Highlights
- •
Primary study applying SPS for a range of lithium disilicate dental glass-ceramics.
- •
Crystal size reduction of lithium disilicate CAD–CAM glass-ceramics via controlled SPS parameters.
- •
Unique textured/nano-crystalline microstructures produced by densification of glasses.
- •
SPS allowed rapid, low temperature crystallization of dental glasses.
Abstract
Objectives
To evaluate the effects of spark plasma sintering (SPS) on the microstructure of lithium disilicate glass-ceramics.
Methods
IPS e.max CAD glass-ceramic samples were processed using spark plasma sintering (SPS) and conventionally sintered (CS) as a comparison. Specimens were sintered at varying temperatures (T1: 840 °C, T2: 820 °C, T3: 800 °C), heating rates (HR1: 150 °C/min, HR2: 300 °C/min, HR3: 500 °C/min) and pressures (P1: 15 MPa, P2: 50 MPa, P3: 70 MPa). IPS e.max Press glass powder samples were densified at 750 and 800 °C (50 or 200 MPa pressure). Samples were characterized using XRD, HTXRD, and SEM and quantitative image analysis.
Results
There was a significant increase in median crystal size (MCS) between the CS and the SPS T1 groups. A statistical difference ( p > 0.05) in MCS between SPS T1 and SPS T2 groups was observed. The SPS HR3 sample produced a smaller MCS than the CS, SPS HR1 and HR2 groups ( p < 0.05). The SPS P3 sample had a reduction in MCS compared with the CS group ( p < 0.05). XRD of the SPS samples revealed major lithium disilicate/lithium metasilicate phases and minor lithium orthophosphate and cristobalite/quartz phases. Densified IPS e.max Press glass samples resulted in fine fibrils or graduated lithium disilicate crystals.
Significance
The effects of SPS were used to refine the microstructure of IPS e.max CAD lithium disilicate glass-ceramics. Densification by SPS of IPS e.max Press glass resulted in textured and fine nano-crystalline microstructures. SPS generated glass-ceramic microstructures may have unique properties and could be useful in the production of CAD/CAM materials for dentistry.
1
Introduction
Lithium disilicate glass-ceramics are now well established for the construction of dental appliances using heat extrusion and computer-aided design/computer-aided machining (CAD–CAM). Depending on the manufacturing process these materials are produced as ingots (IPS e.max Press, IPS Empress 2, Ivoclar-Vivadent, Schaan, Liechtenstein) or blocks (IPS e.max CAD, Ivoclar-Vivadent). A colored pressure cast block used for CAD/CAM applications is produced in a partially crystallized state with a 40% lithium metasilicate phase (Li 2 SiO 3 ), to allow ease of machining and reduced tooling costs. A second heat treatment is given following the milling procedure at 840–850 °C to allow the formation of 70% lithium disilicate crystals (Li 2 Si 2 O 5 ) . The ingots for heat extrusion are in contrast produced by the crystallization of the base glass to form the final lithium disilicate glass-ceramic which can be heat extruded at 920 °C. This also imparts the material a high flexural strength and fracture toughness of 360 MPa and 2.5 MPa m ½ for IPS e.max CAD , and 440 MPa and 2.75 MPa m ½ for IPS e.max Press .
These glass-ceramics are however synthesized by crystallizing a base glass using nucleation and crystal growth heat treatment schedules in a conventional furnace. This process is limited by the typical maximum rate at which a furnace can be ramped (typically 5–30 °C/min) and is carried out at atmospheric pressure. Heat extrusion of these glass-ceramics is also conducted at very low pressures (3–4 × 10 5 Pa) compared with more modern processing techniques. These manufacturing restrictions potentially limit the extent and scope of the microstructural and property optimization of these materials.
Spark plasma sintering (SPS) is a consolidation technique used to rapidly produce fully dense ceramics/glasses with fine grained microstructures at low temperatures. The SPS process involves the compaction of a powder into a graphite mold followed by applying a pulsed DC current, under uniaxial pressure and at high heating rates (up to 1000 °C/min) . These rapid heating rates make it possible to avoid undesirable grain growth at the lower sintering rates used in conventional techniques like hot pressing . This has resulted in dramatic reductions in grain size (0.5–0.6 μm) when compared with conventional sintering (30 μm) of alumina ceramics . The applied pressure in this process also acts as a driving force for sintering resulting in higher densities at lower temperatures . It is also thought that during SPS the production of a plasma acts to clean the powders surface of impurities (absorbed CO 2 and H 2 O), enhancing grain boundary diffusion processes and densification . SPS of ceramic materials results in improved transparency , reduced porosity and increased flexural strength . SPS has demonstrated some success in producing dense optical materials including oxygen sensitive chalcogenide glasses and glass-ceramics for infrared applications . This processing technique may therefore be useful in the preparation of dental materials to tailor/optimize the optical and mechanical properties. There may also be the opportunity for greater control of the crystallization, giving greater latitude for more precise phase/crystal size control . Faster and more efficient densification of fine grained CAD–CAM ceramic materials compared with existing methods could therefore be achieved. Fully optimized SPS ceramic materials produced as blocks could be integrated with CAM to allow more cost effective machining/processing and retaining unique material properties. There is little known in the literature on the effect of SPS on lithium silicate glass-ceramics and glasses used in dentistry, including the effects of changes in temperature, heating rate and pressure. The aims of the study were to evaluate the effects of spark plasma sintering (SPS) on the microstructure of lithium disilicate glass-ceramics.
2
Materials and methods
The materials used in the current study comprised lithium disilicate glass-ceramics/glasses used in dentistry to produce either CAD–CAM restorations (IPS e.max CAD, Inlab LT A3/C14, LOT: P37665, Ivoclar-Vivadent, Schaan, Liechtenstein) or heat extruded restorations (IPS e.max Press glass LT A2, LOT: 603221, Ivoclar-Vivadent). These materials were selected as they provided the opportunity to evaluate the effects of SPS on glass-ceramics in this system in the partially crystallized state (IPS e.max CAD) and on that of the starting glass (IPS e.max Press) before the crystallization heat treatments.
The IPS e.max CAD glass-ceramic was used for the spark plasma sintering experiment in powder form at varying pressures, temperatures, and hold durations ( Table 1 ). To produce the powder the glass-ceramic block was cut using a diamond disc (Skillbond, UK) and ground in to a powder using an alumina mortar and pestle (Milton Brook 3, UK) and screened to 45 μm. The IPS e.max Press glass was received as a powder ( D 50 = 8.25 μm) from the manufacturer.
IPS e.max CAD | Pressure (MPa) | Heat rate (°C/min) | Temperature (°C) | Hold (min) |
---|---|---|---|---|
SPS (T1) | 50 | 90 | 840 | 7.0 |
SPS (T2) | 50 | 90 | 820 | 7.0 |
SPS (T3) | 50 | 90 | 800 | 7.0 |
SPS (HR1) | 50 | 150 | 840 | 7.0 |
SPS (HR2) | 50 | 300 | 840 | 7.0 |
SPS (HR3) | 50 | 500 | 840 | 7.0 |
SPS (P1) | 15 | 90 | 840 | 7.0 |
SPS (P2) | 50 | 90 | 840 | 3.0 |
SPS (P3) | 70 | 90 | 840 | 7.0 |
2.1
Differential scanning calorimetry
The IPS e.max CAD and IPS e.max Press powders were characterized using differential scanning calorimetry (DSC) using a Stanton Redcroft DSC 1500 (Rheometric Scientific, Epsom, UK). A bulk sample (IPS e.max CAD) was also run to help identify the type of crystallization (e.g. surface or bulk). Alumina was used as the reference material. Powders and the bulk sample were heated from room temperature to 1100 °C at a heating rate of 20 °C/min.
2.2
Preparation of the sintered IPS e.max specimen
An IPS e.max CAD block (Ivoclar-Vivadent) was cut to produce a section using a diamond disc (Skillbond, UK) at a speed of 20 rpm. The sample was placed on an IPS e.max CAD crystallization tray using IPS object fix flow auxiliary paste (LOT: P36330, Ivoclar-Vivadent). A dehumidification program was run using the Programat EP3000 furnace (Ivoclar-Vivadent), prior to sintering the samples on the crystallization program in the EP3000. Samples ( Table 1 ) were ramped at a rate of 90 °C/min from 403 to 820 °C (0.1 min hold) followed by ramping (30 °C/min) to 840 °C with a 7 min hold. A vacuum was maintained from 550 to 840 °C during the heat treatment cycle. A Type K external thermocouple attached to a data logger (Hoboware Light Software, UK) was placed in the EP3000 furnace to monitor temperature and hold times during the program.
2.3
Spark plasma sintering (SPS) of IPS e.max CAD
1.6 g of the IPS e.max CAD glass-ceramic powder was weighed using a microbalance (Adam Equipment Co, Milton Keynes, UK). A lower graphite punch ( Ф = 2 cm) was pushed into the graphite die opening before placing the weighed glass-ceramic powder between the upper and lower graphite punches. The die/punch assembly was wrapped in a graphite insulating felt and placed in the spark plasma sintering (SPS) system (HPD 25/1, FCT Systeme, Germany). The powder was then heated according to the heat treatment schedules in Table 1 under vacuum (10 Pa). The SPS schedule was varied to asses the effects of temperature, heat rate and pressure on the glass-ceramic specimens. The temperature was measured using a top pyrometer focused inside a hole in the punch at distance of 4 mm from the sample. A pyrometer rather than a thermocouple was used to allow more accurate temperature measurement, due to the problems of following temperature cycles with heating rates as high as 500 °C/min. The direct current (DC) pulse was 15 ms on and 5 ms off throughout the whole process. Following the heating treatment cycles in Table 1 all specimens were cooled to 450 °C in 2 min and then left to cool to room temperature. The compacted samples were retrieved from the graphite mold and the carbon-coated layer was removed using P320 silicon carbide paper (Struers, UK).
2.4
Spark plasma sintering of IPS e.max Press
Densification of 0.5 g of IPS e.max Press glass was carried out according to the previously described SPS method. The sample diameter was however prepared at 1 cm. A K-type thermocouple was attached through the graphite mold for accurate temperature measurement in this part of the study. Glass samples were densified using two schedules. Sample 1 (D1-750) was heated at a rate of 50 °C/min from 23 to 750 °C under a pressure of 200 MPa (no hold). Sample 2 (D2-800) was heated at a rate of 50 °C/min from 23 to 800 °C under a pressure of 50 MPa (2 min hold). Both specimens were cooled to 450 °C (2 min) and then cooled to room temperature in 4 min.
Lower temperatures, ramps and holds were used in the current experiment to avoid excessive flow and loss of the glass.
2.5
X-ray diffraction analysis
All the samples ( Table 1 ) were analyzed using a Panalytical X’Pert Pro powder diffractometer (Panalytical B.V., Almelo, Netherlands). Data was collected continuously with an X’Celerator RTMS detector and a counting time equivalent to 100 s per point over 5–70° 2 θ with a stepsize of 0.0334 or 0.0167° 2 θ .
CuKα radiation was used with the tube powered at 45 kV and 40 mA. X’pert Highscore Plus (v2.2a) was used to identify the crystal phases using the ICDD database. Diffraction patterns for lithium disilicate (De Jong et al.) , lithium metasilicate (00-033-0766), β-lithium orthophosphate (01-087-0039), crystobalite (high = 04-008-8250, low = 00-039-1425) and quartz (α = 01-089-8936, β = 00-047-1144) were used.
High temperature X-ray diffraction (HTXRD) analysis was carried out on IPS e.max CAD powder specimens in a temperature calibrated HTK16 platinum strip furnace (Anton Paar GmbH., Graz, Austria) under air. The heating rate was 90 °C/min. Data was collected at 800, 820, 840 and 860 °C with a 7 min delay before data collection at each temperature for equilibration.
2.6
Secondary electron microscopy (SEM)
All samples were embedded and ground to P 4000 silicon carbide paper (Struers, UK) followed by 1 μm and 0.3 μm alumina micro polish (Buehler Ltd., UK). The SPS IPS e.max CAD samples were etched for 10 s using 4% hydrofluoric acid mixed with 30% sulfuric acid. Densified IPS e.max Press glass samples (D1-750, D2-800) were etched using 2% hydrofluoric acid mixed with 15% sulfuric acid. Etched samples were gold coated using an Agar auto sputter coater (Agar Scientific, UK) for 30 s at 40 mA. A Scanning Electron Microscope (FEI Inspect, Eindhoven, Netherlands) was used to view the specimens in the secondary electron imaging mode. Quantitative image analysis was used to measure the median crystal size, area fraction, and number of crystals using a Sigma Scan Pro 5.0 image analysis software program (Systat Software Inc., Chicago, USA). Crystal size results were analyzed for significant differences ( p < 0.05, Dunn’s multiple comparisons test, Sigma stat, version 2.03, SPSS Inc.).
2
Materials and methods
The materials used in the current study comprised lithium disilicate glass-ceramics/glasses used in dentistry to produce either CAD–CAM restorations (IPS e.max CAD, Inlab LT A3/C14, LOT: P37665, Ivoclar-Vivadent, Schaan, Liechtenstein) or heat extruded restorations (IPS e.max Press glass LT A2, LOT: 603221, Ivoclar-Vivadent). These materials were selected as they provided the opportunity to evaluate the effects of SPS on glass-ceramics in this system in the partially crystallized state (IPS e.max CAD) and on that of the starting glass (IPS e.max Press) before the crystallization heat treatments.
The IPS e.max CAD glass-ceramic was used for the spark plasma sintering experiment in powder form at varying pressures, temperatures, and hold durations ( Table 1 ). To produce the powder the glass-ceramic block was cut using a diamond disc (Skillbond, UK) and ground in to a powder using an alumina mortar and pestle (Milton Brook 3, UK) and screened to 45 μm. The IPS e.max Press glass was received as a powder ( D 50 = 8.25 μm) from the manufacturer.
IPS e.max CAD | Pressure (MPa) | Heat rate (°C/min) | Temperature (°C) | Hold (min) |
---|---|---|---|---|
SPS (T1) | 50 | 90 | 840 | 7.0 |
SPS (T2) | 50 | 90 | 820 | 7.0 |
SPS (T3) | 50 | 90 | 800 | 7.0 |
SPS (HR1) | 50 | 150 | 840 | 7.0 |
SPS (HR2) | 50 | 300 | 840 | 7.0 |
SPS (HR3) | 50 | 500 | 840 | 7.0 |
SPS (P1) | 15 | 90 | 840 | 7.0 |
SPS (P2) | 50 | 90 | 840 | 3.0 |
SPS (P3) | 70 | 90 | 840 | 7.0 |
2.1
Differential scanning calorimetry
The IPS e.max CAD and IPS e.max Press powders were characterized using differential scanning calorimetry (DSC) using a Stanton Redcroft DSC 1500 (Rheometric Scientific, Epsom, UK). A bulk sample (IPS e.max CAD) was also run to help identify the type of crystallization (e.g. surface or bulk). Alumina was used as the reference material. Powders and the bulk sample were heated from room temperature to 1100 °C at a heating rate of 20 °C/min.
2.2
Preparation of the sintered IPS e.max specimen
An IPS e.max CAD block (Ivoclar-Vivadent) was cut to produce a section using a diamond disc (Skillbond, UK) at a speed of 20 rpm. The sample was placed on an IPS e.max CAD crystallization tray using IPS object fix flow auxiliary paste (LOT: P36330, Ivoclar-Vivadent). A dehumidification program was run using the Programat EP3000 furnace (Ivoclar-Vivadent), prior to sintering the samples on the crystallization program in the EP3000. Samples ( Table 1 ) were ramped at a rate of 90 °C/min from 403 to 820 °C (0.1 min hold) followed by ramping (30 °C/min) to 840 °C with a 7 min hold. A vacuum was maintained from 550 to 840 °C during the heat treatment cycle. A Type K external thermocouple attached to a data logger (Hoboware Light Software, UK) was placed in the EP3000 furnace to monitor temperature and hold times during the program.
2.3
Spark plasma sintering (SPS) of IPS e.max CAD
1.6 g of the IPS e.max CAD glass-ceramic powder was weighed using a microbalance (Adam Equipment Co, Milton Keynes, UK). A lower graphite punch ( Ф = 2 cm) was pushed into the graphite die opening before placing the weighed glass-ceramic powder between the upper and lower graphite punches. The die/punch assembly was wrapped in a graphite insulating felt and placed in the spark plasma sintering (SPS) system (HPD 25/1, FCT Systeme, Germany). The powder was then heated according to the heat treatment schedules in Table 1 under vacuum (10 Pa). The SPS schedule was varied to asses the effects of temperature, heat rate and pressure on the glass-ceramic specimens. The temperature was measured using a top pyrometer focused inside a hole in the punch at distance of 4 mm from the sample. A pyrometer rather than a thermocouple was used to allow more accurate temperature measurement, due to the problems of following temperature cycles with heating rates as high as 500 °C/min. The direct current (DC) pulse was 15 ms on and 5 ms off throughout the whole process. Following the heating treatment cycles in Table 1 all specimens were cooled to 450 °C in 2 min and then left to cool to room temperature. The compacted samples were retrieved from the graphite mold and the carbon-coated layer was removed using P320 silicon carbide paper (Struers, UK).
2.4
Spark plasma sintering of IPS e.max Press
Densification of 0.5 g of IPS e.max Press glass was carried out according to the previously described SPS method. The sample diameter was however prepared at 1 cm. A K-type thermocouple was attached through the graphite mold for accurate temperature measurement in this part of the study. Glass samples were densified using two schedules. Sample 1 (D1-750) was heated at a rate of 50 °C/min from 23 to 750 °C under a pressure of 200 MPa (no hold). Sample 2 (D2-800) was heated at a rate of 50 °C/min from 23 to 800 °C under a pressure of 50 MPa (2 min hold). Both specimens were cooled to 450 °C (2 min) and then cooled to room temperature in 4 min.
Lower temperatures, ramps and holds were used in the current experiment to avoid excessive flow and loss of the glass.
2.5
X-ray diffraction analysis
All the samples ( Table 1 ) were analyzed using a Panalytical X’Pert Pro powder diffractometer (Panalytical B.V., Almelo, Netherlands). Data was collected continuously with an X’Celerator RTMS detector and a counting time equivalent to 100 s per point over 5–70° 2 θ with a stepsize of 0.0334 or 0.0167° 2 θ .
CuKα radiation was used with the tube powered at 45 kV and 40 mA. X’pert Highscore Plus (v2.2a) was used to identify the crystal phases using the ICDD database. Diffraction patterns for lithium disilicate (De Jong et al.) , lithium metasilicate (00-033-0766), β-lithium orthophosphate (01-087-0039), crystobalite (high = 04-008-8250, low = 00-039-1425) and quartz (α = 01-089-8936, β = 00-047-1144) were used.
High temperature X-ray diffraction (HTXRD) analysis was carried out on IPS e.max CAD powder specimens in a temperature calibrated HTK16 platinum strip furnace (Anton Paar GmbH., Graz, Austria) under air. The heating rate was 90 °C/min. Data was collected at 800, 820, 840 and 860 °C with a 7 min delay before data collection at each temperature for equilibration.
2.6
Secondary electron microscopy (SEM)
All samples were embedded and ground to P 4000 silicon carbide paper (Struers, UK) followed by 1 μm and 0.3 μm alumina micro polish (Buehler Ltd., UK). The SPS IPS e.max CAD samples were etched for 10 s using 4% hydrofluoric acid mixed with 30% sulfuric acid. Densified IPS e.max Press glass samples (D1-750, D2-800) were etched using 2% hydrofluoric acid mixed with 15% sulfuric acid. Etched samples were gold coated using an Agar auto sputter coater (Agar Scientific, UK) for 30 s at 40 mA. A Scanning Electron Microscope (FEI Inspect, Eindhoven, Netherlands) was used to view the specimens in the secondary electron imaging mode. Quantitative image analysis was used to measure the median crystal size, area fraction, and number of crystals using a Sigma Scan Pro 5.0 image analysis software program (Systat Software Inc., Chicago, USA). Crystal size results were analyzed for significant differences ( p < 0.05, Dunn’s multiple comparisons test, Sigma stat, version 2.03, SPSS Inc.).
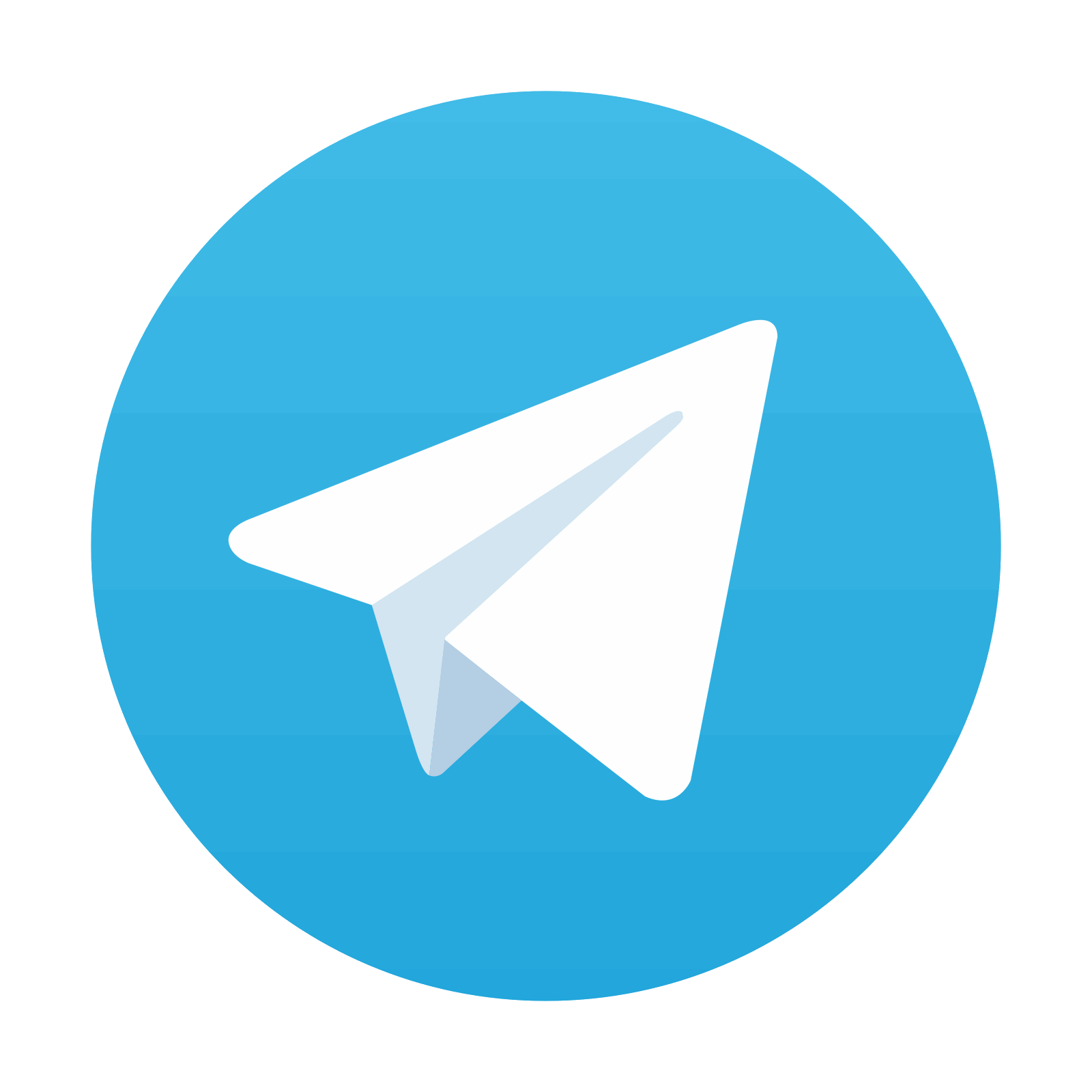
Stay updated, free dental videos. Join our Telegram channel

VIDEdental - Online dental courses
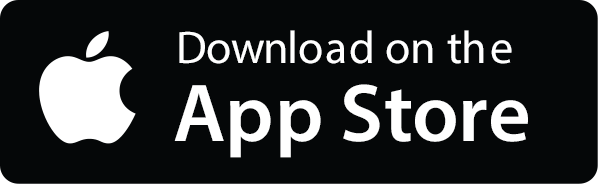
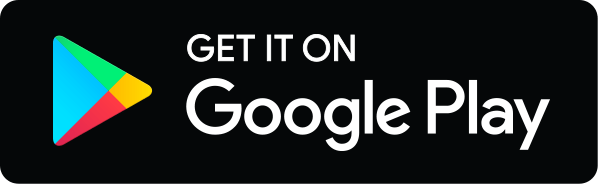