Abstract
Objectives
This study evaluated the relationship between mass changes and hygroscopic dimensional changes during water sorption/desorption cycles in new resin composites.
Methods
A silorane posterior composite (Filtek ® Silorane, FS), two micro-fine hybrid composites (GC Gradia Direct Anterior, GDA; GC Gradia Direct Posterior, GDP), a universal composite (GC Kalore, GCK), and a self-adhering flowable composite (Vertise ® Flow, VF) were evaluated. 25 ( n = 5) disk specimens (15 mm × 2 mm) were prepared according to ISO 4049. Water sorption was measured gravimetrically. Hygroscopic expansions were measured by a laser micrometer regularly during 150 d water storage and 40 d recondition periods, all at 37 °C. Data were analyzed by repeated measures ANOVA, one-way ANOVA and Tukey’s post hoc test ( p < 0.05).
Results
Mass changes after 150 d water immersion ranged from 0.68% (±0.02) for FS to 2.83% (±0.11) for VF and the corresponding hygroscopic expansions were from 0.74% (±0.05) for FS to 4.79% (±0.18) for VF. The differences were significant for all materials ( p < 0.001), except mass changes between GCK and GDP, as well as hygroscopic changes between GCK and GDA. The relationship between hygroscopic expansions and mass changes was initially non-linear and then tended toward linear behavior. But at the late stage of water sorption the hygroscopic expansion of GCK and VF was slightly sigmoidal with respect to their mass changes.
Significance
Relationships between hygroscopic expansion and mass change were determined for some diverse resin-matrix composites. The initial non-linearity for all materials suggests a lower expansion rate due to occupancy of internal free volume by water ingress. The silorane composite FS showed statistically the lowest mass change and hygroscopic dimensional change.
1
Introduction
Esthetic restorations consisting of resin composites continuously encounter a wet intra-oral environment. Thus, during clinical service, these materials may experience water-related interactions, including: elution of residual monomers or other leachable components , degradation of bonds in the polymer networks or at resin-filler interfaces , uptake of water molecules into materials . Such water sorption has been established as a diffusion-controlled process . During this process, ‘unbound’ water molecules diffuse into the matrix and, at the meso-scale, progressively occupy free volume. During that initial phase, hygroscopic expansion and plasticization may occur to a lesser extent than at later stages when free volume has been fully occupied. However, ‘bound’ water molecules, produced by their interaction with polymer chains through hydrogen bonds, can disrupt inter-chain hydrogen bonding, and facilitate absorption of more water molecules. This may cause hygroscopic expansion and plasticization and these time-dependent effects might weaken mechanical properties and thus increase wear rate .
On the other hand, a potentially beneficial effect of hygroscopic expansion is that it might compensate for polymerization shrinkage . However, some marginal adaption measurements indicate that polymerization shrinkage cannot always be compensated by hygroscopic expansion . Conversely, there seems to be stronger recent evidence that in less hydrophobic restoratives hygroscopic expansion can be greater than the shrinkage and thus cause expansion stress, which may induce micro-cracks or even cracked cusps in the restored tooth .
There are, in principle, two possible types (A and B) of plot, which are nevertheless quite different, relating hygroscopic expansion and water uptake ( Fig. 2 a and b). Type A – produced for each individual composite – presents a ‘correlation over time’ between its expansion and water uptake ( Fig. 2 a), whilst Type B involves data for a set of composites and correlates the ‘maximum measured’ expansion and water uptake for each member of the set ( Fig. 2 b). Linear regressions of Type A, between the dimensional change and amount of water sorption, for six self-curing restorative resin composites were reported by Hirasawa et al. . However, they only presented 7 d data, which now would be an insufficient measurement period for some contemporary resin composites. A linear dependence with Type B plots (first cycle: R 2 = 0.98, second cycle: R 2 = 0.998) between percentage absorbed water and volumetric increase was reported for light-cured dimethacrylate resins . Another study showed that hygroscopic expansion was strongly correlated with water sorption ( R 2 = 0.98) in two compomers, one giomer and one resin composite . However, essentially no correlation (Pearson correlation coefficient = 0.1675) was found between maximum water sorption and hygroscopic expansion for a set of six light-cured posterior resin composites . This poor correlation might due to the diversity of materials. Also many differences in specimen size and shape were reported in different studies. Consequently, the relationship between hygroscopic dimensional changes and mass uptake for some contemporary resin composites invites further study. These composites incorporate some varied structural features, including: a silorane-based matrix, a high molecular mass and long matrix monomer DX-511 and a self-adhering monomer. This paper investigates the exact functional relationship between hygroscopic expansion and water uptake over 150 d for each of these composites, via Type A plots, during water sorption/desorption cycles.


The null hypothesis was that: the functional dependence of hygroscopic expansion on water uptake was completely linear over the full range of water uptake for all materials investigated.
2
Materials and methods
Five commercial materials were chosen to represent a range of restorative resin composites ( Table 1 ).
Code | Material | Manufacturer | Lot no./shade | Filler loading (Wt %) | Fillers | Organic matrix |
---|---|---|---|---|---|---|
FS | Filtek ® Silorane | 3M ESPE, St. Paul, MN, USA | 20080514 A3 |
76 | Quarz (silane layer), Radiopaque Yttrium fluoride | Silorane |
GDA | GC Gradia ® Direct Anterior | GC Dental Products Corp., Tokyo, Japan | 0901134 A3 |
73 | Silica, Prepolymerized filler | UDMA and dimethacrylate co-monomers |
GDP | GC Gradia ® Direct Posterior | GC Dental Products Corp., Tokyo, Japan | 0905201 A3 |
77 | Silica, Prepolymerized filler, Fluoro-alumino-silicate glass | UDMA and dimethacrylate co-monomers |
GCK | GC Kalore | GC America Inc., USA | 0903171 A3 |
82 | Prepolymerized filler (with Lanthanoid Fluoride), Fluoro-alumino-silicate glass, Strontium/Barium glass, Silicon dioxide, Lanthanoid fluoride | DX-511, UDMA and dimethacrylate co-monomers |
VF | Vertise ® Flow | Kerr Corporation, Orange, CA, USA | 3172311 A2 |
70 | Prepolymerized filler, Barium glass, Nano-sized colloidal silica, Nano-sized Ytterbium fluoride | GPDM, HEMA and methacrylate co-monomers |
2.1
Specimen preparation
Twenty-five ( n = 5) disk-shaped specimens, (15.0 ± 0.1) mm in diameter by (2.0 ± 0.1) mm in thickness, were prepared according to International Standard ISO FDIS 4049: 1999 and the manufacturer’s instructions. In a laboratory environment at 23 (±1) °C and relative humidity of 50 (±2)%, uncured materials were carefully dispensed into a brass ring mold and clamped between two glass slides covered by two pieces of polyester film. Then the apparatus were exposed to a halogen curing light with 11 mm exit window (Optilux 501, Kerr Corporation, USA), using a standard curing mode (output wavelength range: 400–505 nm; output irradiance: 580–700 mW/cm 2 ). Specimens were carefully removed and any with visual defects were excluded. Any excess material was removed by grinding with 1000 grit silicon carbide abrasive paper until visually smooth. The specimens were stored in a lightproof desiccator at (37 ± 1) °C for 22 h, and then transferred to another desiccator at (23 ± 1) °C for 2 h. Each specimen was weighed by a calibrated analytical balance with a precision of 0.01 mg. The completion of polymerization and dehydration was reached when the mass change of each specimen was within 0.1 mg in a 24 h period. This recorded constant mass ( m 1 ) was the initial mass. Perspex control specimen disks were used (Polymethylmethacrylate, Imperial Chemical Industries, UK).
2.2
Water sorption/desorption
The specimens were randomly placed in 10 mL de-ionized water at (37 ± 1) °C in individual glass vials and were weighed as a function of different immersion intervals until equilibrium was reached by 150 d. At different time points, each specimen was carefully dried by filter paper, weighed to ±0.01 mg, and returned to fresh water storage. The recorded mass was noted m 2 ( t ). Then the specimen was reconditioned in the above desiccators over a 40 d period until constant mass was obtained. Similarly, the recorded mass at different desorption time points was noted m 3 ( t ). The percentage apparent mass change during water sorption – M s (%) and desorption – M d (%) was calculated by:
2.3
Hygroscopic dimensional changes
In parallel with the measurement of water sorption, the hygroscopic expansion of the same specimens was monitored by a laser scan micrometer (LSM) described previously . This measures the size of each disk specimen rapidly (<30 s) over multiple diameters to a resolution of 200 nm. The diametral value presented for each disk at each time point was an overall average of 445 data values. The grand mean dimension of the five disks per group was obtained at each sorption time point.
The initial diameter of each specimen was measured and noted d 1 . The diameter measured at time t during water sorption was recorded as d 2 ( t ) and the corresponding diameter during water desorption was noted d 3 ( t ). The percentage diametral change of water sorption – d s (%) and desorption – d d (%) were given by:
d s ( % ) = d 2 ( t ) − d 1 d 1 × 100
d d ( % ) = d 3 ( t ) − d 1 d 1 × 100
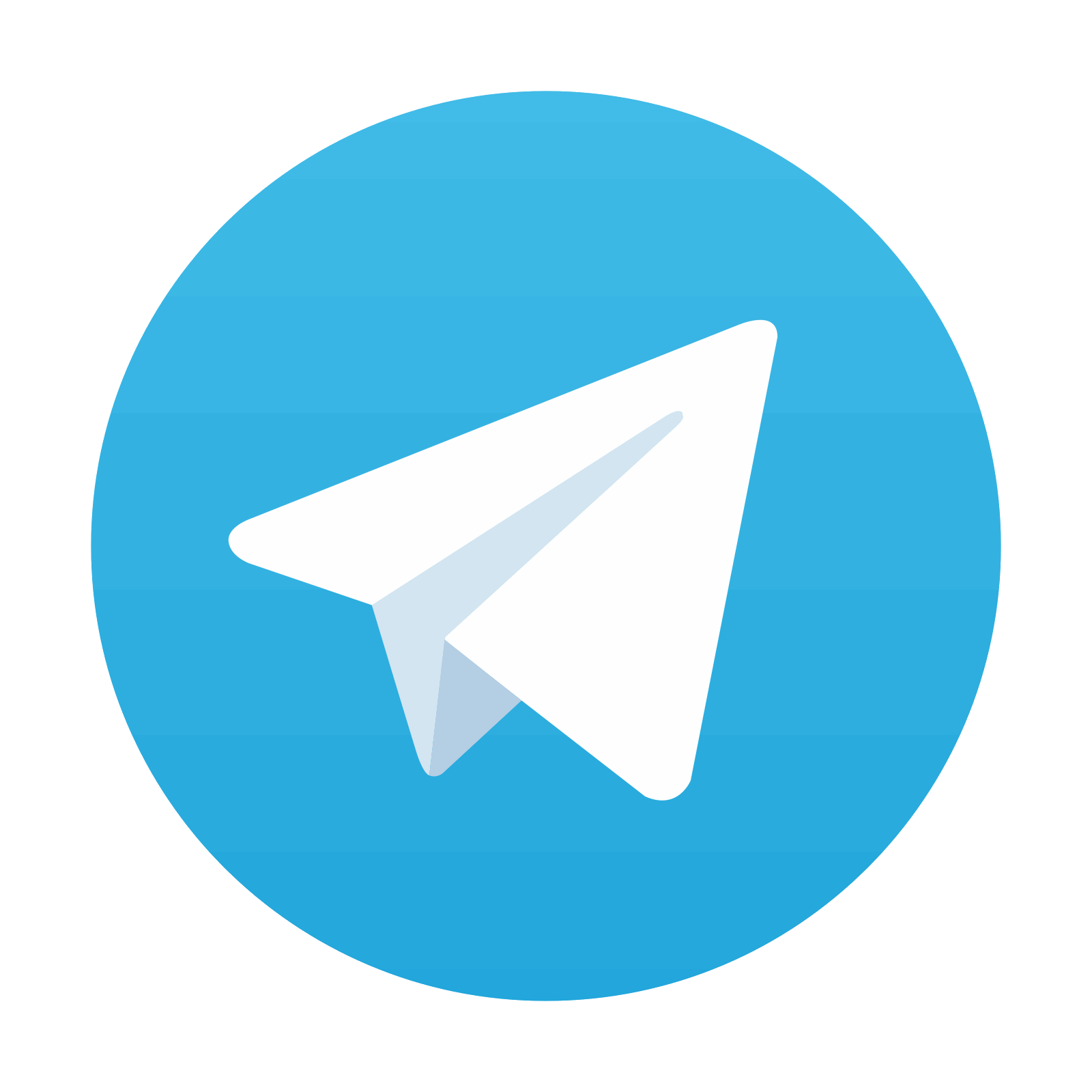
Stay updated, free dental videos. Join our Telegram channel

VIDEdental - Online dental courses
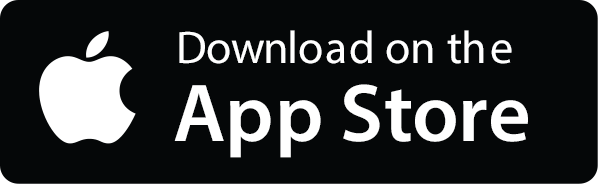
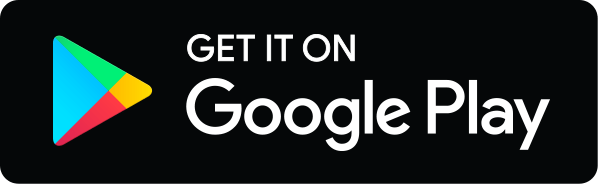