Abstract
Objective
To assess the influence of laser-textured surfaces on the adhesion of composite to dentin after being rotary prepared.
Methods
Thirty healthy teeth were kept in 0.1% thymol solution prior to being ground down to dentin to create a 4 × 4 mm 2 flat surface. Teeth were divided into 3 groups ( n = 10). Groups 1 and 2 utilized the prototype Erbium doped, Yttrium–Aluminum–Garnet Er:YAG laser by Dental Photonics, Inc. A single pulse was delivered to each spot to create an equally spaced square 4 × 4 mm 2 matrix of micro craters. All craters had 100 μm diameter/45 μm depth; two different spacing patterns were prepared in Groups 1 and 2. In Group 1, distance between crater centers was 50 μm; Group 2 had 100 μm. In Group 3 (control), 10 samples were prepared without laser texturing. G-bond (GC America) was applied to testing area of all samples in all groups according to manufacturer’s instructions. Bonding resin was applied and shear-bond strength tests were employed using an Instron machine to measure adhesive strength.
Results
One-way analysis of variance (ANOVA) was used to compare the 3 groups. Pair wise t -tests implementing the Bonferroni correction for multiple comparisons found a statistically significant difference between Group 3 and Group 2 ( p = 0.019) but no statistically significant difference between Group 3 and Group 1 ( p = 0.263) or Group 1 and Group 2 ( p = 0.743).
Significance
The bond strengths between bonded composite to laser-textured dentinal surfaces with larger spacing patterns are greater than that of non-textured surfaces.
1
Introduction
Modern dentistry has been concerned with reducing patients’ risk of caries, preserving tooth structure, and noninvasive conservative techniques such as composite restorative material. However, the benefit of this treatment relies upon the ability of the restorative material to promote an appropriate sealing and remain completely intact and bonded to the surface, thus increase the longevity of the restoration.
Air turbine drills for preparations of surfaces has been the most used method. It is less expensive and smaller in and can be used with ease. However, it presents disadvantages like the unpleasant noise and vibration in the dental structure, which can generate pain and tension in the patients as well as cause contamination of the clinical atmosphere . Alternative methods for preparing dental surfaces, such as laser , that emits light through a process of optical amplification based on the stimulated emission of photon, have been developed and their effect on bond strength to tooth structure has been a research interest.
The application of laser beam on dental hard tissue was investigated by Goldman et al. shortly after its discovery by Maiman . Improvements in laser technology have led to a great number of applications in the field of dentistry such as periodontal soft tissue surgery , gingivectomy , crown lengthening procedure , gingival retraction , frenectomy , treatment of aphthous ulcers , tooth whitening , endodontic procedures , caries detection , caries removal and controlled bleeding post dental operative surgery .
The carbon dioxide (CO 2 ) laser was the first dental laser approved by the FDA and has been successfully used in soft tissue surgeries . The Neodymium–Yttrium–Aluminum–Garnet (Nd:YAG) laser uses a fiber optic delivery system that penetrates wet tissue more easily than the CO 2 laser. There has been interest in using the Nd:YAG laser on mineralized tissue to possibly enhance the bond strength of composite to dentin , but the Nd:YAG laser is still not approved for hard tissue applications.
Erbium doped, Yttrium–Aluminum–Garnet (Er:YAG) laser is a system that can be used for both soft and hard tissue procedures but most of its applications has focused on hard tissue . Er:YAG laser is a system which was approved by the FDA for removal of caries, preparations of cavities, and modification of enamel and dentin .
It has been shown that preparation of tooth using Er:YAG did not significantly increase the temperature compared to a rotary handpiece and the thermal effects are negligible . In addition, it was demonstrated that the Er:YAG laser produces smaller thermal effects in comparison to other laser system during tooth preparation .
Unlike enamel, dentin contains a higher percentage of water and organic material like collagen and is heterogeneous tissue . Composite bonding to dentinal surfaces poses a greater challenge than enamel due to its complexity. Therefore, dentinal substrate is less receptive to adhesive treatments. Mechanical theories state that adhesives interlock micromechanically with irregularities of the surface of the adhered .
The reported bond strengths of composite to (Er:YAG) laser textured surfaces vary in the literature. For example, Visuri et al. reported that shear bond strength of composite to dentin prepared with an Er:YAG laser was significantly higher. In contrast, Sakakibara et al. , Van Meerbeek et al. , and Cardoso et al. showed that the bond strength to laser-irradiated dentin decreased. In addition, Armengol et al. , Sattabanasuk et al. and Kataumi et al. found no difference between laser-irradiated and non-irradiated teeth.
In most of these past studies of bonding to Er:YAG lased surface, the surface of dentin was uniformly irradiated. In other studies, spatial patterning of dentin surfaces was postulated to influence the magnitude of the bond strength to composite. For example, as shown by Gardner et al. , dentin surfaces irradiated with varying spatial overlap of adjacent laser pulses produced different bond strengths to composite. However, in this study, the shear bond strength of the non-irradiated dentin control group was always higher than those of irradiated ones. Therefore, producing a laser-textured dentin surface with appropriate spatial pattern that yields high shear bond strength to composite poses a greater challenge. To this end, the aim of this study is to assess the influence of the laser textured surface, prior to the adhesion of composite to dentin after preparation with diamond disk.
We hypothesize that texturing the surface of the rotary prepared dentin using spatial patterns with Er. YAG laser would significantly affect the shear bond strength of resin composite to dentin.
2
Materials and methods
2.1
Teeth collection
Thirty human molar teeth were collected and kept in 0.1% thymol solution for no more than six months prior to specimen preparation. The teeth were free of visible caries and other surface defects. The buccal surface of each tooth was ground down with bur part number 806 with a diamond head ADO-22 (“NPOOO Systema” company, Minsk, Belarus) to create a flat uniform layer of peripheral dentin of 4 × 4 mm 2 surface. Teeth were randomly divided into 3 groups ( n = 10).
Teeth in Groups 1 and 2 were micro-textured to create craters with diameter of 100 μm and depth of 45 μm. This was done using the laser device which is explained below. However, two different spacing patterns were prepared in Groups 1 and 2. In Group 1, distance between crater centers was 50 μm. For Group 2 this was 100 μm. In Group 3 (control), ten samples were prepared without laser texturing.
2.2
Laser device
The Er:YAG laser apparatus (prototype laser by Dental Photonics, Inc.) emits radiation at a wavelength of 2940 nm, with an output energy in the range 30–350 mJ/pulse, repetition rate of 1 Hz, and pulse mode of 100 μs duration. Energy output was monitored using a power meter (Field Master and detector LM-P10i; Coherent Company, OH). Laser irradiation was performed perpendicular to the dentin surface to create cone shaped craters. The flat 4 × 4 mm 2 dentin surface was placed in a focal plane of 38 mm lens. A single pulse with 1.2 mJ energy was delivered to each spot and then the sample was laterally moved to the next spot to create an equally spaced 4 × 4 mm 2 matrix of laser micro craters.
2.3
Bond strength measurements
The teeth were then embedded in acrylic in similar size and shape molds with prepared buccal dentin surfaces extending 1-mm above the level of the acrylic.
G-Bond (GC America) was applied to testing area of all samples in all groups according to manufacturer’s instructions. The surface of the prepared area was gently dried by blowing air with air syringe. G-Bond was applied and left undisturbed for 10 s and then was thoroughly dried under maximum air pressure for 5 s. It was cured with a halogen light for 10 s.
An approximately 3-mm long composite rod (Tetric-EvoCeram, A1 shade) was bonded to all dentin specimens using a jig (Ultradent) with an inner diameter of 2.38-mm. The mold was secured to the specimen, and then two 1.5-mm increments of composite were polymerized separately with halogen light for 40 s each. The jig was removed and specimens were stored in water at 37 °C for 24 h. After this period, Shear-bond strength tests were employed using Universal Testing Machine (Instron 4201) to measure adhesive strength in megapascals (MPa) with crosshead speed of 5 mm/min.
The force to failure was recorded for each specimen. The mean shear bond strength in MPa was determined for each group. Fractured specimens were observed with a stereomicroscope (Olympus) at 20× magnification for determination of failure modes, such as cases of cohesive, and mixed with adhesive.
Data were subjected to a one-way analysis of variance (ANOVA) to compare the effects of preparation type among the three groups. All statistical analyses were performed at p < 0.05.
2.4
Scanning electron micrographs
For electrospunned silk mats, the materials were sputter-coated with gold/palladium using a Polaron SC502 Sputter Coater (Fison Instruments, UK). Specimens were then examined using an ISI-DS-130 SEM (Avon, CT) at 15 kV. Images of representative samples were obtained once prior to bonding and once after shear-bond strength test.
2
Materials and methods
2.1
Teeth collection
Thirty human molar teeth were collected and kept in 0.1% thymol solution for no more than six months prior to specimen preparation. The teeth were free of visible caries and other surface defects. The buccal surface of each tooth was ground down with bur part number 806 with a diamond head ADO-22 (“NPOOO Systema” company, Minsk, Belarus) to create a flat uniform layer of peripheral dentin of 4 × 4 mm 2 surface. Teeth were randomly divided into 3 groups ( n = 10).
Teeth in Groups 1 and 2 were micro-textured to create craters with diameter of 100 μm and depth of 45 μm. This was done using the laser device which is explained below. However, two different spacing patterns were prepared in Groups 1 and 2. In Group 1, distance between crater centers was 50 μm. For Group 2 this was 100 μm. In Group 3 (control), ten samples were prepared without laser texturing.
2.2
Laser device
The Er:YAG laser apparatus (prototype laser by Dental Photonics, Inc.) emits radiation at a wavelength of 2940 nm, with an output energy in the range 30–350 mJ/pulse, repetition rate of 1 Hz, and pulse mode of 100 μs duration. Energy output was monitored using a power meter (Field Master and detector LM-P10i; Coherent Company, OH). Laser irradiation was performed perpendicular to the dentin surface to create cone shaped craters. The flat 4 × 4 mm 2 dentin surface was placed in a focal plane of 38 mm lens. A single pulse with 1.2 mJ energy was delivered to each spot and then the sample was laterally moved to the next spot to create an equally spaced 4 × 4 mm 2 matrix of laser micro craters.
2.3
Bond strength measurements
The teeth were then embedded in acrylic in similar size and shape molds with prepared buccal dentin surfaces extending 1-mm above the level of the acrylic.
G-Bond (GC America) was applied to testing area of all samples in all groups according to manufacturer’s instructions. The surface of the prepared area was gently dried by blowing air with air syringe. G-Bond was applied and left undisturbed for 10 s and then was thoroughly dried under maximum air pressure for 5 s. It was cured with a halogen light for 10 s.
An approximately 3-mm long composite rod (Tetric-EvoCeram, A1 shade) was bonded to all dentin specimens using a jig (Ultradent) with an inner diameter of 2.38-mm. The mold was secured to the specimen, and then two 1.5-mm increments of composite were polymerized separately with halogen light for 40 s each. The jig was removed and specimens were stored in water at 37 °C for 24 h. After this period, Shear-bond strength tests were employed using Universal Testing Machine (Instron 4201) to measure adhesive strength in megapascals (MPa) with crosshead speed of 5 mm/min.
The force to failure was recorded for each specimen. The mean shear bond strength in MPa was determined for each group. Fractured specimens were observed with a stereomicroscope (Olympus) at 20× magnification for determination of failure modes, such as cases of cohesive, and mixed with adhesive.
Data were subjected to a one-way analysis of variance (ANOVA) to compare the effects of preparation type among the three groups. All statistical analyses were performed at p < 0.05.
2.4
Scanning electron micrographs
For electrospunned silk mats, the materials were sputter-coated with gold/palladium using a Polaron SC502 Sputter Coater (Fison Instruments, UK). Specimens were then examined using an ISI-DS-130 SEM (Avon, CT) at 15 kV. Images of representative samples were obtained once prior to bonding and once after shear-bond strength test.
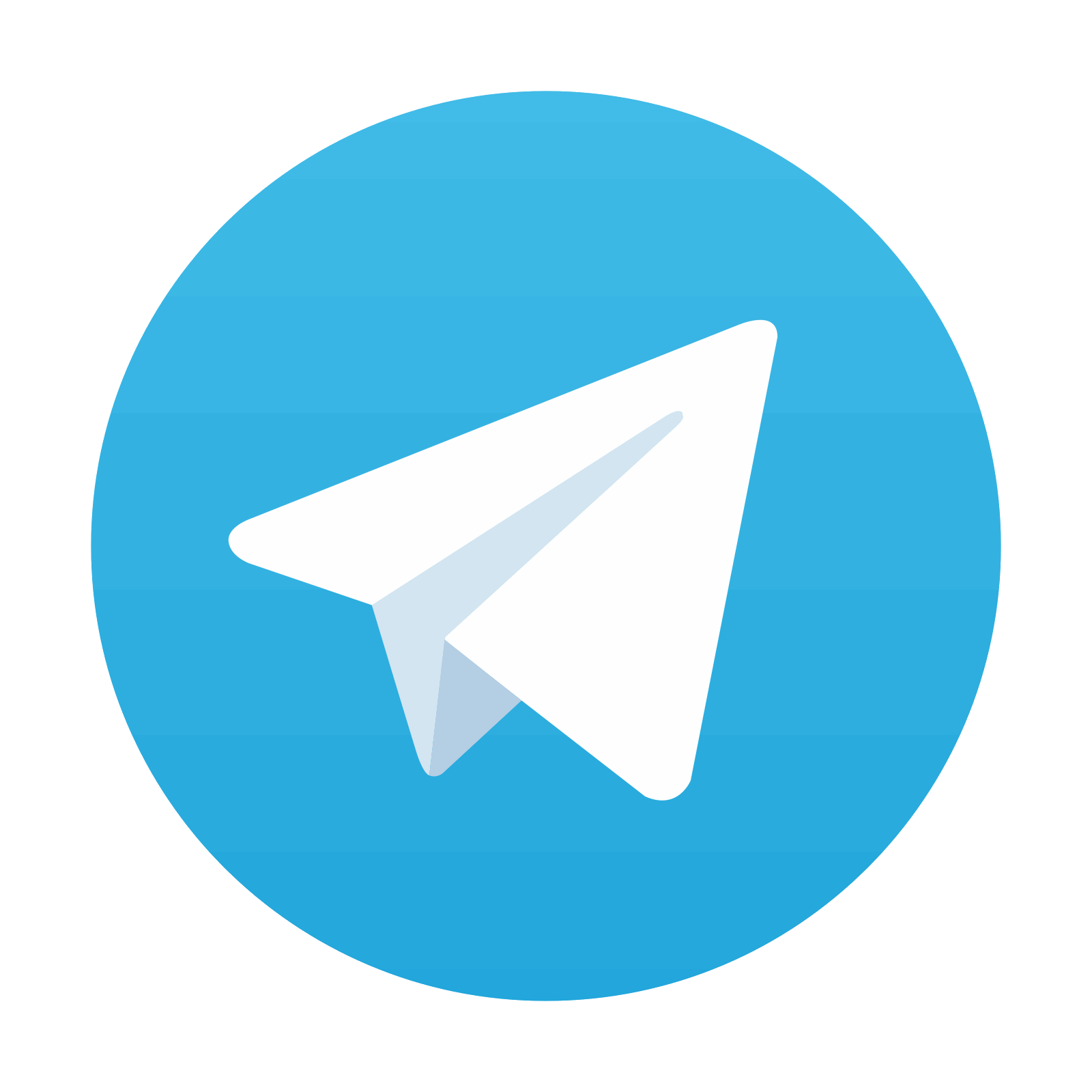
Stay updated, free dental videos. Join our Telegram channel

VIDEdental - Online dental courses
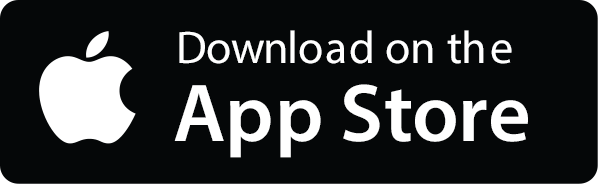
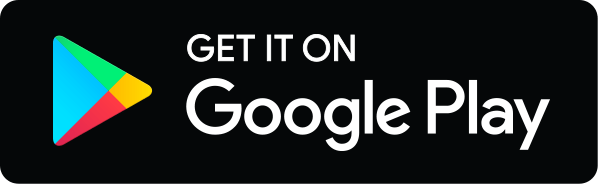