Graphical abstract

Highlights
- •
C(T) shows be a reliable indicator of the zirconia/cement bond quality.
- •
R a , R sk and area variation were evaluated by AFM.
- •
Weibull analysis evaluates the structural integrity of the adhesion zone.
- •
SIE resulted in higher K IC values with great Weibull survivor probability.
- •
Remained alumina debris negatively affected the interface fracture toughness.
Abstract
Objective
Consider the efficacy of glass infiltration etching (SIE) treatment as a procedure to modify the zirconia surface resulting in higher interfacial fracture toughness.
Methods
Y-TZP was subjected to 5 different surface treatments conditions consisting of no treatment (G1), SIE followed by hydrofluoric acid treatment (G2), heat treated at 750 °C (G3), hydrofluoric acid treated (G4) and airborne-particle abrasion with alumina particles (G5). The effect of surface treatment on roughness was evaluated by Atomic Force Microscopy providing three different parameters: R a , R sk and surface area variation. The ceramic/resin cement interface was analyzed by Fracture Mechanics K I test with failure mode determined by fractographic analysis. Weibull’s analysis was also performed to evaluate the structural integrity of the adhesion zone.
Results
G2 and G4 specimens showed very similar, and high R a values but different surface area variation (33% for G2 and 13% for G4) and they presented the highest fracture toughness (K IC ). Weibull’s analysis showed G2 (SIE) tendency to exhibit higher K IC values than the other groups but with more data scatter and a higher early failure probability than G4 specimens.
Significance
Selective glass infiltration etching surface treatment was effective in modifying the zirconia surface roughness, increasing the bonding area and hence the mechanical imbrications at the zirconia/resin cement interface resulting in higher fracture toughness (K IC ) values with higher K IC values obtained when failure probability above 20% was expected (Weibull’s distribution) among all the experimental groups.
1
Introduction
The preferential use of ceramics for fixed dental prostheses is widespread in dentistry today. All-ceramic (metal-free) fixed dental prostheses (FDPs) are considered a reliable alternative material to metal-ceramic FDPs in daily dental clinical practice. Besides its aesthetic quality – perfectly capable of substituting the dental substrate – dental ceramics also have high chemical stability, biocompatibility and superior mechanical properties. These characteristics result in great longevity of all-ceramic dental prosthesis and make its use in dentistry more advantageous over other tooth colored materials, such as composite resins and ceromers .
Zirconia (zirconium dioxide) is used as a biomaterial, for hip replacement, since the late 1960s. Currently, dental researchers focus more often on zirconia stabilized by yttria (yttrium oxide), which forms at room temperature Tetragonal Zirconia Polycrystal (Y-TZP) and presents excellent mechanical properties as its high elastic modulus, around 200 GPa, flexural strength in the range of 700–1400 MPa and fracture toughness of 4.4–6.5 MPa √m.
The Y-TZP high fracture toughness is related to a martensitic type phase transformation from tetragonal to monoclinic when subjected to mechanical stress, for example. This phase transformation is accompanied by very large shear strain (16%) and volume increase (4%) which generates a field of compressive stresses around the propagating crack tip that may difficult or even prevent crack propagation .
On the other hand, the zirconia surface is highly chemically inert, thus usual ceramics etching techniques, that enroll the use of 10% hydrofluoric acid gel followed by silane coupling agent, do not promote a proper adhesion at Y-TZP substrates. For this reason, establishing a strong and stable bond with resin composite cements has proven to be difficult without a proper zirconia surface treatment . Therefore, one of the biggest challenges in dentistry, regarding the use of Y-TZP, is the effective adhesion of this ceramic to the resin cement used for luting prostheses with limited or no mechanical retention.
Alternatively to the usual etching technique, several new methods that intend to improve the bonding of Y-TZP ceramics have been investigated, such as alteration of the surface topography by roughening ; chemical bonding promotion through silica coating deposition (silica coating) using or not zirconate silane agents and use of plasma .
Nowadays, Y-TZP surface air-abrasion with refined alumina (aluminum oxide) particles at a moderate pressure in combination with the use of primers and/or luting resins containing phosphate monomers, as phosphate ester MDP (10-metacriloiloxidecil dihydrogen phosphate), can be considered the state-of-the-art methodology of bonding to zirconia . According to Kern recent review article, air-abrasion in combination with MDP provides clinical evidence of long term durable bonding to zirconia. However, despite the many laboratory shear and tensile bond strength data, only a few clinical trials are available in academic literature and so, the relation to its interface bonding durability under function still requires further investigation.
The use of airborne particles still raises two main concerns: its possible tetragonal to monoclinic phase transformation inducement and the controversial effect on the zirconia strength, since some authors have attributed a zirconia strengthening effect to the airborne and others have reported a strength reduction effect related to the possible creation of surface microcracks . However, according to recent literature the clinically relevant effects on ceramic strength can be avoided if a moderate airborne pressure is used.
In order to obtain an alternative form to create a rougher and more chemically active zirconia surface, Aboushelib et al. have proposed to perform a selective glass infiltration (SIE) with promising results in terms of bond strength to resin cements. The SIE technique demonstrates better bond strength than airborne particle abrasion method even after two years of aging in laboratorial study . Despite the increased bond strength found by the authors, they did not perform a roughness study of the surface formed after the SIE treatment.
Casucci et al. , used atomic force microscopy (AFM) to measure the surface roughness of the zirconia surface treated with SIE. However, these authors only evaluated the arithmetical surface roughness (R a ) alone, not correlating it with other roughness parameters. Furthermore, there was no investigation, either about the role of glass in roughness formation or the effect of the ultrasonic bath with hydrofluoric acid (methodology to remove glass in the SIE treatment) on the zirconia surface.
A more complete analysis of the surface relief after SIE, that includes not only the arithmetical roughness value but also the calculation of the bond area increase and a 3rd order parameter analysis (R sk ) is necessary and therefore became the objective of the AFM analysis performed in the present study.
In addition to evaluating the relief changes as a function of surface treatment, this study also aims to evaluate the bond effectiveness of the interface 3Y-TZP/resin cement after selective glass infiltration treatment (SIE).
Papia et al. have investigated the bond strength of SIE treated zirconia to resin cements, but all investigations have quantified the nominal mechanical strength (microtensile or shear tests) of the bond interfaces. The nominal mechanical strength tests are only a valid way to evaluate the bond strength, if they are performed under a homogeneous stress state. Unfortunately, this situation hardly occurs during the mechanical tests previously described in the literature.
Scherrer et al. , have already shown that the nominal tensile/microtensile test does not allow stress concentrations at the adhesive interface. In addition to this non-homogeneous stress state, the microtensile test specimens are difficult to obtain – they often detach before the test – and they have a great variability both in the location of the crack origin and in the mode of failure even when the same adhesive interface is observed. These findings often invalidate the results, since they corroborate the test dependence on the specimens’ geometry and loading rate, and make the microtensile strength values (μMTS) impossible to be compared among different studies.
An alternative to the use of the nominal strength as a measure of the interfacial resistance can be the interfacial fracture toughness (K IC ), which is an intrinsic property of an interface associated to its ability to resist crack propagation, and consequently, interfacial debonding . For this reason, it is an adequate way of measuring interfacial bond quality.
Some authors suggest that the interfacial fracture toughness tests have a greater discriminative capacity than other tests, like microtensile. It can detect differences between surface preparation methods, not detected by other tests, and also allow stress concentration at the interface in contrast with the nominal strength approach.
The compact tension (C(T)) test piece is suggested for K IC test of dental materials because its geometry requires smaller specimens to attain the plane strain condition necessary to perform the test properly. Furthermore, its geometry facilitates the surface treatments and the luting procedure when compared to other specimen’s geometries.
Traditionally, the C(T) specimen is used for K IC evaluation in dentistry for resilient materials like dentin and resin composites. It has not been used yet for testing zirconia/cement interfaces .
The analysis of the Weibull’s distribution together with mode of failure and fractographic analysis are suggested by Della Bona et al. , as important tools to evaluate the structural integrity of the adhesive zone.
The Weibull’s analysis of an interface property, like fracture toughness, provides either the Weibull’s modulus of the distribution, which gives an indication of the reliability of the bond strength, or the probability of failure, that can be taken as an indicator of bond quality leading to predictions of clinical performance limits, independently, of the mean fracture toughness values .
This paper aims to apply the C(T) test to correlate interfacial fracture toughness (K IC ) of zirconia/resin cement composite interface with Y-TZP surface topography parameters, measured by AFM, because of zirconia surface treatment. Moreover, the study is intended to give a contribution to the understanding of the role of each step of SIE surface treatment in developing the optimum Y-TZP surface topography for the best zirconia/resin cement bond quality.
2
Experimental procedure
2.1
Specimen preparation
For the mechanical test, machinable CAD/CAM partially sintered blocks of Y-TZP (Ceramill Zi, Amann Girrbach AG, Koblach, Austria) were soft milled (by the Ceramill Motion 2) into rectangular-shape specimens ( Fig. 1 ). Prior sintering, a diamond round bur in a drill press with a micrometer stage was used to drill one hole in each specimen with the objective of enabling the attachment to the mechanical testing machine.
One disc-shape (10 mm thick and 10 mm diameter) specimen per group was also soft milled by CAD/CAM for surface topography analysis.
After cleaned and dried, all the specimens were sintered in a high-temperature furnace (Ceramill therm, AmannGirrbach AG, Koblach, Austria) at 1450 °C for 2 h in accordance to Programe 1 for Ceramill Zi.
After complete sintering, all disc-shape specimens were ground with 200–800 grit SiC (silicon carbide) abrasive paper and polished with 1 μm and 0.5 μm diamond paste followed by thermal etching during 10 min at 1350 °C . This grounding and polishing step was introduced in order to enable Nano roughness characterization, even though it is not likely to be part of the process for the C(T) specimens. As Flamant et al. , the authors assumed that the effect of the treatments proposed in this study on a polished and thermal etched surface or as sintered surface Nano roughness would be comparable since the presence of a Micro scale relief in as sintered zirconia surface avoid the Nano roughness AFM characterization, see Appendix A.
2.2
Surface treatments
The samples were randomly divided into 5 groups in accordance with the surface treatment proposed, wherein group 1 (G1, control) received no surface treatment was done.
In group 2 (G2), the specimens had their bonding surface coated with a thin low melting glass layer (composition in Table 1 ). A standardized amount (5 g ± 1 g) of glass was applied with a micro brush tip on the bond surface. The specimen was held on a vibrating plate for 5 s and then allowed to dry for 60 s. Heated in air to 750 °C (30 °C/min) for 3 min. After that, the glass formed a thin and uniform layer — about 0.5 mm verified using the Mitutoyo Absolute Digimatic Indicators. This thin glass layer was completely removed by a 10% hydrofluoric acid solution in ultrasonic bath for 50 min at 20 °C, followed by washing with distilled water for 5 min.
Materials | Chemical composition a |
---|---|
IPS e.max ® CeramGlaze paste (Low fusion glass) | SiO 2 (61–68 wt%); Al 2 O 3 (5–8 wt%); Na 2 O (5–8 wt%); K 2 O (5–8 wt%); ZnO (2–4 wt%); other oxides (3–17 wt%); pigments (0–1 wt%); glycerine (20–25 wt%) and 1,3-butandiol (15–20 wt%). |
RelyX™ U200 | Base paste: methacrylate monomers containing phosphoric acid groups, methacrylate monomers, silanated fillers, initiator components, stabilizer, rheological additives |
Catalyst paste: methacrylate monomers, alkaline fillers, silanated fillers, stabilizer, initiator components, pigments, rheological additives. | |
Ceramill ZI | Yttria stabilized polycrystalline tetragonal zirconia (Y-TZP) blank (ZrO 2 + HfO 2 + Y 2 O 3 ≥ 99.0 wt%) |
The specimens in group 3 (G3) were exposed to the same heat procedure used in G2 without the low melting glass coating. The specimens in group 4 (G4) were immersed in the same acid solution as G2 (10% hydrofluoric acid solution at 20 °C) for 50 min intending to evaluate the influence of glass infiltration agent and acid etching.
Group 5 (G5) samples were subjected to abrasion with Al 2 O 3 particles (50 μm) with a dental airborne-particle abrasion unit (Micro-Blaster; Daedong Industrial Co., Ltd., Daegu, Korea) that was applied for 15 s at a pressure of 2.5 bar with 15 mm between the nozzle and the surface.
After air abrasion, G5 specimens received ultrasonic cleaning in absolute ethanol for 10 min and groups G1, G2, G3 and G4 specimens received ultrasonic cleaning in distilled water for 10 min .
2.3
X-ray diffraction analysis (XRD)
Subsequently to the Y-TZP surface treatments, to observe possible phase transformations of dental Y-TZP, the disk samples were subjected to X-ray diffraction analysis (XRD-6000, Shimadzu, Japan) using Cu-Kα ( λ = 0.154 nm) radiation. The X-ray diffractograms of the 5 groups were obtained and compared with the crystal structure data obtained from the ICSD Data Base. The diffractograms of all groups were analyzed by Rietveld method using TOPAS academic software , to calculate the phase’s content.
2.4
Atomic force microscopy (AFM)
After the XRD analysis, five zirconia discs (1 per group) were evaluated by atomic force microscopy (JPK, NanoWizard ® , Berlin, Germany). Images were taken in air using the tapping mode performed with a POINTPROBE ® NCSTR-10 (NanoWorld, Neuchâtel, Switzerland) cantilever tip with radius of curvature less than 8 nm. Five images per group were recorded with a resolution of 512 × 512 (pixels) and scanning area of 5 × 5 (μm).
AFM data assessment of average surface roughness (R a ), Skewness (R sk ) and surface area variation (%) was performed using the Statistical Quantities tool of Gwyddion AFM data analysis software . This software follows the Materials Information Society standards: ASME B46.1-1995 and ASME B46.1-1985 recommendations and the International Organization for Standardization ISO 4287-1997 and ISO 4287/1-1997 standards.
Roughness Average (Ra) is the average deviation of all points roughness profile from a mean line over the evaluation length given by Eq. (1)
R a =1 / N Σ j=1 N | r j |
where, R a is the arithmetical roughness average, N is the number of roughness profile points and <SPAN role=presentation tabIndex=0 id=MathJax-Element-2-Frame class=MathJax style="POSITION: relative" data-mathml='|rj|’>|rj||rj|
| r j |
is the height values in modulus.
Skewness (R sk ) is an amplitude parameter that describes the shape of the Amplitude Distribution Function (ADF) that is a probability function that gives the probability that a surface profile has a certain height value at any position over the evaluation length .
Skewness is the degree of the asymmetry of the ADF, or equivalently, it measures the symmetry of the surface profile heights about its mean line and is given by Eq. (2)
R sk =1 / NR q 3 Σ j=1 N r j 3
where, R sk is the skewness, N is the number of roughness profile points, R q is the standard deviation height values and rj is the height values over the evaluation length.
Positive skewness (R sk > 0) indicates the preponderance of peaks, negative skewness (R sk < 0) indicates prevalence of valley structures and a zero skewness points a good distributions of valleys and peaks.
The relative surface area (A Rel ) was estimated by the Statistical Quantities tool of Gwyddion AFM data analysis software following the method of triangulation scheme . Then the area increase in percentage of the projected area (5 × 5 μm 2 ) was obtained by Eq. (3)
Area ( % ) = 100 × A Rel − 25 / 25
where, Area (%) is the area increase, A Rel is the relative surface area and 25 is the calculated projected area of the AFM images.
Average roughness values were first checked for normal and equal distribution (Kolmogorov-Smirnov and Levene’s tests). As data were not normally distributed, the non-parametric Kruskall–Wallis’ test was applied to assess significant differences among the experimental groups ( α = 0.05). The Mann–Whitney’s test was applied for post hoc comparisons (p < 0.05). All non-parametric statistical analyses were handled with IBM SPSS and PAST Statistics Software.
2.5
Fracture mechanics
2.5.1
C(T) specimen preparation
The resin cement and dental Y-TZP ceramic used are listed in Table 1 . The steps associated with each bonding procedure were in accordance with instructions given by the manufacturers
A C(T) specimen with a bonded interface along the midplane was used for fracture toughness testing ( Fig. 1 ). The specimens’ dimensions followed the C(T) test recommendations of ASTM E-399.
After surface treatments performed according to their experimental group, twenty Y-TZP half specimens were subjected to luting procedure to form the set of 10 specimens per each treatment group (N = 10).
Measurements of the dimensional parameters: a (crack length), W (net length) and B (thickness) for each half specimen were then recorded using a digital caliper (see Appendix B) and the bond area limits (8 mm × 8 mm) were demarcated on the lateral face of the specimen.
2.5.2
Luting procedure
One half specimen was placed in an acrylic split mold to prevent movement and guarantee standardized luting procedure. To provide the notch at the interface, a razor blade (Gillette ® ) was placed as a spacer as an alternative “precraking” method indicated in Kovarik and Fairhurst’s paper . The razor blade was cut with 8.5 mm width, and it was isolated with a thin layer of solid petroleum jelly before being placed exactly at the notch position (a) on the surface of the one-half specimen previously positioned inside the acrylic split mold.
The self-etching resin cement RelyX™ U200 (3M ESPE Dental, St. Paul, MN, USA), 1518200193 batch number (composition in Table 1 ), was mixed according to manufacturer’s instructions and applied between the two zirconia blocks. A constant pressure of 40 g/mm 2 was applied and a homogeneous cement layer (70 μm ± 10 μm) was produced. After the working time (6 min), the specimen was placed in a light cure polymerization unit (UniXS, Heraeus Kulzer GmbH, Hanau, Germany) for 180 s.
In order to check if the cement layer was homogenous in the specimens, digital images of each specimen was taken to measure the cement thickness using an image processing software.
After the luting procedure, the specimen was immediately stored in distilled water at 37 °C for 24 h prior to mechanical testing .
2.5.3
Fracture mechanics compact tension (C(T)) test
The specimens were fractured in an EMIC universal testing machine (Model DL 10000, Instron Brasil Equipamentos Científicos LTDA; São José dos Pinhais, PR; Brazil) at cross-head speed of 0.1 mm/min.
Maximum load at fracture was recorded for each specimen and apparent K IC was calculated according to Eq. (4) .
K I C = ( P max / B W 1 / 2 ) × f ( a / W )
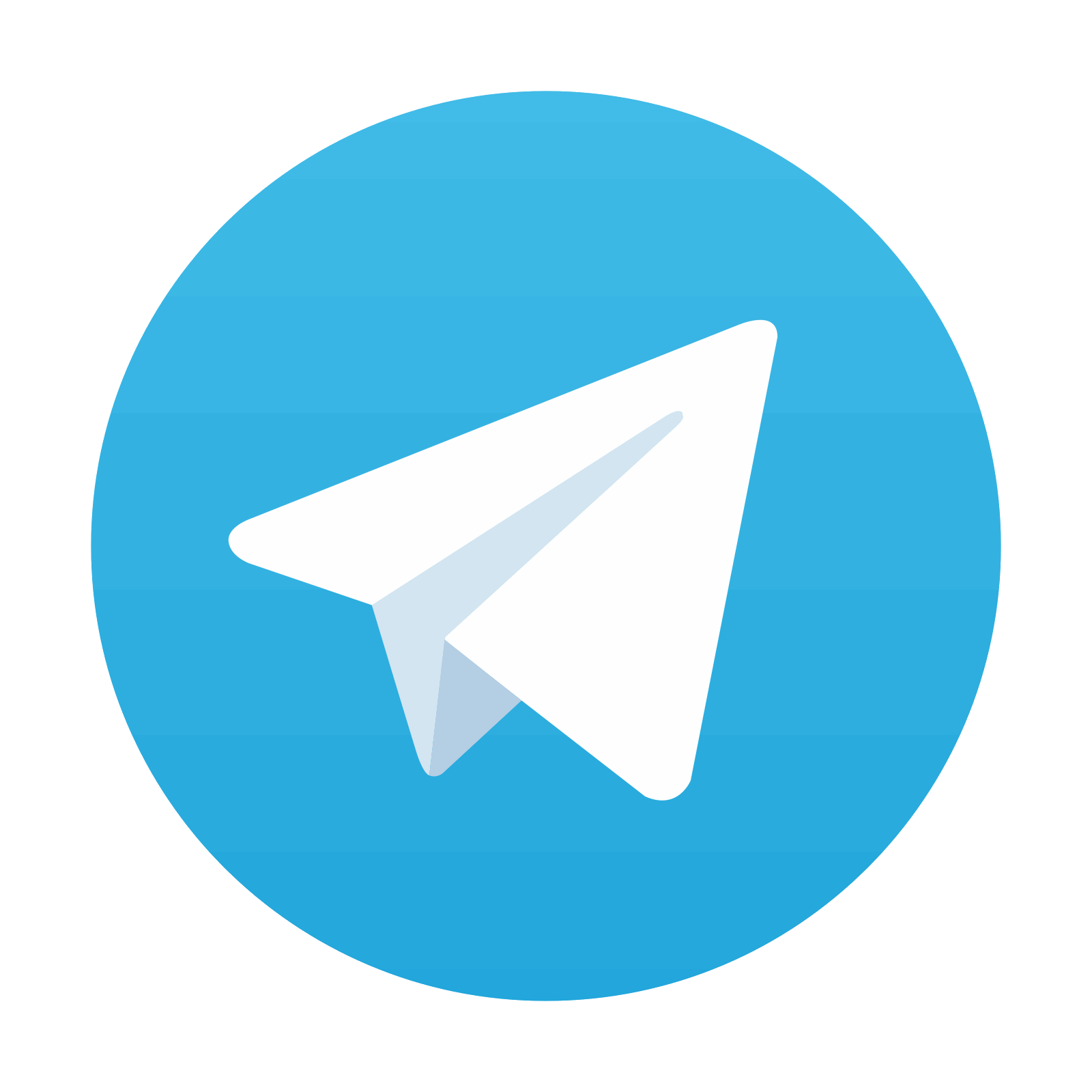
Stay updated, free dental videos. Join our Telegram channel

VIDEdental - Online dental courses
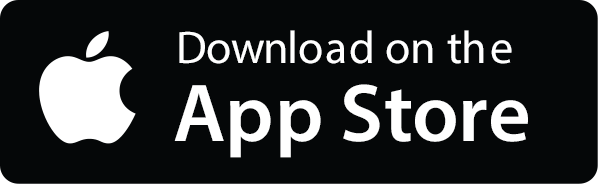
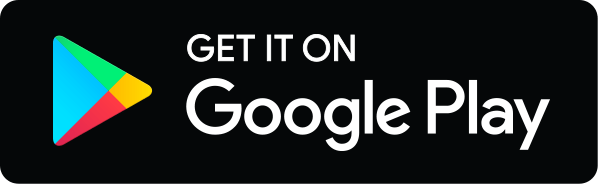