sterilized by gamma radiation (0.6 × 10 4 Gy, 6 min) prior to implantation.

Surgery
15 New Zealand white rabbits weighing about 3.5 kg were randomly divided into 3 groups of 5 animals each according to the observation period: 4, 12 and 24 weeks. Surgery was carried out under aseptic conditions and sedation by intravenous injection of 3% pentobarbital sodium (0.03 g/kg). A 2 cm incision was made approximately 1 cm lower to the lower edge of the mandible body to expose the bone. A bicortical critical-size defect (15 mm × 10 mm) was drilled at low speed under continuous saline irrigation on both sides of the mandible body in all animals ( Fig. 2 ) . The periosteum was removed during surgery to exclude the interference of periosteum osteogenesis. The nHA/PA blocks were inserted into the defects on one side of the mandible defect and the defects on the other side were kept empty. Implant placement was randomized to right and left sides and was secured in position by suturing muscle, subcutaneous tissue and skin in layers. All animals received intramuscular injection of penicillin 10,000 units per day immediately after surgery and continued for 3 days. The animals were killed by a lethal dose of barbiturate after a healing period of 4, 12 or 24 weeks. The mandibles were removed, cleaned of soft tissues and prepared for radiographic and histologic testing.

Local inflammatory reaction and would healing
Food intake, weight bearing, bone repair in terms of palpable callus, swelling, hematoma and associated signs of local inflammatory reactions were observed from the day of operation up to postoperative week 24.
Radiological examination
Radiographic examinations were performed immediately after surgery and after 4, 12 and 24 weeks using a dental digital X-ray unit (Siemens Heliodent, Siemens, Erlangen, Germany). The following parameters were standardized for all radiographs: projection distance of 10 cm, projection angle of 90° and exposure condition of 0.06 s at 4 mA. The degree of new bone formation during the healing period was estimated using the grey scale which is displayed automatically by the digital X-ray imaging system.
Histological study
Block samples were removed by cutting the mandible bodies about 8 mm away from the margin of the defects with a diamond saw. Decalcified sections of the bone samples were prepared to determine the status of the bone growth. The bone samples were washed thoroughly with normal saline and were placed in 10% formalin for 7 days. After initial fixation, the samples were dehydrated in a graded series of alcohol until the absolute was reached. They were decalcified and embedded in epoxy resin. Cross-sections of 5 μm thickness were cut at 200 μm intervals using a LEICA 2500E diamond saw microtome (Leica SpA, Milan, Italy) and were stained with Trichrome-masson. Three stained sections for each sample were analysed using a light microscope (NICON E 600) and a digital camera for the relative distribution of neo-bone and fibrous tissue.
Fluorescent labelling and histomorphometric analysis
To determine bone formation rates, undecalcified specimens were created in separate animals. Three rabbits in each group were injected subcutaneously with tetracycline and calcein blue at a dose rate of 25 mg/kg and 5 mg/kg body weight, respectively (11 and 3 days, respectively, before death). Immediately after execution, the mandibles were removed and placed in 70% alcohol for 48 h. After initial fixation, mandibles were dissected free of soft tissue. The bone including the defect site was cut away perpendicular to the occlusal plane for making subsequent transverse serial sections. During the next 14 days, the specimens were dehydrated in graded ethanol and acetone, and then embedded individually in epoxy resin. Cross-sections, 10 μm thick, were made from the embedded bones.
Three pieces from the undecalcified sections of each sample were measured for fluorescent labels using an ultraviolet light microscope (LEICA DMI6000 B) equipped with a video camera. The images were transferred to the computer for automatic image analysis. The distance between the double labels (DDL) was measured under 400× magnification. Mean osteoid seam width (MOSW) was obtained by dividing the total osteoid volume by its absolute linear extent. The mineral apposition rate (MAR) was calculated by dividing the width of the double labels by 28, 84 or 168 days described. Mineralization lag time (MLT) was calculated by dividing the mean osteoid seam width by mineral apposition rate (i.e. MLT = MOSW/MAR) .
Statistical analysis
Intragroup and intergroup differences between treatment types and days of healing, were compared using paired t test or one-way ANOVA and SNK test (SPSS11.0). Differences were considered significant at P values <0.05.
Results
Local inflammatory reactions and wound healing
There were no marked signs of inflammation or rejection during the postoperative period. Food intake and weight bearing in each animal gradually improved within 10 days. Wound healing was uneventful in all cases and sutures were removed on the tenth postoperative day.
Macroscopic observations
At the conditioned time period, the mandibles were removed and macroscopically observed. The bone defects were healing well, and no necrosis or obvious inflammation was detected in any animal.
At 4 weeks, macroscopic observations revealed that the implant was stable in the defects and the extent of callus formation was so high that almost 70% of the implant surface was covered. Only a few calluses were found in the control group ( Fig. 3 ). At 12 weeks, firm organized callus covered almost the whole surface of the implant and the profile of the mandible edge was basically recovered, indicating an advance stage of bone repair ( Fig. 3 b). In the blank control, the callus increased but there were fewer than in the experimental group. A broad, curvilinear defect on the edge of the mandibular defect was observed in the control group. At 24 weeks postoperatively, the defect in the nHA/PA group was osteointegrated with the natural bone and the edge of the defect was indiscernible ( Fig. 3 c). In the control group, the edge of the mandible was almost restored, but the covering callus was thin and the margins of the defect were visible ( Fig. 3 c′).

Radiological evaluation
At 4 weeks post-surgery, the implants were more radiodense than they were immediately after implantation, and the margins of the defect sites were blurred, suggesting mineralization around the implant ( Fig. 4 ). The radiopacity derived from the control group was evident despite small areas of mineralization at circumferential areas of the defects. At 12 weeks, mineralization progressed continuously in both groups. In the experimental group, bone trabecula networks were detected and the defect area was nearly completely bridged. In the control group, a broad, curvilinear impression on the edge of the mandible was visible in the defect area. At 24 weeks, bone trabecula networks in the experimental group were well organized and the bone density was similar to that of host bone. For the blank controls, the radiographs showed substantial reduction in the defect size, the presence of dent at the defect site indicating that the defect had not been completely filled in by the host bone, and a critical-size model for bone construction .

The apparent bone mineral density, measured by X-ray grey scale, revealed a significant difference between the experimental and control groups over the whole observation period ( P < 0.05) ( Fig. 5 ).

Histological studies
Histological analyses were conducted using plastic-embedded decalcified sections stained with Trichrome-masson. 4 weeks after nHA/PA implantation, the defect sites were filled with newly formed bone and connective tissue ( Fig. 6 a) . Direct bone bonding was observed in various areas at the interface. Large amounts of osteoid tissue and a line of cuboidal-shaped active osteoblasts were seen in the pore structure, indicating that the three-dimensional interconnected pore structure can induce the ingrowth of bone tissue. Osteoclast infiltration was observed on the surface of the implant, which contributed to the bioresorption of the material. In the control group, the cavity was occupied with loose fibro-collagen tissue and no bone formation was observed ( Fig. 6 a′).
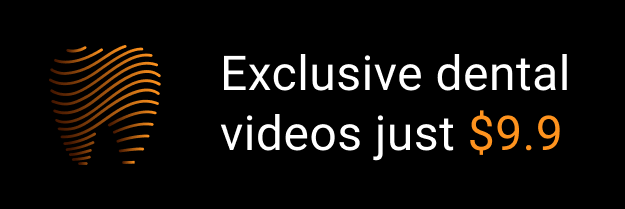