Abstract
Information concerning the effects of the implant cutting flute design on initial stability and its influence on osseointegration in vivo is limited. This study evaluated the early effects of implants with a specific cutting flute design placed in the sheep mandible. Forty-eight dental implants with two different macro-geometries (24 with a specific cutting flute design – Blossom group; 24 with a self-tapping design – DT group) were inserted into the mandibular bodies of six sheep; the maximum insertion torque was recorded. Samples were retrieved and processed for histomorphometric analysis after 3 and 6 weeks. The mean insertion torque was lower for Blossom implants ( P < 0.001). No differences in histomorphometric results were observed between the groups. At 3 weeks, P = 0.58 for bone-to-implant contact (BIC) and P = 0.52 for bone area fraction occupied (BAFO); at 6 weeks, P = 0.55 for BIC and P = 0.45 for BAFO. While no histomorphometric differences were observed, ground sections showed different healing patterns between the implants, with better peri-implant bone organization around those with the specific cutting flute design (Blossom group). Implants with the modified cutting flute design had a significantly reduced insertion torque compared to the DT implants with a traditional cutting thread, and resulted in a different healing pattern.
The initial stability of an implant is essential for achieving osseointegration. This is affected by the mechanical stability achieved due to different surgical procedures, such as altering the drilling technique, and also by the friction between the implant and the bone. With high initial stability it is possible for the implant to interact with growth factors and proteins, which induces osteogenic cell migration to the implant surface. If, in the early stages of healing, the implant is not in an immobile state, the biological pendulum will shift towards soft tissue induction around the implant surface, which may result in fibrous encapsulation. The so-called micromotion of the implant has been studied extensively and it is generally agreed that this phenomenon, when over 150 μm, leads to tissue damage and triggers negative biological bone healing responses.
It has also been stated that a high initial torque is one of the requirements for obtaining primary initial stability. A study conducted on fresh bovine bone showed that higher insertion torque on a specific implant reduced the amount of micromotion, suggesting that there is an inverse relationship between the two factors. Furthermore, it was demonstrated in some clinical studies that an implant with a modified macro-geometry generated higher insertion torque and was more suitable for immediate provisionalization when compared to the conventional straight wall design implants. In contrast, a previous long-term clinical study reported that a low rotational stability at the time of implant insertion is not an indication for delayed loading, and suggested that there may be a misconception with regard to what is actually correlated with primary stability or a low level of micromotion. In that study, implants with a low insertion torque of 25 Ncm were immediately loaded and clinical follow-up was conducted. The results showed a minimum amount of marginal peri-implant bone loss, with high survival rates, suggesting that implant osseointegration may not have a linear relationship with insertion torque values.
In addition, implant insertion torque and the relationship with micromotion may also depend on the cutting feature design. In a previous bench study, the insertion torque and micromotion of implants with the same macro-geometry, with or without cutting features, were recorded. It was shown that implants with a specific cutting feature placed in polyurethane foam blocks significantly decreased not only the insertion torque, but also the micromotion. Changing the implant architecture to include a more efficient a cutting flute decreases the insertion torque, since it reduces the friction forces. Compression and an increased shear force may destroy the supporting bone structure, which consequently lowers the initial stability.
Many of the studies investigating the effect of implant geometry on rotational forces have been conducted on Sawbone or similar material. However, a limitation of these in vitro studies is that it is uncertain whether the material used is actually simulating the biomechanical properties of cortical and cancellous bone. Also, information regarding the biological effects of the cutting flute design on initial stability in vivo and its influence on osseointegration (secondary stability) is limited.
Hence, in order to evaluate purely the effect of the cutting flute, implants with the same macro-geometry with or without a modified cutting flute were utilized in the present study. The objective of this study was to evaluate the early biological effects of implants with a specific cutting flute design placed in the sheep mandibular model. Since this model resembles the human mandible, the outcomes may be related to the actual situation in the oral cavity. The biological responses were evaluated histologically and histomorphometrically.
Materials and methods
This animal study was performed in accordance with the ARRIVE guidelines, and the relevancy of the animal selection and use of animals were considered carefully.
Implant macro-geometry
The present study evaluated two different implant macro-geometries: Blossom ( n = 24 implants) and DT classic self tapping design. ( n = 24 implants) designs, both with the Ossean surface treatment (Intra-Lock International Inc., Boca Raton, FL, USA) ( Fig. 1 ).

Animals and surgery
Six sheep (approximately 2 years of age) were used for the study. Bioethics committee approval was obtained.
The central region of the mandibular body on the lateral aspect was chosen for the procedure. All procedures were performed under general anaesthesia. Pre-anaesthesia consisted of intravenous (i.v.) thiopental (15 mg/kg), followed by orotracheal intubation. Inhalational general anaesthesia was maintained with isofluorane (2.5%), intramuscular (i.m.) ketamine (0.2 mg/kg), and meloxicam (0.5 mg/kg i.m.). After shaving and exposing the skin, an antiseptic solution containing iodine was applied to the surgical site, as well as the surrounding area. A 5-cm incision was made parallel to the inferior border of the mandible. The platysma was dissected and cut in order to reach the periosteum, which was subsequently incised and reflected with a periosteal elevator. Finally, the mandibular body was exposed using manual retractors. The four-implant sites were prepared by drilling at 900 rpm under abundant saline irrigation, in accordance with the manufacturer’s recommendations. The implants were placed in a line at 1 cm intervals, approximately 2 cm above the basal border of the mandibular body. Each animal received two Blossom implants and two DT implants in an interpolated fashion. For instance, a Blossom, a DT, a Blossom, and a DT were placed from distal to proximal in the first animal, and a DT, a Blossom, a DT, and a Blossom were placed from distal to proximal in the second animal. This approach resulted in balanced surgical procedures that allowed the comparison of the same number of implant surfaces by time in vivo , limb, surgical site (1 through 4), and animal. The implants were inserted in the drilled sites, and the maximum insertion torque was recorded with a portable digital torque metre (Tohnichi, Tokyo, Japan), with a 200 Ncm load cell for each implant placed. In the first surgical procedure, 24 implants were placed in the left mandible; a further 24 implants were placed in the contralateral side at second surgery 3 weeks later. Thus the implants remained in place in vivo for 6 weeks on the left side and 3 weeks on the right.
The surgical sites were sutured layer by layer (internal layers: 3–0 vicryl; skin: 3–0 nylon). Postoperatively, all animals were given antibiotics (benzylpenicillin 15 mg/kg and dihydrostreptomycin 20 mg/kg, i.m.) for 5 days, analgesics on the skin (fentanyl patch, 3 μg/h/kg, effect over 3 days), and an anti-inflammatory for 2 days (meloxicam 0.5 mg/kg, i.m.). No signs of infection or other complications were observed during the postoperative period. Euthanasia by anaesthetic overdose was performed after 6 weeks, and the mandibular body was retrieved. After careful removal of the surrounding soft tissue, the surgical site was exposed and implant stability was checked. Thereafter, all samples were subjected to histological processing.
Histological processing and histomorphometric analysis
The bones containing the implants were reduced to blocks and immersed in 10% buffered formalin solution for 24 h. The blocks were then washed in running water for 24 h, and steadily dehydrated in a series of alcohol solutions ranging from 70% to 100% ethanol. Following dehydration, the samples were embedded in a methacrylate-based resin (Technovit 9100; Heraeus Kulzer GmbH, Wehrheim, Germany) following the manufacturer’s instructions. The blocks were then cut into slices (∼300 μm thickness) aiming at the centre of the implant along its long axis with a precision diamond saw (IsoMet 2000; Buehler Ltd., Lake Bluff, IL, USA). The slices were glued to acrylic plates with an acrylate-based cement and left for 24 h to set prior to grinding and polishing. The sections were then reduced to a final thickness of ∼30 μm by means of a series of silicon carbide abrasive papers (SiC 400, 600, 800, 1200, and 2400; Buehler Ltd., Lake Bluff, IL, USA) in a grinding/polishing machine (MetaServ 3000, Buehler Ltd., Lake Bluff, USA) under water irrigation. The sections were then stained with toluidine blue and submitted to optical microscopy at 50–200× magnification (Leica DM2500M; Leica Microsystems GmbH, Wetzlar, Germany) for histomorphological evaluation.
The bone-to-implant contact (BIC) was determined at 50–200× magnification (Leica DM2500M) using computer software (Leica Application Suite; Leica Microsystems GmbH, Wetzlar, Germany). The regions of bone-to-implant contact along the implant perimeter were subtracted from the total implant perimeter, and calculations were performed to determine the BIC. The bone area fraction occupied (BAFO) between threads in trabecular bone regions was determined at 100× magnification (Leica DM2500M) by means of computer software (Leica Application Suite). The areas occupied by bone were subtracted from the total area between threads, and calculations were performed to determine the BAFO (reported in percentage values of bone area fraction occupied).
In order to test the null hypothesis that no differences in insertion torque and biological outcomes (BIC and BAFO) exist between the two different implant designs, statistical analyses were performed using IBM SPSS v. 20 software (IBM Corp., Armonk, NY, USA). Preliminary statistical analyses showed no effect of implant site (i.e., there were no consistent effects of different positions along the mandible) on any measurement. Therefore, site was collapsed in the further analysis. Box plots were used to show the range and distribution of each dependent measure as a function of time and implant design. There were six animals, and each received both implant designs twice at two different times in vivo . Thus, the primary statistical evaluation of the effects of time and implant design on torque, BIC, and BAFO was done with two-way mixed model analysis of variance (ANOVA), with one grouping (time in vivo ) and a repeated factor (implant design), each at two levels while estimating a random intercept. Post hoc comparisons were done with a t -test based on the pooled standard error. Statistical significance was set at 5%, based on the result of either test ( α ≤ 0.05).
Results
A total of 48 dental implants of two different macro-geometries were inserted into the mandibular bodies of six sheep. The surgical procedures and follow-up presented no complications regarding procedural conditions or other immediate clinical concerns. No postoperative complication was detected and no implant was excluded from the study.
The insertion torque recorded for each group is presented in Fig. 2 . The torque was significantly lower for the Blossom implants relative to the DT implants ( P < 0.001).
Histomorphometric evaluation ( Fig. 3 ) showed a remarkably similar pattern for both BIC and BAFO values. A significant increase was detected for both parameters from week 3 to week 6 ( P < 0.01 for both groups), and no significant differences were noted between the implant groups at 3 or 6 weeks in vivo ( P > 0.45).
Qualitative evaluation of the biological response showed close contact between cortical and trabecular bone for both groups at both implantation times, including regions that were in close proximity or substantially distant from the osteotomy walls.
Despite the similar BIC and BAFO values, different healing patterns were observed for the two different implant designs ( Figs. 4–7 ). At 3 weeks, the bone around the DT implant presented two very distinct regions ( Fig. 4 ). The first, the empty space that was present immediately after placement due to the geometric interplay between implant bulk and instrumentation (healing chambers) presented substantial bone formation ( Fig. 4 a). However, regions where primary engagement and bone compression between implant and bone occurred immediately after implantation (the thread tips) presented several areas of bone resorption by multinucleated cells ( Fig. 4 b). At 6 weeks, higher degrees of contact were observed between implant and bone, primarily in the healing chamber regions ( Fig. 5 ). However, despite higher amounts of bone filling the regions between implant threads, several areas of bone resorption by multinucleated cells were also consistently observed in the bone regions adjacent to the thread tips where a high concentration of stress occurs during placement.
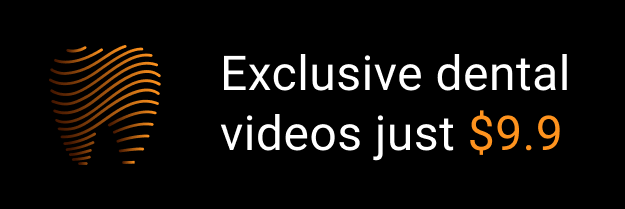