1
The Importance of Maintaining Pulp Vitality
Bruno Cavalcanti and Daniel Chiego, Jr.
Department of Cariology, Restorative Sciences and Endodontics, University of Michigan School of Dentistry, Ann Arbor, MI, USA
Introduction
When the pulp is inflamed, either reversibly or irreversibly, it is important for clinicians to question the success rates, possible outcomes and patient satisfaction associated with each treatment option. This is also true when considering using more conservative options like vital pulp treatment (VPT) or traditional therapies such as root canal treatment. As highlighted elsewhere in this book, both have a good success rate and can be used as reliable options for the management of pulpal disease.
In this context, one question commonly asked by clinicians is ‘what are the real benefits of maintaining the pulp?’ It is well‐known that immature teeth benefit considerably from VPT. However, clinicians may not see the importance of keeping the pulp tissue for other cases, as the only perceived benefit would be the maintenance of tooth sensitivity. While this benefit is correct, the pulp plays other important roles within the tooth, most notably with the molecular mechanisms to defend the body from bacterial challenge. Furthermore, with the fast development of regenerative therapies, the pulp will be essential to rebuild lost tooth structures and improve the tooth’s prognosis. This book chapter will show a number of molecular mechanisms that are involved in this regenerative process, how the pulp physiology contributes to clinical practice and, most important, show the importance of the pulp tissue in the maintenance of tooth homeostasis.
Dental Pulp Development
To appreciate the importance that the pulp plays on tooth homeostasis, it is necessary to appreciate how the dentine‐pulp complex develops. The developmental stages bring a number of insights into the participation of different cell types and the subsequent organization of dental tissues, which can also be recapitulated into the desired repair and regeneration processes after dental procedures.
Tooth development is a process that involves a well‐coordinated effort between cells from different origins, leading to the formation of a complex organ (detailed in Chapter 2). Unfortunately, when analysing pulp repair/regeneration processes, the clinician is not able to mimic whole tooth formation. This is complicated further by the cells present in the pulp tissue that may not have the same potential as the original cells during tooth development. Nonetheless, the translation of the process at the molecular level to the clinical activity can be of great importance to direct future studies and biomaterial development.
In this context, the participation of stem cells in the development process is important. It is now well established that the pulp tissue has its own reserve of multipotent cells, capable of differentiating into the necessary tissues and/or replacing cells that were damaged or simply completed their function and enter into senescence (1). In fact, most dental tissues have their own mesenchymal stem cells; the enamel, for obvious reasons, is deprived of any cells after tooth eruption and, thus, does not have the same regenerative potential as other tissues. The discovery of dental pulp stem cells (DPSCs) brought many possibilities, more specifically in the understanding of the success of VPT and regenerative endodontics. DPSCs are capable of differentiation into a myriad of cells and tissues, including odontoblasts, fibroblasts, endothelial cells and neurons (2–4), being capable of regenerating all cell types necessary to form a new pulp tissue. This potential appears superior to responses observed with other stem cell populations (3). In addition, the literature shows, in animal models, that the neoformed pulp tissue in complete root models is very similar to the original pulp tissue (5, 6) (Figure 1.1). Moreover, these new pulp tissues were induced only in the presence of the host vasculature and DPSCs, suggesting that the potential is there for complete regeneration or at least functional repair.
Cell Signalling and Dental Pulp Development
Most studies using DPSCs have focused on the potential of these cells to differentiate into odontoblasts (7–9). This is understandable as, in the reparative process, clinicians want the neoformed pulp tissue to produce dentine in order for this barrier to serve as protection to the tissue. Understanding odontoblast differentiation is a difficult process: some studies have shown that the reparative dentine, particularly when induced by certain materials, can be tubular (10–12); other studies have shown that, most of the time, the barrier does not have the tubular aspect, meaning that the formed tissue is more akin to ‘osteodentine’ than to actual dentine (13, 14). This brings uncertainty, as there is no specific odontoblast marker(s) to confirm that the differentiated stem cell is a real odontoblast. Studies have looked into dentin‐matrix‐acidic‐phoshoprotein 1 (DMP‐1), dentin‐sialophosphoprotein (DSPP), matrix extracellular phosphoglycoprotein (MEPE), nestin and others, generally trying to observe their presence in combination with each other (2, 7, 15–17). However, it is important to emphasize that some of these genes can be expressed by osteoblasts and other cell types, meaning that the differentiated cells observed in molecular analysis may not be a typical odontoblast cell.
With this, it is possible to separate the two concepts of regeneration and repair: from a clinical standpoint, having an adequate regeneration/repair with a solid dentine (or osteodentine) barrier, pulp vitality and functional tooth is good enough for patient satisfaction. On the other hand, from a research standpoint, it can be frustrating that with all the potential observed from the stem cells, we are still not able to regenerate the tissues as they are, complete with an odontoblast pseudostratified layer, cell junctions and tubular dentine.
Some answers for this dichotomy may be due to a shortage of information on molecular signalling. As discussed above, during tooth development, there is a very well‐coordinated effort and interaction between different cell types and layers to induce tissue formation and even tooth shape (18, 19). For example, it is known that the signalling between the ameloblasts and mesenchymal cells in the dental papilla induces the initial cell differentiation towards odontoblasts, which consequently directs the deposition of an initially ‘disorganized’ layer (the mantle dentine) and then starts the construction of the typical tubular pattern of orthodentine, by a centripetal direction of movement of the odontoblast layer (Figure 1.2). This signalling is comprised principally of DSPP, enamelysin and ameloblastin (20). However, it is evident that the Wnt and Sonic hedgehog (SHH) pathways and the deposition of a baseline collagen matrix are also essential for the correct cell lining (18, 21). With this, attempts were made to use enamel derivative proteins to induce pulp repair. However, there are differences between the differentiation induced by enamel matrix derivatives (EMD) and mineral trioxide aggregate (MTA), for example, even if the gene expression changes are similar (Figure 1.3). Clinically, none of the materials used for pulp capping and/or regenerative procedures is capable of synthesizing and secreting the original signalling molecules layered by ameloblasts. This does not mean that the regeneration process is impossible. The literature has shown examples that the dentine itself is a major reservoir of multiple growth factors that can activate multiple signalling pathways into DPSCs (22). More importantly, these growth factors can be mobilized by current materials used for pulp capping (e.g. calcium hydroxide and MTA), which can be of great importance in chemotaxis prior to inducing cell differentiation and production of the dentine barrier (23–25).

Figure 1.1 Dental pulp tissue engineering with SHED injected into human root canals and transplanted into immunodeficient mice. (a) Low‐magnification and (b) high‐magnification images of tissues formed when SHED mixed with scaffolds (Puramatrix™, rhCollagen type I groups) were injected into full‐length root canals of human premolars. A vascularized connective tissue occupied the full extension of the root canal. Cell densification and many blood vessels were observed along dentin walls. Scaffolds (Puramatrix™) injected into the root canals without cells were used as controls for SHED. Freshly extracted human premolars were used as tissue controls. Black arrows point to blood vessels close to the odontoblastic layer. (c) Graph depicting microvessel density and (d) cellular density of dental pulp tissues engineered with SHED injected into full human root canals. Microvessel density and cellular density were similar in both experimental conditions and the control group (human pulp), as determined by one‐way ANOVA (5). Reproduced with permission from International & American Associations for Dental Research
.

Figure 1.2 Sequence of dentine formation – (1) signalling from pre‐ameloblasts to pre‐odontoblasts (DSPP, enamelysin and ameloblastin) and deposition of a baseline collagen matrix; (2) deposition of pre‐dentine by the immature odontoblasts, leading to signalling to ameloblasts; (3) enamel deposition; (4) dentin (tubular deposition). Both ameloblasts and odontoblasts mature throughout the process.

Figure 1.3 Western blotting analysis of pathways affected by BMP‐2 (a known dentine inducer) and EMD (enamel matrix derivatives) on dental pulp stem cells. Differences can be seen by the increase of expressed Wnt pathway proteins for EMD (a) and the increase of Smad 4 for BMP‐2 in the canonical BMP pathway (b). Unpublished data.
The Role of the Apical Papilla
Last, but not least in tooth development, is the role of the apical papilla. While considered an embryonic tissue, the apical papilla is a mesenchymal tissue that remains active until the complete maturation of the tooth (Figure 1.4). After the deposition of the first layers of dentine at the crown level and subsequent formation of the primitive pulp tissue, the remnants of the dental papilla continue to work with the Hertwig’s epithelial root sheath in order to guide the development of the cementum, alveolar bone, radicular dentine and periodontal ligament (26, 27). As with the crown, the signalling between epithelial and mesenchymal cells is responsible for tissue differentiation of the stem cells present in this tissue and consequent dentinogenesis. Even after the tooth eruption, the root(s) will continue to develop and, until the development is complete, the apical papilla will remain. As the original dental papilla, the apical papilla is rich in undifferentiated cells with multipotent capacity, which makes it a great source of stem cells for revitalization or revascularization procedures (28).

Figure 1.4 Histologic image from the apical papilla, detaching from the root apex of an immature third molar. Observe the high cellularity and vascularization of the tissue, which can be preserved partially and used for endodontic regenerative purposes (HE, 40×).
This understanding of the tooth development process brings insight into the repair process induced by VPTs. The first associated with the presence of undifferentiated cells and its potential to differentiate into odontoblasts and other cell types necessary for repair and regeneration. Second, it is expected that an effective material will closely mimic the interactions between these mesenchymal stem cells and the epithelial cells that formerly underlined the primitive pulp tissue. Third, molecules that participate not only in the differentiation process but also in the cell recruiting events may be of interest for using dental materials. This can happen directly or indirectly as dentine serves as a reservoir for many of these factors, and materials can be affected by extracting them from the tissue and mobilizing them for cell intake.
Stem Cell Reserve and Healing/Regeneration Potential of the Dental Pulp
Current concepts on pulp regeneration rely on stem cells, either by transplantation or by attracting stem cells present in the body to the area, also known as cell homing. The same can be said about the repair process: it is known that the pulp has a reparative potential, and most of this potential comes from the cellularity of the tissue. Notably, previous research has shown that older patients present lower healing potential in the pulp, and that conservative pulp procedures should be mostly reserved for young patients. This comes from the fact that older connective tissues will have their cell turnover and cellularity negatively affected, and the constant production of secondary dentine determines that cells in this ‘older’ pulp tissue may enter senescence (29, 30). The aged pulp tissue will appear radiographically to have smaller pulp chambers and clinically have less profuse bleeding and higher levels of fibrosis. This means that a tooth that has been previously decayed with tertiary dentine production and then restored is pathologically ‘older’ than a completely sound tooth. It is important to differentiate this pulp age from the patient’s age. With this, the literature has shown that the patient’s age has little effect on the success of VPT procedures (31).
Of course, a clinical evaluation of the cellularity of the pulp tissue is not possible currently; in fact, dentistry, despite all the evolution in the last century, still does not have a reliable way to determine an exact pulp diagnosis (see Chapter 3). Thus, the professional opting for a conservative or radical procedure needs to take other factors into account besides the patient’s age. Indeed, the patient’s age still plays a role as the fibre: cell ratio changes over time. But other factors such as clinical diagnosis, dental history and presence of tertiary dentine and/or internal calcifications are also signs that the pulp tissue in question has already been irritated and responded. Having a clinical understanding that an aged tissue can respond less ideally in a young patient and vice versa is essential to explain both success and failure in VPT.
Pulp Vascularization and its Clinical Implications
As with most connective tissues, the dental pulp can be seen as comprised of structural fibres, cells, nerves, blood vessels and lymphatic vessels. However, when looking closely, it is clear that the pulp is more complex; it is made up of different anatomical zones and is encircled by a hard tissue secreted by odontoblasts (Figure 1.5). The impact of these anatomical attributes and other factors on the potential of the pulp to heal and/or on the determination of success in clinical procedures will be discussed further below.
Among these complexities, the internal anatomy of the pulp chamber and canals is one of the factors contributing to the evolution of inflammatory and reparative processes. As stated above, the pulp is surrounded by dentine, which is a hard tissue. This makes it impossible for the pulp tissue to swell and/or expand in case of inflammation. The apical foramen is the main point of entry for the neurovascular bundles, and the more immature the tooth is, meaning that the foramen is increased in size (open‐apex), the better the tissue vascularization is. This happens even in inflammatory events and, thus, can result in better clinical outcomes when performing pulp capping and/or pulpotomy procedures. In addition, due to their small size and inconsistent numbers, lateral canals, for example, do not appear to contribute significantly to the presence of neurovascular bundles.
If clinical outcome and success of VPTs is considered, the presence of vascularization is important, as it is responsible for providing the immune cell response and the nutrients for a successful repair. It is known that angiogenesis can be induced by the presence of hypoxia, which is observed in a connective tissue that achieves 200 μm in size (32). The dental papilla does not have any blood vessels until it achieves this diameter, and cells start to suffer from the lack of oxygen. This lack of oxygen induces the production of hypoxia‐inducible transcription factor 1 – alpha (HIF1‐alpha), which then induces the activation of vascular endothelial growth factor (VEGF), responsible for cell differentiation and formation of new blood vessels (33, 34). This factor is important and explains why the coronal pulp is much richer in microvessels per mm3 than the radicular pulp, as its volume is larger. Moreover, HIF‐1alpha has been connected to increased production of tertiary dentine in inflamed pulps, suggesting these factors can also contribute to the repair process (33, 35). It is essential to understand the role that hypoxia plays, not only in pulp development but also in other aspects of the homeostasis of the tissue. After any sort of damage and/or induced inflammatory process by the presence of bacterial lipopolysaccharides (LPS), it is possible that small areas of the pulp tissue will undergo different levels of hypoxia. The lack of oxygen in this tissue has the primary participation in the angiogenic process, particularly by the expression of HIF1‐alpha and VEGF (Figure 1.6). Other molecules are also related to angiogenesis, more specifically the B‐cell lymphoma 2 (Bcl‐2) (36) and cytokines such as interleukin 1 beta (IL‐1beta) and tumour necrosis factor‐alpha (TNF‐alpha) (37), which all have a clear effect on both the angiogenic and inflammatory processes. Moreover, the presence of hypoxia is known to activate DPSC differentiation and consequent mineralization (35, 38, 39), illustrating the close connection between the pulp damage and the repair process.

Figure 1.5 Histologic image from the pulp tissue encircled by dentine/enamel structure (HE, 40×).
In addition to the simple presence of blood vessels, it is well‐known that a vascularized tissue can also respond more effectively to harm from bacteria and materials in order that the tissue can be repaired or regenerated properly. Many studies on pulp regeneration have been focusing on the importance of vascularization and have specifically shown that, without proper angiogenesis, true regeneration cannot occur. In this context, the use of angiogenic factors such as the above‐cited HIF1‐alpha or the VEGF has been proven as a way to promote angiogenesis by the differentiation of DPSC into endothelial cells and by assisting with anastomosis with the host original blood vessels (2, 33). This process is essential to allow for the complete fill of the pulp space with functional tissue and, in the case of repair, VEGF, for example, could be used as an adjuvant to promote adequate oxygen levels for the pulp tissue during the initial inflammatory process and, consequently, allowing the cells to migrate and differentiate.
Besides blood vessels, lymphatic vessels appear to be of major importance in regulating inflammatory and repair processes. While the literature has opposing views on this subject, with data showing the complete absence of this type of structure (40, 41) and others showing its presence (42–45
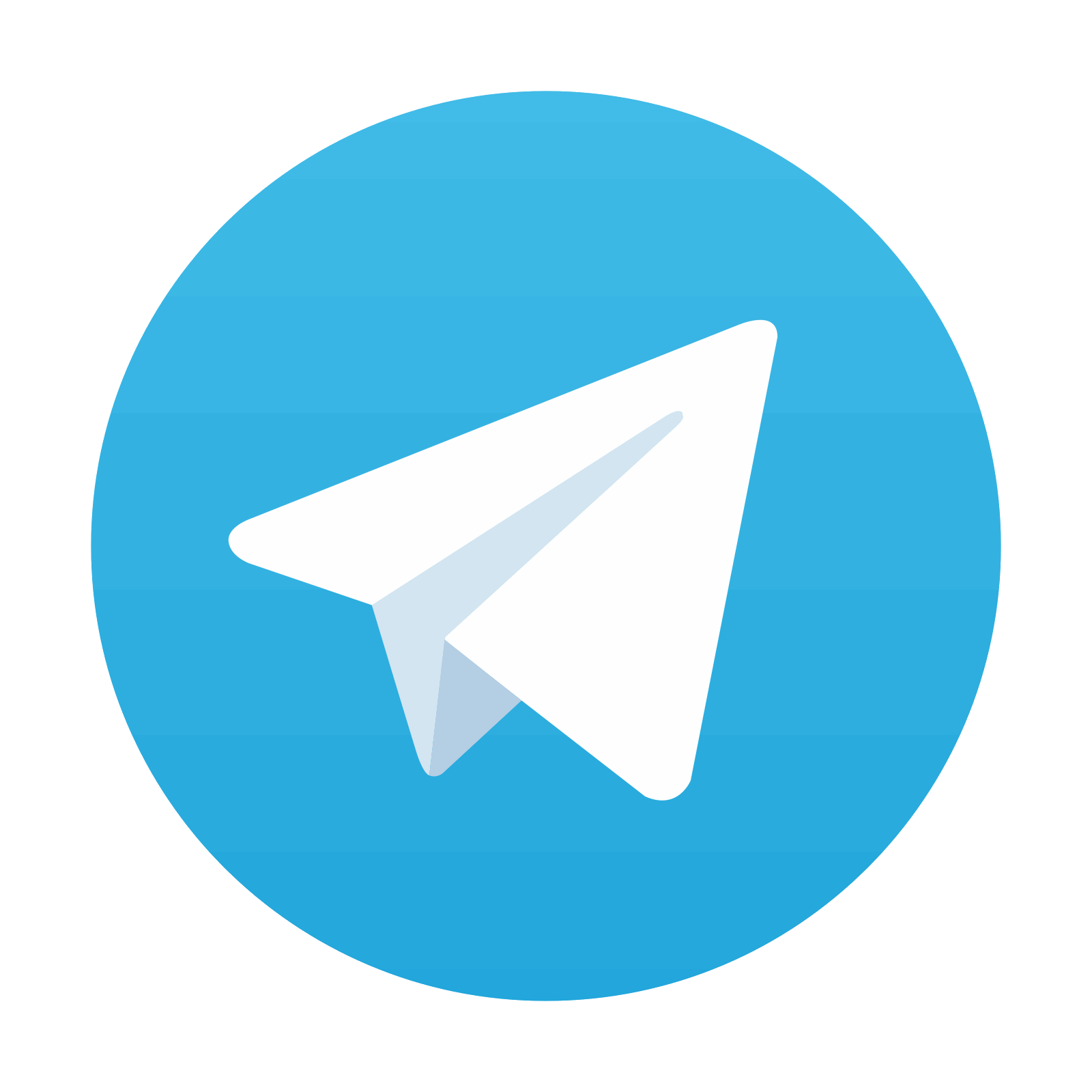
Stay updated, free dental videos. Join our Telegram channel

VIDEdental - Online dental courses
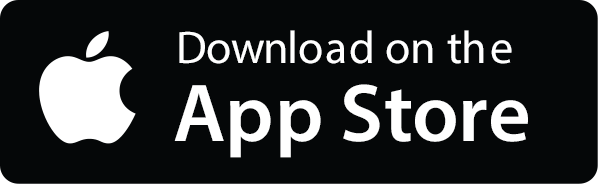
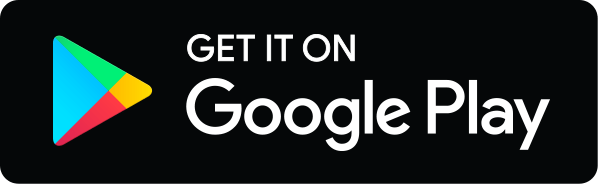