The integration and control of metabolism
Publisher Summary
This chapter discusses the integration and control of metabolism. The main pathways of carbohydrate, fat, and protein metabolism, that is, of the major sources of energy, are described in terms of the enzymes responsible for them. However, the bodies of vertebrates contain about a hundred different types of cell each with a distinctive enzyme pattern. Some of the differences involve tissue-specific enzymes, but many are differences in the amounts and sensitivity of particular enzymes within the metabolic pathways. Not every tissue can burn all types of fuel. Tissues that depend on glucose as a major energy source include red- and white-blood corpuscles, brain, retina, renal medulla, intestinal mucosa, and skeletal muscle in severe exercise. Liver, kidney cortex, heart muscle, and skeletal muscle, except in severe exercise, obtain most of their energy from the oxidation of fatty acids, while the brain and renal cortex, as well as both heart and skeletal muscle, can utilize ketone bodies. The necessary control and integration of energy and other types of metabolism within and between the tissues is brought about by the action of hormones that, in conjunction with the nervous system, ensure that the cells of the body exist in a controlled environment of more or less constant composition.
The metabolic characteristics of some individual tissues
The liver
< ?xml:namespace prefix = "mml" />

Liver hexokinase is similar to the hexokinases in other tissues and has a low Km for glucose (0·01−0.1 mM) but glucokinase which catalyses the same reaction has a much higher Km for glucose (). The concentration of glucose in the blood is normally of the order of 3−7 mM, and glucose equilibrates rapidly across the liver cell membrane. Hexokinase is therefore normally operating at its maximum rate which is relatively low in liver. The presence of glucokinase with its high Km allows the liver cell to respond to an increased concentration of blood glucose by increasing the rate of glucose phosphorylation (Figure 23.1) and hence of overall glucose metabolism.
Muscle
A further feature of muscles is the presence of appreciable amounts of myoglobin which acts as an auxiliary source of oxygen (page 373). The muscles of diving mammals such as seals, whales and dolphins and the flight muscles of birds owe their deep red colour to myoglobin, which in Man is present in significant amounts only in heart muscle.
Protein breakdown and amino acid metabolism are also of importance in muscle, and the major nitrogen-containing products are glutamine and alanine, which are released by the tissue. The pathways of gluconeogenesis and fatty acid synthesis do not exist in muscle. Heart muscle differs from skeletal muscle in that at high work loads it can use the lactate released by skeletal muscle as an energy source. Pyruvate produced by glycolysis in the heart is not converted to lactate. Heart lactate dehydrogenase is inhibited by high concentrations of pyruvate while lactate dehydrogenase from skeletal muscle is not (page 230).
The kidney
The main function of the kidney is to regulate the composition of the body fluids and a pathway of particular importance in this respect is the conversion of glutamine to ammonia and glucose. This conversion is involved in acid/base homeostasis (page 395), and is greatly stimulated in metabolic acidosis. The kidney uses fatty acids as a major energy source and much of the ATP produced from their oxidation is utilized in the active transport of metabolites. The kidney is the only tissue other than the liver which is capable of gluconeogenesis, and, under certain conditions, kidney gluconeogenesis can make a significant contribution to the overall glucose requirements.
The adjustment of tissue metabolism to different physiological states
Absorptive state
During the period immediately after eating a meal, synthetic and storage processes predominate. Under these conditions, the major pathways operating in the liver are the synthesis of glycogen and fatty acids from glucose and the conversion of the fatty acids into triglycerides (Figure 23.2). The amino acids which are not needed for protein and other synthetic processes cannot be stored and are catabolized. The nitrogen atoms derived from the amino groups are excreted as urea whereas the carbon skeletons are mostly oxidized.
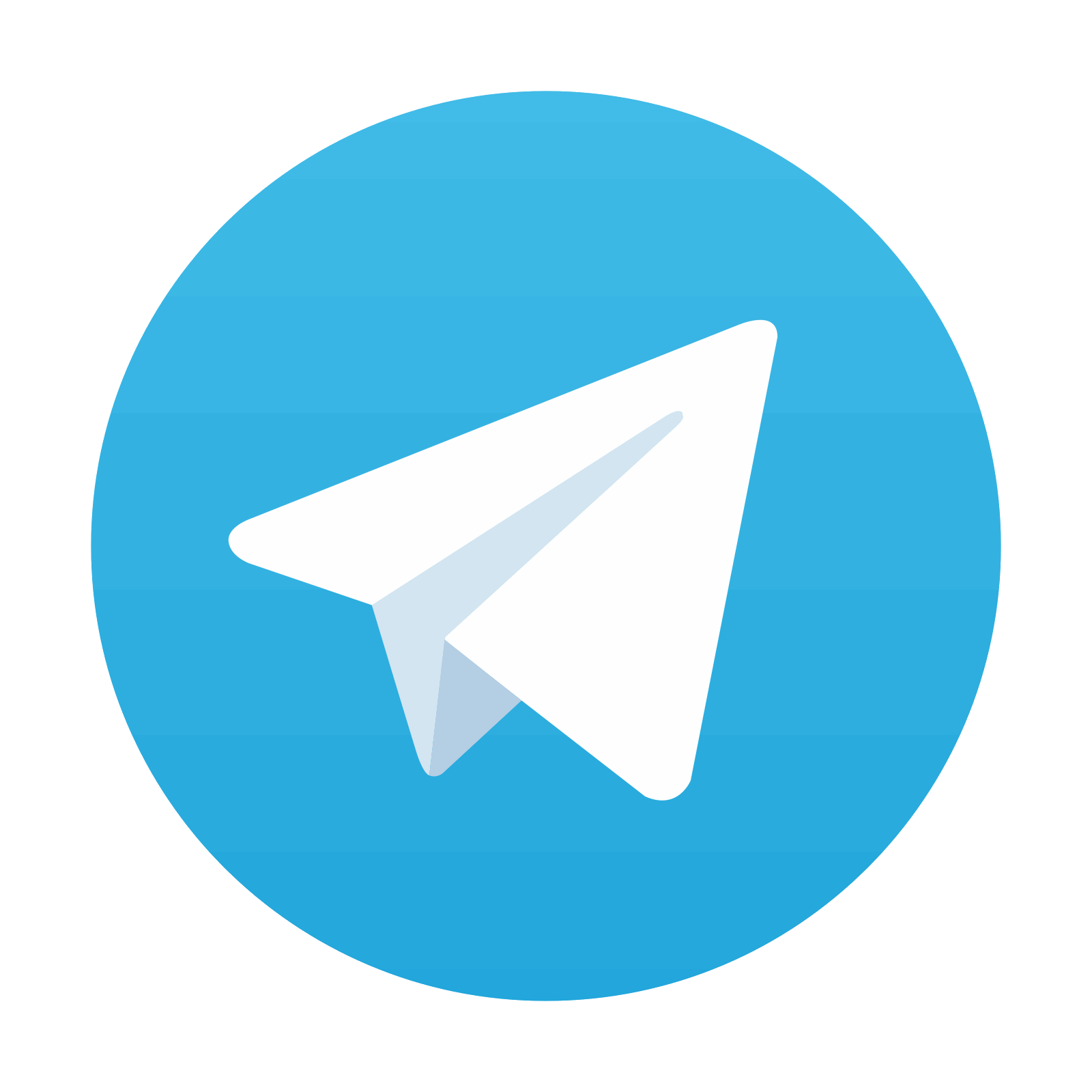
Stay updated, free dental videos. Join our Telegram channel

VIDEdental - Online dental courses
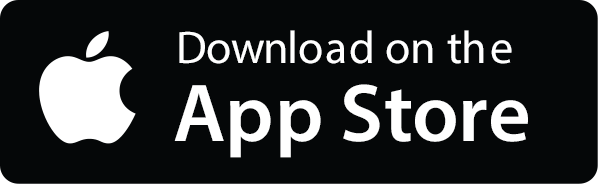
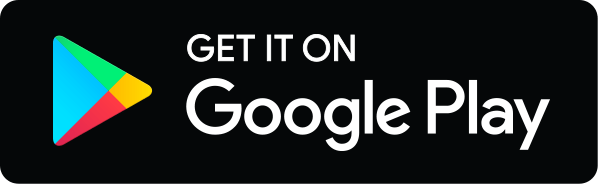