Abstract
Angiogenesis is critical for peri-implant bone regeneration and osseointegration. Endothelial cells (ECs) play an important role in angiogenesis during the early stage of bone formation. Nerve growth factor (NGF) is also reported to function as an angiogenic growth factor. The effects of NGF on ECs seeded on titanium surfaces are unclear. This study was done to investigate the influence of NGF on peri-implant angiogenesis in vitro and in vivo. We used two different titanium surfaces. ECs seeded on these surfaces were treated with indicated concentrations of NGF or vascular endothelial growth factor (VEGF). Proliferation, differentiation, morphological features, and amounts attached were assessed. Chicken embryo chorioallantoic membrane (CAM) was adopted to evaluate the effect of NGF in vivo. The results showed that NGF could promote EC proliferation on both titanium surfaces ( F 1d = 2.083, P = 0.156; F 3d = 30.857, P = 0.0002; F 5d = 4.440, P = 0.041; F 7d = 11.065, P = 0.001). NGF and the SLA surface upregulated mRNA of NGF, TrkA, and p75 expression ( F NGF = 11.941, P = 0.003; F TrkA = 28.514, P = 0.004; F p75 = 7.725, P = 0.01). In vivo, the supernatants of the NGF-treated group could promote neovascularization in CAM ( F = 17.662, P = 0.009). This study demonstrated that NGF could enhance EC proliferation, gene expression on different titanium surfaces, and neovascularization in CAM. This provides novel information in relation to the promotion of early dental implant osseointegration.
Dental implants are a reliable treatment for the replacement of missing teeth. Early stage peri-implant bone formation is an important factor for the success of dental implants. Angiogenesis is a critical process during the formation of new bone, bone regeneration, and osseointegration. Bone mineralization and ossification are tightly coupled with angiogenesis . The formation of blood vessels is one of the earliest events during bone repair.
Nerve growth factor (NGF), first investigated over 60 years ago, is known for its important role in the central and peripheral nervous system. NGF was the first discovered nerve growth-promoting factor. Evidence has shown that it has effects not only on neuronal cells but also on several non-neuronal cells. NGF functions by binding to its two receptors: one is the high-affinity receptor, tyrosine kinase receptor A (TrkA), and the other is the low-affinity receptor, neurotrophin receptor p75 (p75). The discovery of the TrkA and p75 receptors in non-neural tissues, including endothelial cells (ECs), indicates that the influence of NGF is not restricted to the nervous system.
ECs play a pivotal role in the osseointegration of dental implants. ECs have been regarded as an important initiator of the development, remodelling, and repair of bone for a long time. When bone is injured, there is always a hypoxic milieu around the fracture caused by the disruption of blood vessels (oxygen supply). Hypoxia can regulate the production of key modulators by osteoblasts that influence EC proliferation, and at the same time, induce the secretion of osteogenic growth factors by ECs.
The effects of NGF on ECs have been studied widely, but the responses of ECs on implant surfaces with different micro-topographies remain unknown. Hence, we chose two titanium surfaces for this study, the sandblasted with large-grits, acid-etched surface (SLA) and the polished titanium surface (PT). The SLA implant surface is the most widely used in clinics for its advantages in improving osseointegration. PT was the first clinically available dental implant surface (Brånemark), and is still used widely in animal experiments due to its low cost and simplicity of manufacture. The purpose of this study was to further investigate the function of NGF in peri-implant angiogenesis in vitro and in vivo.
Materials and methods
Reagents
Recombinant human beta-NGF and recombinant rat vascular endothelial growth factor (VEGF) were purchased from R&D Systems (Minneapolis, MN, USA). Foetal bovine serum (FBS), Dulbecco’s modified Eagle medium (DMEM), and trypsin were purchased from HyClone (Logan, UT, USA). Cell counting kits (CCK-8) were purchased from Dojindo (Mashiki-machi, Kumamoto, Japan).
Cell culture
A clonal cell line – human umbilical vein endothelial cells (HUVECs), a gift from Dr. Yao – was cultured in DMEM containing 10% FBS and 1% penicillin–streptomycin, and incubated at 37 °C with 5% CO 2 .
Proliferation assay
HUVECs were digested by 0.25% trypsin with ethylenediaminetetraacetic acid (EDTA) and re-suspended in DMEM containing no FBS. Cells were then seeded in 96-well plates at a density of 10 4 /well and incubated at 37 °C with 5% CO 2 . After 12 h, the medium was replaced with fresh DMEM containing 2% FBS with or without NGF at concentrations of 0, 1, 10, and 100 ng/ml. The viability of HUVECs was assessed with the CCK-8 assay on days 1, 3, 5, and 7. The optical density (OD) values were measured at 450 nm using a microplate reader (Varioskan Flash; Thermo Fisher Scientific, USA). After this first step, the group showing the best result was chosen to repeat the CCK-8 assay, comparing this with the VEGF (10 ng/ml) group as the positive control.
Cell cycle assay
After 2 days of culture in different concentrations of NGF, HUVECs were digested with trypsin. The collected cells were then washed with phosphate buffered saline (PBS), centrifuged twice at 2000 × g for 5 min, and fixed with 70% ice-cold alcohol at 4 °C overnight. Cells were then centrifuged, washed with PBS, and incubated in 100 μl RNase at 37 °C for 30 min. Then 300 μl propidium iodide was added and the cells were incubated for 30 min at 4 °C. The S-phase fraction of total cells (SPF) and the DNA proliferation index (PI) of each sample were analyzed and calculated using flow cytometry (Beckman Coulter, USA), according to the following formulae: SPF (%) = S/(G0/G1 + S + G2/M) × 100%; PI (%) = (S + G2/M)/(G0/G1 + S + G2/M) × 100%.
Titanium surfaces
The titanium discs (14 mm in diameter and 1.0 mm in thickness) used in this study were made of commercial pure titanium and supplied by Prof. Liu (National Engineering Research Centre of Biomaterials, Sichuan University, China). These titanium substrates have been examined and used in many other studies by our group. Briefly, for the smooth surfaces, the discs were polished with a sequence of silicon carbide papers. To fabricate the SLA surfaces, the discs were blasted with 300-μm alumina oxide particles and were then ultrasonically cleaned and dried. The blasted samples were next subjected to double chemical etching with hydrogen peroxide and hydrochloric acid. Before the in vitro tests, all titanium discs were ultrasonically cleaned and sterilized in an autoclave. The roughness of the smooth surface was 2.59 Rz; the roughness of the SLA surface was 11.9 Rz.
The HUVECs seeded on these two surfaces were divided into four groups: (1) NGF + SLA group, in which cells were seeded on the SLA surface and treated with NGF; (2) SLA group, in which cells were seeded on the SLA surface, but not treated with NGF; (3) NGF + PT group, in which cells were seeded on the PT surface and treated with NGF; (4) PT group, in which cells were seeded on the PT surface, but not treated with NGF.
Cell proliferation on different titanium surfaces
The titanium discs were placed into 24-well plates (Costra, Coning). HUVECs were seeded at a density of 5 × 10 4 /well and treated with 10 ng/ml of NGF. Cell proliferation on the titanium surfaces was evaluated by MTT assay on days 1, 3, 5, and 7. The test was repeated with VEGF (10 ng/ml) as positive control; cell proliferation was evaluated by CCK-8 on day 3.
Cell attachment on different titanium surfaces
The titanium discs were placed into 24-well plates (Costra, Coning) and seeded with HUVECs at a density of 10 4 /well. After 4 h and 24 h, 4′,6-diamidino-2-phenylindole (DAPI) staining was performed to evaluate the amounts of attached cells under a fluorescent microscope (five visual fields for each sample); a scanning electron microscope (SEM) was used to evaluate the morphological features of HUVECs on the titanium substrates. Briefly, we fixed the cells with 2.5% glutaraldehyde for 2 h and dehydrated the samples with an ascending series of ethanol. The samples, treated by dehydration and coated with gold alloy, were then viewed under the SEM.
Real-time polymerase chain reaction (PCR)
HUVECs on titanium were collected with trypsin/EDTA solution at 37 °C and 5% CO 2 for 5 to 10 min. Total RNA was extracted with an RNA extraction kit (Bioer Technology, China) following the manufacturer’s instructions. The total RNA was quantified by spectrophotometer at A260 nm, and an A260/A280 between 1.8 and 2.0 was considered high purity. The total RNA was then reverse-transcribed to cDNA using a PrimeScript RT-PCR kit (Takara, Japan) following the manufacturer’s protocol. Each real-time PCR was carried out in triplicate and performed using an ABI PRISM 7300 Real-time PCR System (Applied Biosystems, USA). Human glyceraldehyde-3-phosphate dehydrogenase (hGAPDH) was used as internal control. The primers used for the real-time PCR are presented in Table 1 .
Target gene | Primers | Sequence |
---|---|---|
NGF | Forward | 5′ ACAGGAGCAAGCGGTCATCA 3′ |
Reverse | 5′ ATGTCTGTGGCGGTGGTCTTA 3′ | |
TrkA | Forward | 5′ TGGAGAAGAAGGACGAAACACC 3′ |
Reverse | 5′ GCGGTTGATCCCAAACTTGTT 3′ | |
p75 | Forward | 5′ CAAGACCTCATAGCCAGCACG 3′ |
Reverse | 5′ GCCAGGATGGAGCAATAGACA 3′ | |
VEGF | Forward | 5′ GAGGGCAGAATCATCACGAAGT 3′ |
Reverse | 5′ GGCACACAGGATGGCTTGAA 3′ |
Chicken embryo chorioallantoic membrane (CAM) assay
Fertilized eggs were incubated at 37 °C with 60% humidity. On the 8th day, a square window was opened. CAMs were treated with 0.5 ml cell supernatant of each group. The embryos were then incubated at 37 °C with 60% humidity for another 24 h. The CAMs were then fixed in 4% paraformaldehyde for 1 h. Following this the CAMs were removed with curved-tip forceps and each specimen transferred to a Petri dish. Images were acquired with a computer scanner and analyzed with Image Pro Plus (Media Cybernetics, Silver Spring, MD, USA).
Statistical analysis
Data are presented as the mean ± standard deviation (SD). Statistical comparisons between groups were performed using one-way analysis of variance and the least significant difference (LSD) for multiple comparisons.
Results
Different concentrations of NGF induce the proliferation of ECs
To determine the best concentration, we initially examined the effects of different concentrations of NGF on EC proliferation using the CCK-8 and cell cycle assays. As shown in Table 2 , on day 3, 10 ng/ml NGF could significantly induce proliferation of ECs ( F = 5.849, P = 0.108), and this was very stable until day 7 ( F = 4.136, P = 0.025). The cell cycle assay showed that the 10 ng/ml group SPF and PI indexes were higher than those of the other groups ( F SPF = 14.145, P = 0.001; F PI = 161.332, P < 0.001; Table 3 ). Thus we chose the concentration 10 ng/ml for the subsequent experiments.
Day | Concentration of NGF | Mean | SD | 95% CI | P -value a | |
---|---|---|---|---|---|---|
Lower bound | Upper bound | |||||
1 | 0 ng/ml | 0.504 | 0.053 | 0.438 | 0.570 | 0.775 |
1 ng/ml | 0.530 | 0.082 | 0.428 | 0.631 | ||
10 ng/ml | 0.500 | 0.043 | 0.446 | 0.554 | ||
100 ng/ml | 0.495 | 0.039 | 0.447 | 0.543 | ||
3 | 0 ng/ml | 1.411 | 0.146 | 1.178 | 1.643 | 0.108 |
1 ng/ml | 1.556 | 0.122 | 1.362 | 1.749 | ||
10 ng/ml | 1.689 | 0.186 | 1.394 | 1.986 | ||
100 ng/ml | 1.502 | 0.126 | 1.301 | 1.702 | ||
5 | 0 ng/ml | 2.832 | 0.824 | 1.809 | 3.856 | 0.602 |
1 ng/ml | 3.335 | 0.178 | 3.052 | 3.618 | ||
10 ng/ml | 3.119 | 0.055 | 2.623 | 3.615 | ||
100 ng/ml | 3.230 | 0.088 | 2.435 | 4.024 | ||
7 | 0 ng/ml | 3.104 | 0.149 | 2.919 | 3.288 | 0.025 |
1 ng/ml | 3.174 | 0.542 | 2.501 | 3.846 | ||
10 ng/ml | 3.595 | 0.118 | 3.449 | 3.742 | ||
100 ng/ml | 3.644 | 0.072 | 3.531 | 3.759 |
Group | Concentration of NGF | Mean | SD | 95% CI | P -value a | |
---|---|---|---|---|---|---|
Lower bound | Upper bound | |||||
SPF | 0 ng/ml | 0.344 | 0.005 | 0.333 | 0.356 | 0.001 |
1 ng/ml | 0.370 | 0.016 | 0.332 | 0.409 | ||
10 ng/ml | 0.375 | 0.004 | 0.364 | 0.386 | ||
100 ng/ml | 0.338 | 0.002 | 0.333 | 0.343 | ||
PI | 0 ng/ml | 0.397 | 0.004 | 0.387 | 0.407 | <0.001 |
1 ng/ml | 0.435 | 0.005 | 0.423 | 0.446 | ||
10 ng/ml | 0.427 | 0.003 | 0.418 | 0.435 | ||
100 ng/ml | 0.359 | 0.006 | 0.344 | 0.375 |
Cell proliferation on titanium
As shown in Table 4 , NGF could promote EC proliferation on both the SLA and PT titanium surfaces from day 3 ( F 1d = 2.083, P = 0.156; F 3d = 30.857, P = 0.0002; F 5d = 4.440, P = 0.041; F 7d = 11.065, P = 0.001). Moreover, the different titanium surfaces had a further effect. The MTT results for the PT + NGF group were significantly higher than for the SLA + NGF group at day 7 ( t = 2.855, P = 0.046). Figure 1 shows the morphological features of HUVECs on the titanium substrates. At 2 h, HUVECs were ball-shaped on both SLA and PT titanium; at 24 h, most HUVECs on PT titanium were stretched with a few exceptions, but on SLA titanium, all of the cells were stretched and fused into the rough titanium background.
Day | Group a | Mean | SD | 95% CI | P -value b | |
---|---|---|---|---|---|---|
Lower bound | Upper bound | |||||
1 | NGF + SLA | 0.494 | 0.025 | 0.455 | 0.533 | 0.156 |
SLA | 0.439 | 0.009 | 0.424 | 0.453 | ||
NGF + PT | 0.558 | 0.060 | 0.463 | 0.652 | ||
PT | 0.509 | 0.119 | 0.320 | 0.699 | ||
3 | NGF + SLA | 1.236 | 0.034 | 0.931 | 1.541 | 0.0002 |
SLA | 1.134 | 0.013 | 1.013 | 1.254 | ||
NGF + PT | 1.164 | 0.096 | 0.926 | 1.402 | ||
PT | 0.851 | 0.022 | 0.816 | 0.886 | ||
5 | NGF + SLA | 2.678 | 0.186 | 2.217 | 3.139 | 0.041 |
SLA | 2.399 | 0.151 | 2.158 | 2.639 | ||
NGF + PT | 2.732 | 0.025 | 2.670 | 2.795 | ||
PT | 2.552 | 0.021 | 2.361 | 2.743 | ||
7 | NGF + SLA | 2.759 | 0.103 | 2.595 | 2.923 | 0.001 |
SLA | 2.452 | 0.083 | 2.320 | 2.583 | ||
NGF + PT | 3.089 | 0.264 | 2.668 | 3.510 | ||
PT | 2.568 | 0.158 | 2.317 | 2.819 |
a NGF + SLA: cells were seeded on an SLA surface and treated with NGF; SLA: cells were seeded on an SLA surface, but not treated with NGF; NGF + PT: cells were seeded on a PT surface and treated with NGF; PT: cells were seeded on a PT surface, but not treated with NGF.

The amounts of HUVECs on the different titanium surfaces are shown in Table 5 . At 24 h, the amounts of cells in the NGF groups (NGF + SLA and NGF + PT) were significantly higher than in the control groups (SLA and PT) ( F = 28.343, P < 0.001). Further, the amount of cells in the NGF + PT group was significantly higher than in the NGF + SLA group ( t = 8.536, P < 0.001).
Time | Group a | Mean | SD | 95% CI | P -value b | |
---|---|---|---|---|---|---|
Lower bound | Upper bound | |||||
4 h | NGF + SLA | 127.250 | 48.717 | 86.522 | 167.979 | 0.809 |
SLA | 117.250 | 42.253 | 81.925 | 152.575 | ||
NGF + PT | 140.000 | 19.630 | 108.764 | 171.236 | ||
PT | 117.333 | 43.122 | 72.080 | 162.587 | ||
24 h | NGF + SLA | 370.500 | 24.266 | 350.213 | 390.787 | <0.001 |
SLA | 278.000 | 60.584 | 214.421 | 341.579 | ||
NGF + PT | 485.000 | 15.011 | 461.114 | 508.886 | ||
PT | 304.000 | 20.785 | 270.927 | 337.073 |
a NGF + SLA: cells were seeded on an SLA surface and treated with NGF; SLA: cells were seeded on an SLA surface, but not treated with NGF; NGF + PT: cells were seeded on a PT surface and treated with NGF; PT: cells were seeded on a PT surface, but not treated with NGF.
EC differentiation
As determined by real-time quantitative PCR, exogenous NGF and the SLA titanium surface could upregulate the expression levels of NGF and its receptors TrkA and p75 ( F NGF = 11.941, P = 0.003; F TrkA = 28.514, P = 0.004; F p75 = 7.725, P = 0.01). The levels of these three mRNAs in the NGF + SLA group increased to almost two-fold those of the SLA group ( Table 6 ).
mRNA | Group a | Mean | SD | 95% CI | P -value b | |
---|---|---|---|---|---|---|
Lower bound | Upper bound | |||||
NGF | NGF + SLA | 2.147 | 0.411 | 1.125 | 3.169 | 0.003 |
SLA | 1.206 | 0.236 | 0.619 | 1.794 | ||
NGF + PT | 1.236 | 0.192 | 0.758 | 1.714 | ||
PT | 1.000 | 0.000 | 1.000 | 1.000 | ||
p75 | NGF + SLA | 1.688 | 0.119 | 1.394 | 1.983 | 0.01 |
SLA | 1.001 | 0.365 | 0.096 | 1.907 | ||
NGF + PT | 1.194 | 0.130 | 0.872 | 1.517 | ||
PT | 1.000 | 0.000 | 1.000 | 1.000 | ||
TrkA | NGF + SLA | 1.558 | 0.000 | 1.558 | 1.558 | 0.004 |
SLA | 0.740 | 0.126 | 0.493 | 0.987 | ||
NGF + PT | 1.296 | 0.139 | 0.043 | 2.549 | ||
PT | 1.000 | 0.000 | 1.000 | 1.000 |
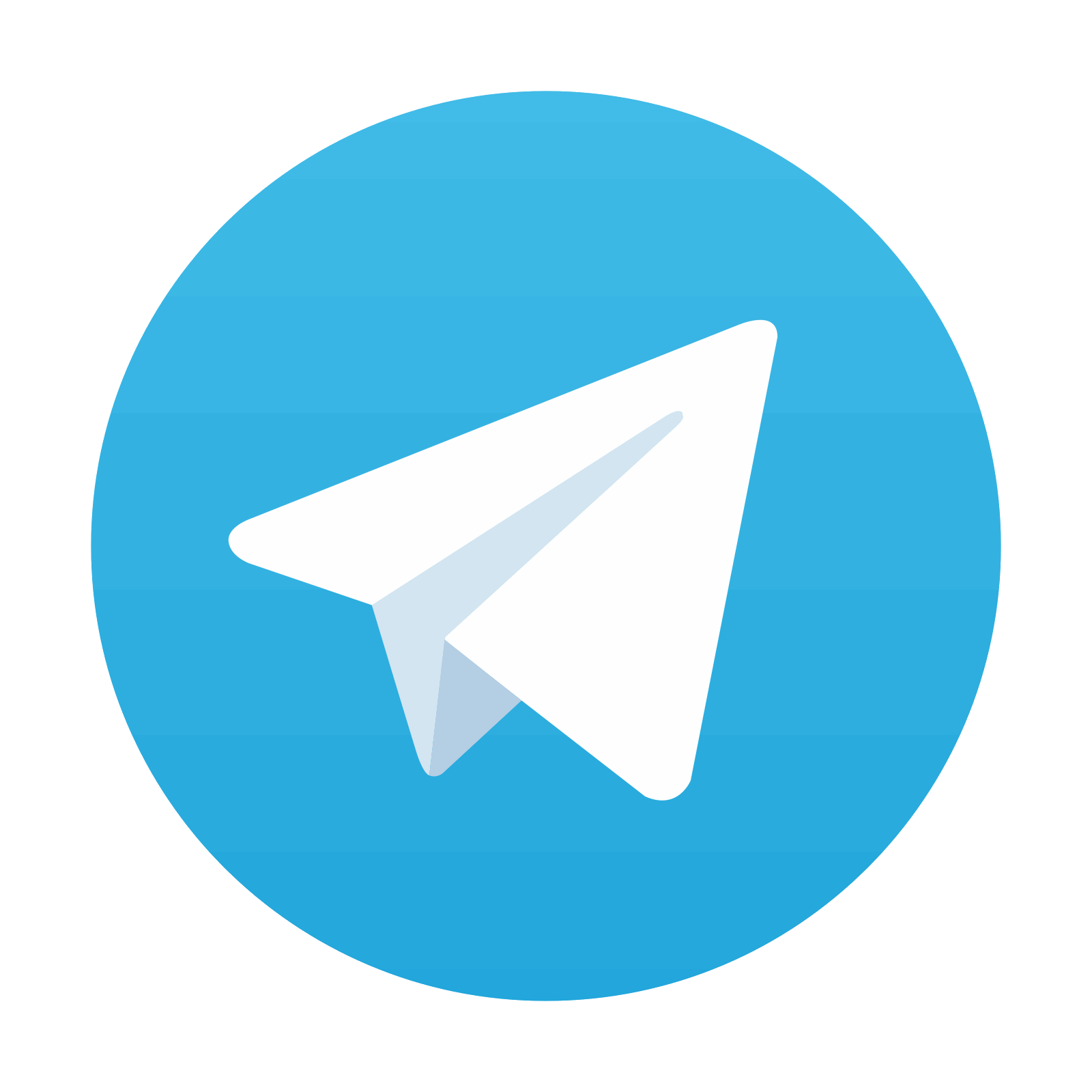
Stay updated, free dental videos. Join our Telegram channel

VIDEdental - Online dental courses
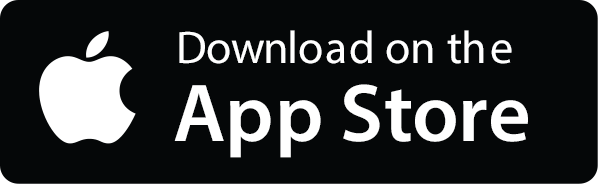
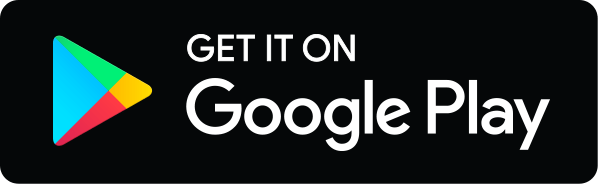
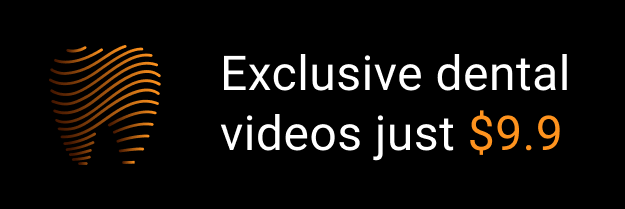