Abstract
Objective
To assess the depth of cure claims of two bulk-fill flowable RBC bases (SDR and x-tra base) using Fourier transform infrared (FTIR) spectroscopy, biaxial flexure strength (BFS), and Vickers hardness number (VHN) for specimen depths of 8 mm (in 1 mm increments).
Methods
The degree of conversion (DC) was measured by monitoring the peak height (6164 cm −1 ) of specimens (11.0 ± 0.1 mm diameter, 1.0 ± 0.1 mm thickness) during light irradiation for 20 s using a quartz tungsten halogen light curing unit at 650 ± 26 mW/cm 2 . DC was measured up to 120 s post irradiation and repeated ( n = 3) for irradiation depths up to 8 mm (in 1 mm increments). Further series ( n = 20) of eight discs were prepared, stacked, light irradiated and numbered from one to eight (distance from the LCU). The specimens were stored at 37 ± 1 °C for 24 h and BFS tested with the fracture fragments used to determine the VHN for each specimen.
Results
X-tra base can be irradiated to 8 mm without a change in DC, something the SDR material cannot claim. However, the DC results confirm both bulk-fill flowable RBC bases have a depth of cure in excess of 4 mm. One-way ANOVAs of BFS and VHN data showed significant differences between irradiation depths for x-tra base and SDR with increasing irradiation depth (4 mm) resulting in significant reductions in mean BFS and VHN.
Significance
The claims that the bulk-fill flowable RBC bases have a depth of cure in excess of 4 mm can be confirmed but the differing chemistry of the resin formulations and filler characteristics contribute to significant differences in DC, BFS and VHN data between the two materials tested.
1
Introduction
The introduction of chemically cured resin-based composites (RBCs) composed of bisphenol-A and glycidyl methacrylate (BisGMA) monomeric resin with a quartz or aluminosilicate glass filler particulate , paved the way for the beginnings of esthetic restorative dentistry. Similar to dental amalgams, the first chemically cured RBCs were placed in bulk without etching the tooth surfaces with phosphoric acid but at that time the in vivo clinical performance was discouraging when compared with dental amalgam . Chemically cured RBCs were therefore not advocated for use in large posterior restorations until the development of light irradiation technology , systems to aid adhesion to sound tooth structure and appropriate filler morphology to effectively withstand masticatory load patterns. In 1978, Dart et al. patented a visible-light irradiated RBC which when used in conjunction with ‘total etch’ adhesives developed in the 1980s truly transformed esthetic restorative dentistry. More than 30 years on, most RBC monomeric formulations contain BisGMA and/or other dimethacrylates although novel technologies such as higher molecular weight resins and hydrogenated dimer acids and novel (non-dimethacrylate) epoxy chemistries have been commercialized . Bowen’s original quartz or aluminosilicate glass fillers have been superseded by a variety of ceramic filler particle compositions often with differing filler densities, filler volume fractions, mean filler particle sizes and a general reduction in average filler particle size .
Notwithstanding the technical advances in RBC technologies since the patenting of chemically cured RBCs by Bowen in 1958 , the major disadvantages of the state-of-the-art RBCs include an insufficient depth of cure and the generation of polymerization shrinkage stress on light irradiation. This is evident, for example, in the restoration of large Class II restorations with conventional RBC materials where as a result of the insufficient depth of cure, an incremental RBC restoration technique is required . This requires either triangular-shaped or horizontal increments of maximum 2 mm thickness for the mesial approximal box, three for the distal approximal box and two for the occlusal cavity. Depending on the RBC formulation, the light irradiation time can vary from 20 to 40 s per increment, which is time consuming for the operator and an inconvenience to the patient . In addition to the increased patient treatment times, there is also an associated increased risk of air entrapment or moisture contamination between the individual RBC increments .
So-called “bulk-fill” flowable RBC bases have been recently introduced into the dental market for use beneath conventional RBC materials . These materials claim to promote light transmittance to enable the achievement of a reported depth of cure in excess of 4 mm . In the dental literature, studies investigating the clinical performance of bulk-fill flowable RBC base materials are limited . However, when bulk-fill flowable RBC base materials were placed in a single 4 mm increment, investigations on marginal quality , cuspal deflection , cuspal deflection in conjunction with microleakage and adhesion to cavity-bottom dentin have been reported. Roggendorf et al. used a Class II fatigue loading design study to establish that the marginal adaption of SDR to enamel and dentin was not influenced by the placement of a single 4 mm bulk-fill flowable RBC base compared with two separate 2 mm increments of a conventional dimethacrylate RBC. Moorthy et al. employed a twin channel deflection measuring gauge to assess the cuspal deflection of standardized large Class II cavities incrementally filled with a conventional dimethacrylate RBC or with bulk-fill flowable RBC bases. The authors established the polymerization stress generated on light irradiation of the bulk-fill flowable RBC bases was significantly lower than a conventional dimethacrylate RBC , confirming the results of Ilie and Hickel . Additionally, the cervical microleakage scores of Moorthy et al. for the teeth restored with the bulk-fill flowable RBC bases (SDR and x-tra base) confirmed the marginal integrity results reported by Roggendorf et al. . Van Ende et al. used microtensile bond strength testing to show that a high bond strength value to the cavity-bottom dentin was maintained when SDR was light irradiated to a cavity depth of 4 mm. While these studies collectively advocate the clinical use of bulk-fill flowable RBC bases, the published reports on the mechanical properties of bulk-fill flowable RBC bases which are often purported to being maintained at a depth of 4 mm are more difficult to confirm. Therefore, while these current manuscripts do add to the existing knowledge base on bulk-fill flowable RBC bases , rarely do the findings reported have any clinical significance in terms of quantifying their ‘true’ depth of cure and as a result their potential clinical efficacy.
The aim of the current study was to assess the physico-mechanical properties of two bulk-fill flowable RBC base materials through depth. The null hypothesis examined in the study was that the physico-mechanical properties of two bulk-fill flowable RBC base materials would be reduced with increased distance from the light irradiation source.
2
Materials and methods
2.1
Materials
The bulk-fill flowable RBC base materials used in the current study were x-tra base (Shade U, Lot 1204418; Voco GmbH, Cuxhaven, Germany) and SDR (Shade U, Lot 1203001032; Dentsply DeTrey GmbH, Konstanz, Germany).
2.2
Degree of conversion (DC)
Degree of conversion (DC) was measured by Fourier transform infrared spectroscopy (FTIRS; Nicolet 6700; Thermo Nicolet Instruments Co., Madison, WN, USA) using attenuated total reflection (ATR) and measuring the change in height of the peak at 1635 cm −1 corresponding with the aliphatic carbon double bond absorbance. This is facilitated by using the aromatic bond absorbance at 1608 cm −1 , as the standard control, which was not affected by the polymerization reaction . A disk-shaped nylon ring-mold (11.0 ± 0.1 mm inner diameter, 1.0 ± 0.1 mm thickness) was placed over the ATR crystal, the bulk-fill flowable RBC monomer paste was placed into the ring-mold ( Fig. 1 a ), an acetate strip positioned on top and a 1 kg load was applied for 20 s to ensure consistent specimen packing. The disk-shaped specimens were light irradiated for 20 s through a 13 mm light tip diameter, using a quartz tungsten halogen (QTH) light curing unit (LCU) operating at an output intensity of 650 ± 26 mW/cm 2 (Optilux 501, Kerr, Orange, CA, USA). The DC measurements ( n = 3) were repeated at irradiation depths up to 8 mm (in 1 mm increments) by stacking nominally identical nylon ring-molds (separated by an acetate strip) on top of each other and packing the specimens according to the procedure outlined above. The ring-molds were aligned using a custom jig and light irradiated for 20 s through the 13 mm light tip diameter using the QTH LCU ( Fig. 1 b) for specimen depths up to 8 mm (in 1 mm increments).
2.3
Biaxial flexure strength (BFS) testing
Disk-shaped specimens (11.0 ± 0.1 mm diameter, 1.0 ± 0.1 mm thickness) were manufactured by dispensing 0.28 g of the bulk-fill flowable RBC base monomer paste, measured using a balance reading to 0.001 g (Sartorius Expert; Sartorius AG, Gottingen, Germany), into a nylon ring-mold positioned on top of an acetate strip ( Fig. 1 a). A second acetate strip was placed on top of the ring-mold and a 1 kg load was applied for 20 s to ensure consistent specimen packing. The load was removed and the sample stored in a light-proof box at room temperature. The specimen preparation procedure was repeated so that a series of eight nominally identical disk-shaped specimens were prepared ( Fig. 1 b) and subsequently stored in the light-proof box. Following light irradiation, each of the eight specimens were removed from their respective ring-molds, numbered from one to eight (depending on the distance from the light tip) and stored in an incubator (Firlabo SP BVEHF, Société Firlabo, Meyzieu, France) maintained at 37 ± 1 °C for 24 h. The procedure was repeated until a group of 20 specimens numbered from one to eight (depending on the distance from the light tip) of each bulk-fill flowable RBC were prepared.
The specimens were removed from the incubator at 24 h and the BFS was determined using a universal testing machine (Instron Model 5565, High Wycombe, England) employing a 2 kN load cell and operating at a loading rate of 1 mm/min. A piece of rubber dam was placed on a 10 mm diameter knife-edge support to accommodate any specimen distortion and the specimen was loaded with the light irradiated surface uppermost and in contact with a 4 mm diameter spherical ball-indenter. The BFS (MPa) was calculated using Eq. (1)
where P was the measured load to fracture in Newtons (N), a was the radius of the knife-edge support (5 mm), v was the Poisson’s ratio for the RBC material (0.225 ) and h was the mean specimen thickness (mm) determined at the point of fracture for each fracture fragment when measured with a micrometer screw gauge reading to 1 μm (Mitutoyo, Kawasaki, Japan).
2.4
Vickers hardness number (VHN) determination
The BFS fracture fragments were used to determine the VHN of the bulk-fill flowable RBC materials. Three random indentations were introduced using a Vickers Micro-Hardness Indentor (Vickers Instruments Ltd., York, England) under a load of 300 g, applied for 15 s for specimens numbered from one to five (distance from the light tip). For distances greater than 5 mm from the LCU, measurements were made (for 15 s) under a load of 200 g (specimen numbered six), 100 g (specimen numbered seven) and 50 g (specimen numbered eight) to ensure an impression of the pyramid-shaped diamond indenter was introduced onto the RBC surface. The VHN was calculated in accordance with Eq. (2)
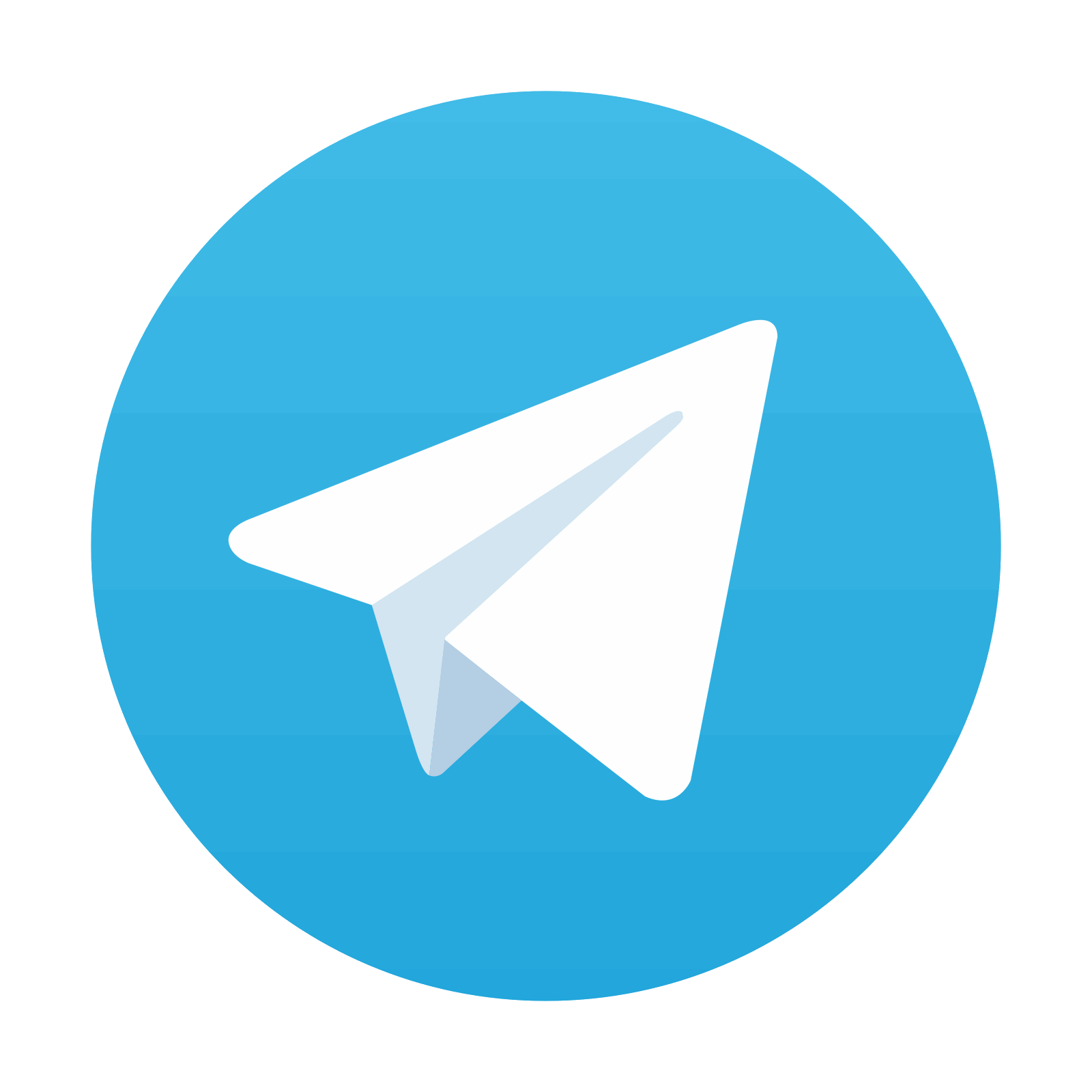
Stay updated, free dental videos. Join our Telegram channel

VIDEdental - Online dental courses
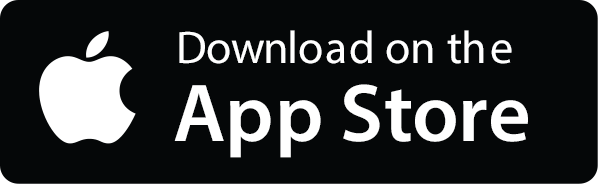
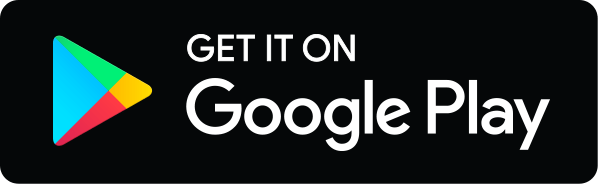