Abstract
Objectives
The aim was to investigate the influence of number average molecular weight and concentration of the poly(acrylic) acid (PAA) liquid constituent of a GI restorative on the compressive fracture strength ( σ ) and modulus ( E ).
Methods
Series of PAA solutions for eight experimental PAAs with molecular weights ranging from 5000 to 200,000 were prepared at concentrations ranging from 10 to 60%. The PAA number average molecular weights were determined using gel permeation chromatography and the viscosity at each concentration was determined using a digital viscometer. The PAA solutions were hand-mixed with a commercial GI restorative powder (Ionofil Molar; Voco, Cuxhaven, Germany). σ and E were determined at 24 h using cylindrical (6 mm height, 4 mm diameter) specimens ( n = 20). Data was analyzed using regression analyses and two-way analyses of variance at p < 0.05.
Results
Regression analyses showed a significant reduction in PAA solution viscosity with reducing PAA number average molecular weight. Two-way ANOVAs for σ and E indicated a significant interaction of number average molecular weight × concentration ( p < 0.001 and p < 0.001, respectively). Quadratic regression analyses showed significant curvature (n-shaped curves) within σ and E data for individual PAA number average molecular weights with increasing concentration. As a result, the optimum PAA concentration for each PAA number average molecular weight was determined from the curve vertexes.
Significance
The choice of PAA molecular weight and concentration and the subsequent viscosity of the PAA solution have a significant influence on the σ and E of a GI restorative, however, the relationship is not a simple one.
1
Introduction
Glass-ionomers (GIs) are a versatile class of dental material that can be employed clinically for the retention of restorations on prepared teeth , as cavity liners or bases or the restoration of natural dentition which makes them indispensable to the dental practitioner . Although available in encapsulated form , GIs are routinely supplied as separate powder and liquid constituents which are hand-mixed to a paste consistency with the relative proportion of powder for a constant volume of liquid varying depending on the particular clinical application . Increased mechanical properties are required to withstand masticatory forces in service when GIs are employed as cavity liners and bases or as restoratives and therefore the powder content is increased compared with GIs used as luting cements. The information available in the dental literature on the influence of powder:liquid mixing ratio on the performance of GI luting and restorative formulations is extensive. However, little information is available on the influence of the polymeric component, namely the polyacrylic acid (PAA) liquid in terms of number average molecular weight and concentration .
Dynamic mechanical thermal analysis of GI cements highlighted the presence of sharp loss peaks typical of thermoplastics and Hill et al. thus considered GIs to be thermoplastic polymer composites since the ionic cross-links are labile and are continually breaking and reforming. This view was supported by Gonzalez who identified ionic cross-links in polystyrene sulfonate ionomers had a limited lifetime. Most polymers exist as a series of chain-like molecules, randomly orientated in a coil configuration in the melt and glassy state , and for chain scission to occur the polymer chain needs to be stretched during deformation and subsequent fracture . Entanglement theory (also known as the reptation model) was proposed by Edwards who recognized that entanglements in polymer melts were not just rigid knots but that a chain could be regarded as being contained within a tube formed by the neighboring chains. Lateral movement of chains was constricted by the presence of entanglements while longitudinal movement was restricted by interactions with neighboring chains . As a result, longer polymer chain lengths (which are proportional to the polymers number average molecular weight) required more stress to remove the chain contained within a tube formed by neighboring chains therefore resulting in a stronger polymer . Previous research suggests a critical number average molecular weight ( M crit ) exists at which the stress required to remove the chain from the tube formed by neighboring chains would be greater than the stress required to cause chain scission . Chain pull-out was therefore proposed as the dominant failure mechanism below M crit , while chain scission was the dominant failure mechanism above M crit . In line with the previous work by Prentice and the application of a simple power law viscous model for PAA, Hill et al. suggested M crit to be approximately 100,000. However, the authors also suggested that, where there were ‘strong interactions’ existing between the polymer chains, M crit would decrease below 100,000 in line with the observations of Martin et al. . Additionally, Hill et al. suggested the entanglement number average molecular weight ( M ent ) for PAA lay between 7000 and 21,000 – corresponding to between 300 and 700 monomeric units in accordance with the findings of Ferry .
Wilson et al. used PAA liquid solutions and although a progressive increase in compressive fracture strength was reported for the GI investigated (prepared with PAA solutions with increasing molecular weights from 3500 to 230,000) no details of polydispersity index or the molecular number of the PAAs employed were reported. Progressive increases in compressive fracture strength have been reported for anhydrous GIs prepared with PAAs with increasing molecular weights (11,500–1,080,000 , 9400–263,000 and 9300–210,000 ). In the anhydrous GI systems assessed, the PAA was vacuum-dried and added to the GI glass powder prior to mixing with a tartaric acid solution. Vacuum-dried PAA needed to be dissolved in the available water prior to the initiation of the setting reaction and therefore, the cement forming reaction was retarded which enabled higher PAA molecular weights to be used . However, there were little differences in compressive fracture strengths reported with PAA molecular weights in excess of 100,000 which appeared to confirm the suggestion of Hill et al. that M crit for PAA was approximately 100,000 albeit for the anhydrous GIs investigated . The anhydrous GIs were prepared with powder contents much lower than would be deemed acceptable for routine use clinically as restorative formulations since the mechanical properties were poor and the working characteristics markedly extended above that recommended in ISO 9917-1 .
The aim of the current investigation was to investigate the influence of the PAA liquid of a GI restorative in terms of number average molecular weight and concentration on the compressive fracture strength ( σ ) and the elastic modulus ( E ). The PAA number average molecular weights examined in this study were chosen to encompass a broad range both above M crit and below M ent suggested by Hill et al. for anhydrous GIs.
2
Materials and methods
2.1
Materials
The GI restorative powder used to prepare specimens with the experimental PAAs under investigation was the powder constituent of Ionofil Molar (Voco, Cuxhaven, Germany) which is marketed as a hand-mixed GI restorative. The experimental PAAs used in the current study were supplied by the manufacturers as either powders or liquids with reported molecular weights varying from 5000 to 200,000. The PAAs supplied as powders had reported molecular weights of 15,000 (PAA15), 25,700 (PAA26), 40,000 (PAA40) and 80,800 (PAA81) ( Table 1 ). The PAAs supplied as liquids – with reported molecular weights of 5000 (PAA5), 50,000 (PAA50), 100,000 (PAA100) and 200,000 (PAA200) and the liquid component of Ionofil Molar reported to have a molecular weight of 70,000 (PAA70), were vacuum dried to remove the water. The resulting powder was ground using a pestle and mortar to a similar particle size to the PAAs supplied in powder form.
PAA | Manufacturer | Supplied as |
---|---|---|
PAA5 | Polysciences Europe GmbH, Eppelheim, Germany. | Liquid |
PAA15 | Advanced Healthcare Limited, Tonbridge, England. | Powder |
PAA26 | Robert Hill, Queen Mary, University of London, England. | Powder |
PAA40 | Advanced Healthcare Limited, Tonbridge, England. | Powder |
PAA50 | Polysciences Europe GmbH, Eppelheim, Germany. | Liquid |
PAA70 | Voco, Cuxhaven, Germany | Liquid |
PAA81 | Robert Hill, Queen Mary, University of London, England. | Powder |
PAA100 | Polysciences Europe GmbH, Eppelheim, Germany. | Liquid |
PAA200 | Polysciences Europe GmbH, Eppelheim, Germany. | Liquid |
2.2
Gel permeation chromatography
The number average molecular weight, molecular number and polydispersity index of the experimental PAAs and the liquid component of the Ionofil Molar GI restorative were determined using gel permeation chromatography (Varian 210; Varian Inc., Palo Alto, CA, USA) coupled with a refractive index detector (Varian 350 RI Detector; Varian Inc. Palo Alto, CA, USA). An aqueous gel permeation column was used (PL Aquagel OH-Mixed 8 μm; Varian Inc. Palo Alto, CA, USA) in conjunction with an eluent, a phosphate buffer maintained at pH level of 7.4 (K 2 HPO 4 + H 3 PO 4 ), at a flow rate of 0.2 mL/min during the analysis. Molecular weight calibration was conducted using PAA standards with molecular weights of 2000 and 350,000. The gel permeation chromatography assembly was run overnight with the eluent at a flow rate of 0.2 mL/min. Each experimental PAA powder was diluted using the phosphate buffer solution to a 1% PAA concentration by volume, injected slowly into the gel permeation chromatography assembly and monitored until the number average molecular weight and molecular number were determined by the software. The procedure was repeated in triplicate for each PAA solution investigated and the mean number average molecular weight, mean molecular number and polydispersity index for the nine PAAs determined ( Table 2 ).
PAA | Molecular weight | Molecular number | Polydispersity index |
---|---|---|---|
PAA5 | 5330 (494) | 3469 (277) | 1.54 |
PAA15 | 15,250 (1567) | 8680 (691) | 1.76 |
PAA26 | 25,910 (1863) | 13,680 (1550) | 1.89 |
PAA40 | 40,156 (2717) | 21,930 (2422) | 1.83 |
PAA50 | 50,335 (1393) | 26,468 (1933) | 1.90 |
PAA70 | 69,471 (3014) | 37,573 (1943) | 1.85 |
PAA81 | 80,650 (5400) | 41,840 (3069) | 1.93 |
PAA100 | 99,927 (9328) | 48,670 (5036) | 2.05 |
PAA200 | 206,593 (17,186) | 95,039 (13,531) | 2.17 |
2.3
Polyacrylic acid solution preparation
A series of solutions of each experimental PAA investigated were prepared at concentrations ranging from 10 to 60% by dissolving the appropriate PAA powder weight (measured using a balance accurate to 0.001 g (Sartorius Expert; Sartorius AG, Goettingen, Germany)) into 2 mL of distilled water at room temperature for 24 h prior to use.
2.4
Viscosity determination
The viscosity of each experimental PAA investigated and prepared at concentrations ranging from 10 to 60% was measured using a digital viscometer (Brookfield DV-E Viscometer; Brookfield Engineering Laboratories Inc., Middleboro, MA, USA). A small sample adaptor was attached to the viscometer and 2.1 mL of a PAA solution was dispensed into the inner chamber using an adjustable 200 μL pipette (Gilson, Middleton, WI, USA). A spindle was inserted slowly into the chamber to avoid entrapping air bubbles in the PAA solution and attached securely to the viscometer. The spindle was rotated in the PAA solution at 100 rpm until a constant viscosity reading was obtained and the viscosity (mPas) was recorded. The procedure was repeated in triplicate and the mean viscosity for each PAA molecular weight and PAA concentration investigated was calculated.
2.5
GI restorative preparation
The Ionofil Molar GI powder was hand-mixed at a powder to liquid mixing ratio of 4:1 (g:g) as a standard throughout the current study in accordance with the manufacturers’ instructions. A glass slab was placed on the balance, 0.188 g of an experimental PAA solution was dispensed onto the glass slab using an adjustable 200 μL pipette (Gilson, Middleton, WI, USA) along with 0.75 g of the Ionofil Molar powder. The powder was separated into two parts, the first part was mixed with the liquid for 20 s using a stainless steel spatula with the remaining powder added and mixed to ensure a total mixing time of 40 s.
2.6
Compression testing protocol
Cylindrical GI specimens (6.0 ± 0.1 mm height and 4.0 ± 0.1 mm diameter) were fabricated in accordance with ISO 9917-1: 2003 using a polytetraflouroethylene (PTFE) split-mold assembly . The PTFE split-mold was placed on a PTFE base covered with an acetate strip and aligned with a holding pin and nylon wedges to ensure equal pressure was applied along the length of the split-mold . The restorative mix was applied to the split-mold using a stainless steel spatula within 60 s of the completion of mixing. The largest convenient portion of the mix was conveyed to one side of an individual hole and allowed to flow into the mold to provide laminar flow and minimize the incorporation of air bubbles into the cylindrical specimens . The filled split-mold assembly was covered with an acetate strip and isolated from the surrounding atmosphere using a glass slab. To ensure parallelism of the cylindrical specimen ends for uniform contact with the compressive platens of the testing machine , the split-mold assembly was clamped with a G-clamp. The clamped assembly was stored in a water-bath maintained at 37 ± 1 °C for 1 h. The cylindrical specimens were removed from the split-mold and hand-lapped on P600 silicon carbide abrasive paper (Buehler, Lake Bluff, IL, USA) using water as a lubricant. Specimens which contained visible defects such as chipped edges, non-parallel ends or pores were discarded. The specimens were placed into 50 mL of distilled water, maintained at 37 ± 1 °C, and placed into an incubator (Climate Cabinet; Firlabo, Meyzieu, France) for a further 23 h prior to compression testing. The specimen preparation procedure was repeated so that a minimum of 20 cylindrical GI restorative specimens were prepared for each experimental PAA number average molecular weight and concentration investigated.
Twenty-four hours after the commencement of mixing, the specimens were removed from the incubator and the mean diameter of each cylindrical specimen was determined using a digital micrometer screw gauge reading to 1 μm (Mitutoyo, Kawasaki, Japan). The specimens were tested ‘wet’ to mimic the oral environment by placing a piece of wet filter paper on the flat ends of each cylindrical specimen . A compressive load was applied to the long axis of the specimen at 1 mm/min using a universal testing machine (Instron Model 5565, High Wycombe, England) and the maximum load at failure was recorded.
The compressive fracture strength ( σ ) in MPa of each specimen was determined using Eq. (1) :
σ = 4 F f π d 2
where F f was the load at fracture (N) and d was the mean original specimen diameter (mm). The modulus ( E ) in GPa was determined by calculating the slope of the linear portion of the stress–strain plot generated for each individual cylindrical specimen as shown in Fig. 1 .

2.7
Statistical analyses
Statistical analyses (two-way analyses of variance (ANOVAs)), as well as regression analyses were made in software (SPSS 12.0.1; SPSS Inc., Chicage, IL, USA) at a significance value of p = 0.05. Individual two-way ANOVAs for viscosity, σ and E , respectively were determined where factors were identified as the number average molecular weight and concentration. Regression analyses were conducted to check for general trends within the viscosity, σ and E data for each PAA molecular weight and concentration.
2
Materials and methods
2.1
Materials
The GI restorative powder used to prepare specimens with the experimental PAAs under investigation was the powder constituent of Ionofil Molar (Voco, Cuxhaven, Germany) which is marketed as a hand-mixed GI restorative. The experimental PAAs used in the current study were supplied by the manufacturers as either powders or liquids with reported molecular weights varying from 5000 to 200,000. The PAAs supplied as powders had reported molecular weights of 15,000 (PAA15), 25,700 (PAA26), 40,000 (PAA40) and 80,800 (PAA81) ( Table 1 ). The PAAs supplied as liquids – with reported molecular weights of 5000 (PAA5), 50,000 (PAA50), 100,000 (PAA100) and 200,000 (PAA200) and the liquid component of Ionofil Molar reported to have a molecular weight of 70,000 (PAA70), were vacuum dried to remove the water. The resulting powder was ground using a pestle and mortar to a similar particle size to the PAAs supplied in powder form.
PAA | Manufacturer | Supplied as |
---|---|---|
PAA5 | Polysciences Europe GmbH, Eppelheim, Germany. | Liquid |
PAA15 | Advanced Healthcare Limited, Tonbridge, England. | Powder |
PAA26 | Robert Hill, Queen Mary, University of London, England. | Powder |
PAA40 | Advanced Healthcare Limited, Tonbridge, England. | Powder |
PAA50 | Polysciences Europe GmbH, Eppelheim, Germany. | Liquid |
PAA70 | Voco, Cuxhaven, Germany | Liquid |
PAA81 | Robert Hill, Queen Mary, University of London, England. | Powder |
PAA100 | Polysciences Europe GmbH, Eppelheim, Germany. | Liquid |
PAA200 | Polysciences Europe GmbH, Eppelheim, Germany. | Liquid |
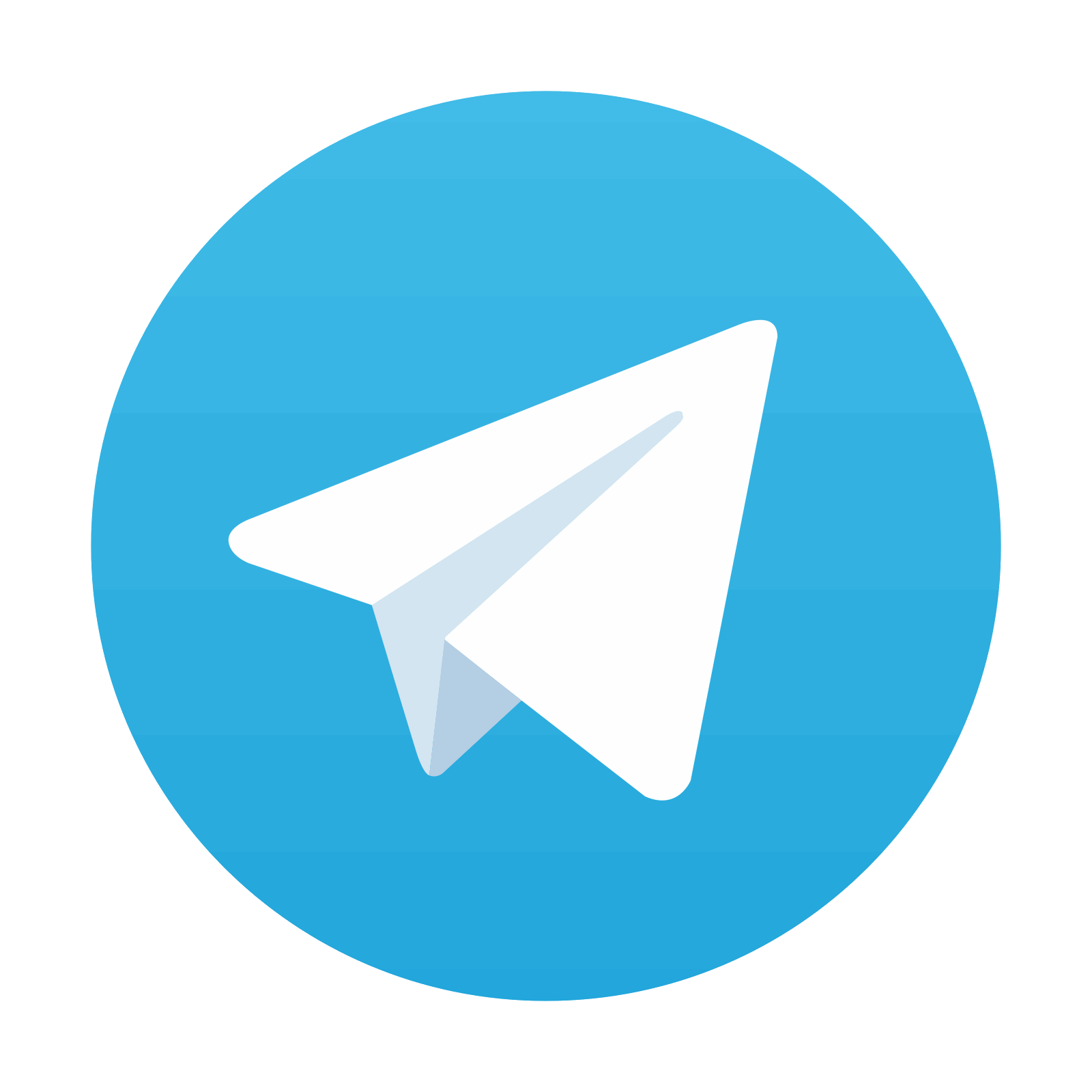
Stay updated, free dental videos. Join our Telegram channel

VIDEdental - Online dental courses
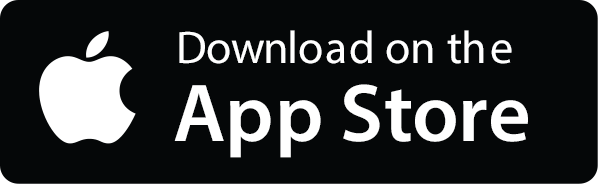
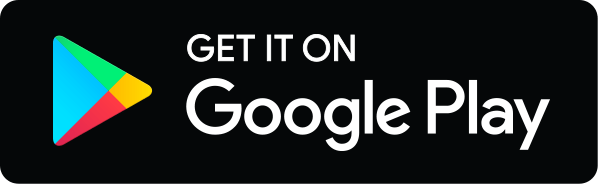
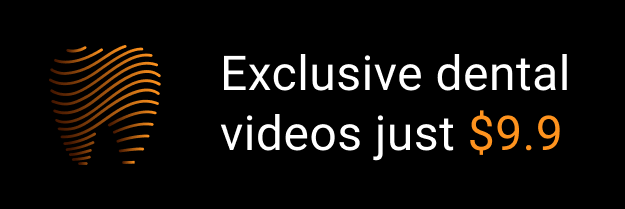