Abstract
Objectives
The purpose was to assess the influence of ferrule effect and length of cast post and cores, and FRC posts on the strength of anterior teeth.
Materials and methods
The investigations were conducted by means of finite element analysis with the application of contact elements. Thirteen 3D models of maxillary first incisors were generated: model 1 was an intact tooth; 2A – a tooth with all-ceramic crown with ferrule effect, 2B–D a teeth restored with, ferrule and FRC posts of various lengths 13 mm, 8 mm, 4 mm, 3A – a tooth with ceramic crown without, ferrule effect, 3B and C teeth without ferrule and 10 mm, 5 mm length FRC posts, 4B–D teeth with ferrule, restored by cast posts and cores of 13 mm, 8 mm, 4 mm lengths and 5B and C teeth without ferrule, restored with cast posts and cores of 10 mm, 5 mm lengths. Each model was subject to a load with a total force of 100 N uniformly distributed under the lingual cingulum, at an angle of 130°. To evaluate, the strength of tooth tissues, ceramics and composites, the modified von Mises failure criterion was used, for FRC the Tsai-Wu criterion and for cast NiCr alloy the von Mises criterion. Contact stresses were calculated at the luting cement–tissue interface.
Results
Ferrule effect in teeth reduces mvM stresses in dentin, in posts and in luting cement. The contact tensile stresses around posts in teeth with ferrule effect were 1.7–3.0 times smaller than in, teeth without ferrule. Lower mvM stresses occurred in teeth with cast posts in comparison with FRC posts. The mvM stresses in teeth with posts of various lengths were similar, irrespective of post material.
Significance
Ferrule effect in teeth with posts and cores has a critical influence on stress reduction. Using posts and cores made of rigid materials leads to stress reduction in teeth. Post length has a small, effect on stress values in tooth structures.
1
Introduction
Posts and cores are used to restore endodontically treated teeth the supragingival structures of which have suffered considerable damage and can fracture during mastication. Strength and longevity of a tooth restored with post and core depends on post material and length, root wall width and length, good attachment of the post to tissues, presence of ferrule and tooth load .
Ferrule effect – the effect of a crown encircling remaining supragingival tooth structure – considerably improves tooth resistance to fracture . Most authors demonstrate a positive influence of ferrule effect on the strength of teeth restored with posts . In addition, ferrule effect increases the post/core ratio and prevents the luting cement from being washed away, and in turn improves post retention. The height of the remaining tooth structure is of secondary significance , as is the location of supragingival structures . However, Nauman et al. and Tan et al. hold contrary opinions. According to Ng et al. , maintaining coronal dentin on the palatal aspect in upper incisors increases their resistance to fracture 2 times, maintaining coronal dentin on the lingual aspect increases the resistance considerably less.
Most commonly recommended at present is the use of custom cast metal posts and cores or standard prefabricated fiberglass-reinforced composite (FRC) posts . FRC posts have different biomechanical properties to metal posts. Gold-alloy cast and FRC posts have, respectively, 7 times (1542 MPa) and 4 times (879 MPa) the flexural strength of dentin (213 MPa) .
Many years of experience and strength testing have allowed the optimum dimensions of cast metal posts and cores to be determined. The length of custom metal posts should constitute 2/3–3/4 of root length and be at least equal to the length of the future crown . In order to protect periapical tissues, 3 mm of apical canal filling is maintained. Deep root preparation removes a considerable amount of its structure and involves the risk of root perforation . However, short posts are associated with considerable stresses in dentin around their apex , which may lead to debonding or root fracture. According to some studies, the FRC post/root length ratio should be equal to that in metal posts. Some authors suggest that due to adhesive bonding and good bonding to dentin these posts can be shorter .
The purpose was to assess the influence of ferrule effect and length of cast and FRC posts on the stresses of anterior teeth.
2
Materials and methods
Scans of the surface of the maxillary first left incisor were made with the laser Dental 3D Scanner D250 (3ShapeA/S, Copenhagen, Denmark). The scans were processed with 3Shape Dental Designer CAD software. Files with the PTS extension, including coordinates of the points on the surfaces of the tooth under analysis, were introduced into FEA software – ANSYS 14 (ANSYS version 14, ANSYS Inc., Canonsburg, PA, USA) . In addition, a CT of the tooth being investigated was made with a GXCB-500/i-CAT (Gendex Dental Systems, Des Plaines, IL, USA) system. The points on the tooth surface (obtained from the scanner) and the points on the enamel–dentin–pulp junction (obtained from the CT), in horizontal layers (every 1 mm), were introduced into the code preprocessor. The points were connected with splines and on this basis the tooth cross-section areas were reconstructed. The connection of these crosswise cross-section areas allowed us the construction of a solid model of the central incisor, divided into enamel, dentin and pulp. The size and shape of the tooth were in accordance with the data from the anatomical atlas . The crown was 10.5 mm long, root length was 13 mm, bocco-lingual diameter of crown was 7 mm. A 0.2 mm-thick periodontal ligament was modeled around the tooth root (model 1). The tooth model was located in the system of coordinates such that the Z axis was parallel to the long axis of the tooth, the X axis was oriented mesiodistally and the Y axis was oriented buccolingually.
The tooth was prepared for a ceramic crown in accordance with standard clinical recommendations . 2.5 mm of the incisal edge was removed and a 0.8 mm-wide rounded shoulder was prepared at the gingival margin. The crown was scanned with the Dental 3D Scanner D250 (3ShapeA/S, Copenhagen, Denmark). A cloud of points was introduced into the ANSYS code and on this basis a solid representing the prepared tooth crown was developed. An additional 0.1 mm-thick layer was added under the prepared crown to simulate the cement layer. The solid was added to model 1, thereby constructing an incisor with an all-ceramic crown with ferrule (model 2A) ( Fig. 1 a).

The tooth model 2A was sectioned perpendicular to the longitudinal axis, at a distance of 3.5 mm from the cemento-enamel junction ( Fig. 2 a). In the ANSYS code preprocessor, a 17 mm × 1.2 mm cylinder with a truncated cone at the end was generated. This solid was introduced into the root canal and then added to the model 2A ( Fig. 2 a). The length 13 mm of the cylinder was introduced into root and 4 mm into crown, measured from catting plane. A 0.1 mm-thick layer was added around the root part of post to simulate the cement layer. In this way, a tooth model 2B was created with a 13 mm-long standard post (in relation to catting plane) and a prosthetic crown with ferrule effect ( Fig. 1 b). Then, the post in root was catted from 13 mm to 8 mm and 4 mm. Similarly, tooth models were developed with a medium 8 mm-long (model 2C) ( Fig. 1 c) and short 4 mm-long FRC post (model 2D) ( Fig. 1 d). The lengths of the long, medium and short posts were, respectively, about 80%, 50% and 25% of root length.

Next, the coronal dentin of model 2A was sectioned perpendicular to the longitudinal axis, at the tooth cervix. Tooth models with the crowns without ferrule effect and cores beginning at the level of the gingiva were developed (model 3A) ( Figs. 1g and 2b ). The cylinder of the same size 17 mm × 1.2 mm was introduced into the model 3A. The length 10 mm was introduced into the root and 7 mm into the crown, measured from catting plane ( Fig. 2 b). In this way, models with a long FRC post – 10 mm (model 3B) ( Fig. 1 e) and a short post – 5 mm (model 3C) ( Fig. 1 f) were generated. In the models without ferrule effect, the lengths of the long and short posts accounted for 77% and 38% of root length, respectively.
It was assumed that the standard posts were made of fiberglass-reinforced composite FRC (models 2 and 3) ( Fig. 1 b–f) and that the individual posts were made of NiCr alloy (models 4 and 5) ( Fig. 1 h–l). The cores of the FRC posts were composite, while in the cast posts these were made of metal and integrated with the post. The crown was modeled from leucite ceramic. Posts and crowns were ideally bonded to the tooth structure with Variolink II composite resin cement (Ivoclar, Vivadent AG, Schaan, Lichtenstein). The values for Young’s modulus, Poisson’s ratio were entered for enamel , dentin , periodontium , NiCr alloy , FRC posts (Young’s modulus along the long axis being 37 GPa, and in the perpendicular direction 9.5 GPa) , luting composite resin cement , core composite resin , and ceramic . Data are presented in Table 1 . The models were considered to be linear, elastic, homogeneous and isotropic (with the exception of the FRC post), but had variable compressive and tensile strengths. Tensile and compressive strength was assumed for enamel (11.5 MPa , 384 MPa ), dentin (105.5 MPa , 297 MPa ), NiCr alloy (710 MPa) , FRC post (1200/35 MPa, 910/110 MPa) , core composite resin (41 MPa, 293 MPa) , ceramic (48.8 MPa, 162.9 MPa) and luting composite resin cement (45.1 MPa, 178 MPa) .
Material | Modulus of elasticity (GPa) | Poisson ratio | Tensile strength (MPa) | Compressive strength (MPa) |
---|---|---|---|---|
Enamel | 84.1 | 0.33 | 11.5 | 384 |
Dentin | 18.6 | 0.31 | 105.5 | 297 |
Periodontium | 0.05 | 0.45 | ||
Cast NiCr post | 188 | 0.33 | 710 | 710 |
Glass fiber post | E X = 37 E Y = 9.5 E Z = 9.5 |
ν X = 0.34 ν Y = 0.27 ν Z = 0.27 |
R tX = 1200 R tY = 35 R tZ = 35 |
R cX = 910 R cY = 110 R cZ = 110 |
Crowns leucite ceramic | 65.0 | 0.19 | 48.8 | 162.9 |
Composite core | 14.1 | 0.24 | 41 | 293 |
Luting resin cement | 8.3 | 0.35 | 45.1 | 178 |
For calculation purposes, each tooth model was divided into 10-node structural solid elements (Solid 187). Used in each of the thirteen tooth models were approximately 120,000 elements joined at 150,000 nodes. Pairs of bonded contact elements, Targe 170 and Conta 174, were used around the posts, at the interface of the luting cement–dentin bond and under the crown.
The models were fixed in nodes on the outer surface of the periodontium and subjected to loads simulating forces acting on incisors during clenching in central occlusion. Their total value was 100 N and they were applied evenly to the nodes under the lingual cingulum, at 130° to the longitudinal axis .
The contact simulation conducted with finite element analysis is a nonlinear analysis and therefore requires that the load be divided into steps. The components of stresses (normal, shear and principal stresses) in the models during oblique loading were calculated. The use of von Mises’ theory for material failure requires that the compressive and tensile strengths be equal, whereas for ceramic, tooth tissues, composite resin the compressive strength values are several times larger than the tensile strength values. One criterion used to assess the failure of such brittle materials under complex stress conditions is the modified von Mises failure criterion (mvM) . This considers the ratio between compressive and tensile strength (enamel 33.4; dentin 2.8; leucite ceramic 3.3; composite resin cement 3.9). The mvM stresses were calculated from principal stresses in models, using the original computers programme in APDL (Ansys Parametric Design Language). According to the criterion, the material will fail when the values of the equivalent stresses exceed its tensile strength. The maximum values of the equivalent stresses which appeared in the model materials were compared to one another and to their respective tensile strengths. To evaluate effort for FRC posts, which have strong anisotropic properties, the Tsai-Wu criterion was applied . The inverse Tsai-Wu ratio index (STWSR) was estimated and the index values above 1 indicate material damage. The results of the calculation are presented as maps of these stresses in the dentin, post and cores, luting cement and crowns of the incisor models. Compressive, tensile, and shear contact stresses at the luting cement–dentin interface around posts and under the crown were also calculated. These were presented graphically as maps on the contact surfaces of the restoration and dentin.
2
Materials and methods
Scans of the surface of the maxillary first left incisor were made with the laser Dental 3D Scanner D250 (3ShapeA/S, Copenhagen, Denmark). The scans were processed with 3Shape Dental Designer CAD software. Files with the PTS extension, including coordinates of the points on the surfaces of the tooth under analysis, were introduced into FEA software – ANSYS 14 (ANSYS version 14, ANSYS Inc., Canonsburg, PA, USA) . In addition, a CT of the tooth being investigated was made with a GXCB-500/i-CAT (Gendex Dental Systems, Des Plaines, IL, USA) system. The points on the tooth surface (obtained from the scanner) and the points on the enamel–dentin–pulp junction (obtained from the CT), in horizontal layers (every 1 mm), were introduced into the code preprocessor. The points were connected with splines and on this basis the tooth cross-section areas were reconstructed. The connection of these crosswise cross-section areas allowed us the construction of a solid model of the central incisor, divided into enamel, dentin and pulp. The size and shape of the tooth were in accordance with the data from the anatomical atlas . The crown was 10.5 mm long, root length was 13 mm, bocco-lingual diameter of crown was 7 mm. A 0.2 mm-thick periodontal ligament was modeled around the tooth root (model 1). The tooth model was located in the system of coordinates such that the Z axis was parallel to the long axis of the tooth, the X axis was oriented mesiodistally and the Y axis was oriented buccolingually.
The tooth was prepared for a ceramic crown in accordance with standard clinical recommendations . 2.5 mm of the incisal edge was removed and a 0.8 mm-wide rounded shoulder was prepared at the gingival margin. The crown was scanned with the Dental 3D Scanner D250 (3ShapeA/S, Copenhagen, Denmark). A cloud of points was introduced into the ANSYS code and on this basis a solid representing the prepared tooth crown was developed. An additional 0.1 mm-thick layer was added under the prepared crown to simulate the cement layer. The solid was added to model 1, thereby constructing an incisor with an all-ceramic crown with ferrule (model 2A) ( Fig. 1 a).
The tooth model 2A was sectioned perpendicular to the longitudinal axis, at a distance of 3.5 mm from the cemento-enamel junction ( Fig. 2 a). In the ANSYS code preprocessor, a 17 mm × 1.2 mm cylinder with a truncated cone at the end was generated. This solid was introduced into the root canal and then added to the model 2A ( Fig. 2 a). The length 13 mm of the cylinder was introduced into root and 4 mm into crown, measured from catting plane. A 0.1 mm-thick layer was added around the root part of post to simulate the cement layer. In this way, a tooth model 2B was created with a 13 mm-long standard post (in relation to catting plane) and a prosthetic crown with ferrule effect ( Fig. 1 b). Then, the post in root was catted from 13 mm to 8 mm and 4 mm. Similarly, tooth models were developed with a medium 8 mm-long (model 2C) ( Fig. 1 c) and short 4 mm-long FRC post (model 2D) ( Fig. 1 d). The lengths of the long, medium and short posts were, respectively, about 80%, 50% and 25% of root length.
Next, the coronal dentin of model 2A was sectioned perpendicular to the longitudinal axis, at the tooth cervix. Tooth models with the crowns without ferrule effect and cores beginning at the level of the gingiva were developed (model 3A) ( Figs. 1g and 2b ). The cylinder of the same size 17 mm × 1.2 mm was introduced into the model 3A. The length 10 mm was introduced into the root and 7 mm into the crown, measured from catting plane ( Fig. 2 b). In this way, models with a long FRC post – 10 mm (model 3B) ( Fig. 1 e) and a short post – 5 mm (model 3C) ( Fig. 1 f) were generated. In the models without ferrule effect, the lengths of the long and short posts accounted for 77% and 38% of root length, respectively.
It was assumed that the standard posts were made of fiberglass-reinforced composite FRC (models 2 and 3) ( Fig. 1 b–f) and that the individual posts were made of NiCr alloy (models 4 and 5) ( Fig. 1 h–l). The cores of the FRC posts were composite, while in the cast posts these were made of metal and integrated with the post. The crown was modeled from leucite ceramic. Posts and crowns were ideally bonded to the tooth structure with Variolink II composite resin cement (Ivoclar, Vivadent AG, Schaan, Lichtenstein). The values for Young’s modulus, Poisson’s ratio were entered for enamel , dentin , periodontium , NiCr alloy , FRC posts (Young’s modulus along the long axis being 37 GPa, and in the perpendicular direction 9.5 GPa) , luting composite resin cement , core composite resin , and ceramic . Data are presented in Table 1 . The models were considered to be linear, elastic, homogeneous and isotropic (with the exception of the FRC post), but had variable compressive and tensile strengths. Tensile and compressive strength was assumed for enamel (11.5 MPa , 384 MPa ), dentin (105.5 MPa , 297 MPa ), NiCr alloy (710 MPa) , FRC post (1200/35 MPa, 910/110 MPa) , core composite resin (41 MPa, 293 MPa) , ceramic (48.8 MPa, 162.9 MPa) and luting composite resin cement (45.1 MPa, 178 MPa) .
Material | Modulus of elasticity (GPa) | Poisson ratio | Tensile strength (MPa) | Compressive strength (MPa) |
---|---|---|---|---|
Enamel | 84.1 | 0.33 | 11.5 | 384 |
Dentin | 18.6 | 0.31 | 105.5 | 297 |
Periodontium | 0.05 | 0.45 | ||
Cast NiCr post | 188 | 0.33 | 710 | 710 |
Glass fiber post | E X = 37 E Y = 9.5 E Z = 9.5 |
ν X = 0.34 ν Y = 0.27 ν Z = 0.27 |
R tX = 1200 R tY = 35 R tZ = 35 |
R cX = 910 R cY = 110 R cZ = 110 |
Crowns leucite ceramic | 65.0 | 0.19 | 48.8 | 162.9 |
Composite core | 14.1 | 0.24 | 41 | 293 |
Luting resin cement | 8.3 | 0.35 | 45.1 | 178 |
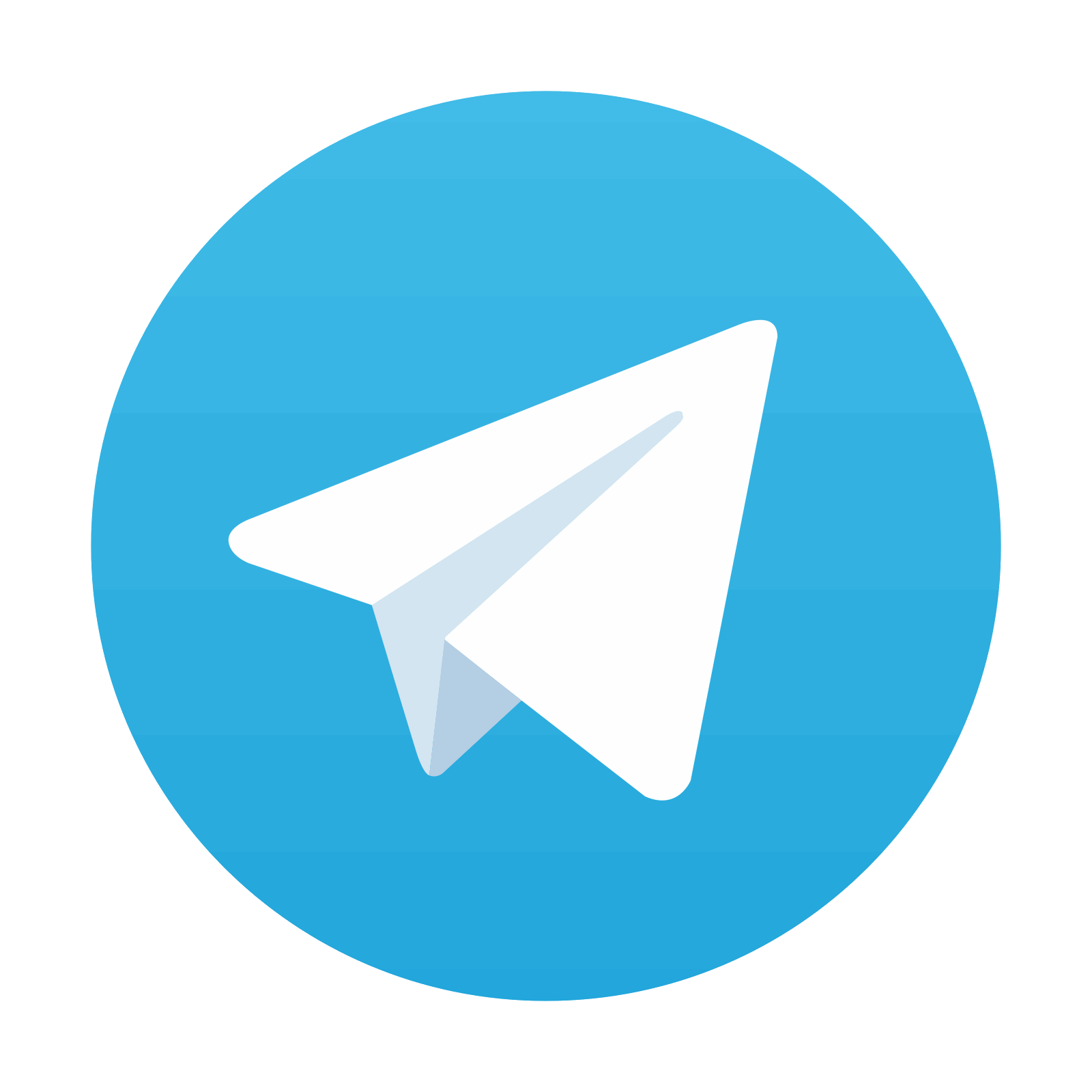
Stay updated, free dental videos. Join our Telegram channel

VIDEdental - Online dental courses
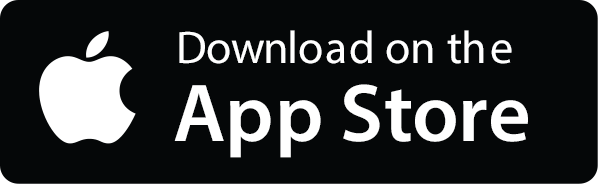
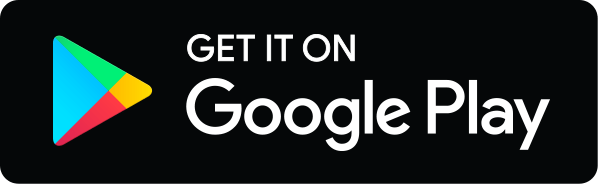
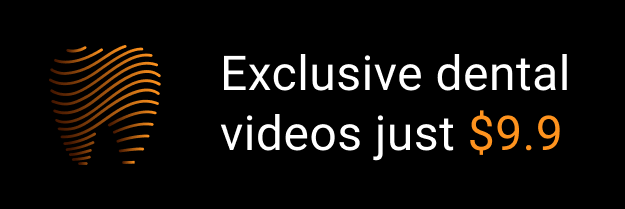