Abstract
Objectives
This study evaluated the effect of etching using 2% proanthocynidin-containing 10% phosphoric acid 2% PA/10% PhA vs. 35% phosphoric acid 35% PhA on immediate (IM) and 6-months (6 M) resin–enamel microshear bond strength (μSBS), resin–dentin microtensile bond strength (μTBS), nanoleakage (NL) and as well as in situ MMP inhibition potential.
Methods
The dentin surface of human were exposed and then etched using 35% phosphoric acid for 15 s or 2% PA/10% phosphoric acid for 30 s. After rinsing with water, the dentin was bonded with Single Bond Plus (3 M ESPE) and composite build-ups were constructed, followed by polymerization. The teeth were sectioned and the bonds were testing for microtensile bond strength (μTBS) and by SEM for NL analysis at IM and 6 M. For MMP activity, resin–dentin slices were prepared for in situ zymography, and analyzed under confocal microscopy. For μSBS, others teeth had flattened enamel surfaces etched according the experimental groups and prepared to microshear procedure. The specimens were tested IM and after 6 M by microshear bond strength. The data were submitted to two-way repeated measures ANOVA and Tukey’s test ( α = 0.05).
Results
Acid-etching using the 2% PA/10% phosphoric acid did not lower the μTBS in IM ( p > 0.05) compared to the control 35% phosphoric acid group. However, after 6 M, only the 2% PA/10% PhA etched dentin had remained stable the resin–dentin bond strength ( p < 0.05). Bonds made with 35% PhA showed significant increase in NL% after 6 M ( p < 0.05). Dentin bonds made with 2% PA/10% phosphoric acid showed no increase in NL% after 6 months. The MMP activity within the resin–dentin interface was almost completely reduced after 2% PA/10% PhA etching, while the 35% PhA exhibited intense MMP activity. For μSBS, the type of etchant and the storage period did not affect the resin–enamel bond strengths ( p > 0.05).
Significance
Ten percent phosphoric acid containing 2% PA can produce stable resin–dentin and enamel–resin interfaces, without requiring additional steps in the bonding procedure. Future studies for longer evaluation time are required.
1
Introduction
There is a general consensus that the resin–dentin bonds created with contemporary hydrophilic dentin bonding systems deteriorate over time . For etch-and-rinse adhesives, there is a decreasing gradient of resin monomer diffusion within the hybrid layers . This results in incomplete resin infiltration at the bottom the hybrid layer, leaving denuded collagen fibrils that are susceptible to enzymatic degradation by host-derived collagen-bound matrix metalloproteinases (MMPs) and cysteine cathepsins . Additionally, simplified etch-and-rinse systems are capable of activating these matrix metalloproteinases (MMPs) . Consequently, procedures that enhance dentin collagen’s resistance towards collagenolytic activity of host-derived enzymes have great potential to improving the longevity of dentin bonding.
To increase the collagen stability, one might employ MMP inhibitors and collagen cross-linking agents. Exogenous MMP inhibitors, such as chlorhexidine, are capable to reduce the protease activity and to prolong the durability of resin–dentin bonds but they lack chemical bond with the collagen fibrils. Collagen cross-linkers establish chemical bonds with the collagen and may also increase the collagen resistance against the effect of host-derived proteases .
The delivery of these agents to demineralized dentin can be via application of primers where a therapeutic agent can be incorporated into one of the components of the bonding protocol . The application of the agents as a primer is hampered by the fact that this procedure adds another step to the bonding protocol, which is against the clinician’s preference for simplification. This fact has motivated some authors to combine MMPS inhibitors or cross-linking agents in the etchants .
Among the cross-linking agents, proanthocyanidin (PA)-rich grape seed extract (GSE) is a promising agent due to its effectiveness under shorter treatment times and its absence of cytotoxicity . Additionally, PA can stabilize the resin–dentin bond strength of the adhesive interface and decrease the dentin-bound MMPs activity .
In previous work, the incorporation of PA into a 10% phosphoric acid showed promising results, rendering the demineralized dentin collagen inert to bacterial collagenase digestion . However, the authors did not evaluate the PA-etchant under clinically relevant bonding procedures, which prevent us from knowing whether or not the low concentrated PA-containing phosphoric acid is capable to promote an effective etching in enamel and dentin substrates, and if such treatment would produce stable resin–dentin bonds after water storage.
Therefore, the aim of this study was to evaluate the immediate and 6-month effectiveness of this modified phosphoric acid etchant in dentin and enamel through resin–dentin microtensile bond strength, resin–enamel microshear bond strength and nanoleakage studies. Additionally, the in situ MMP inhibition potential was also evaluated through in situ zymography .
2
Material and methods
2.1
Specimen preparation
A total of 26 extracted, caries-free, human third molars were used. The teeth were collected after obtaining the patients’ informed consent under a protocol approved by the Ethics Committee Review Board from the State University of Ponta Grossa (Parana, Brazil). The teeth were disinfected in 0.5% chloramine, stored in distilled water, and used within 6 months after extraction.
In 16 teeth, a flat occlusal dentin surface was exposed after wet grinding the occlusal enamel with #180-grit silicon-carbide (SiC) paper for 60 s. The exposed dentin surfaces were further polished with wet #600-grit SiC paper for 60 s to standardize the smear layer. Ten teeth were used for evaluation of the resin–dentin microtensile bond strength (μTBS) and nanoleakage, while the remaining six was used for the evaluation of the MMP-activity.
In 10 teeth, the roots of all teeth were removed by sectioning at the enamel-cementum junction. The dental crowns were then sectioned parallel to the long axis of the teeth to produce four enamel specimens (buccal, lingual, and proximals). Forty enamel specimens were ground wet with # 180 and 600-grit SiC paper for 60 s each. The unsanded surfaces were potted in acrylic resin to stabilize the specimens that were used for the evaluation of resin–enamel microshear bond strength (μSBS).
2.2
Experimental groups and restorative procedure
The tooth specimens were randomly distributed into the control and experimental groups by a person not involved in the research protocol using computer-generated tables. In the control group, the dentin and enamel surfaces of all specimens were conditioned with a 35% phosphoric acid gel for 15 s (Scotchbond etchant, 3 M ESPE, St. Paul, USA, batch number N261433). In the experimental group, an experimental etchant made of 2% PA, containing 10% phosphoric acid was prepared by mixing GSE powder, ethanol, distilled water, and 85% phosphoric acid to final concentrations (weight percentage with respect to total mass) of 2% PA-rich GSE, 20% ethanol and 10% phosphoric acid . The chemicals were purchased from Sigma–Aldrich (St. Louis, MO, USA), except for the GSE which was purchased from Mega Natural Gold (Polyphenolics, Madera, CA, USA). The experimental etchant was then applied to dentin or enamel for 30 s and then rinsed with water.
After conditioning, the etched surfaces were rinsed with distilled water for 30 s, air-dried for 5 s and kept slightly moist for the application of the two-step etch-and-rinse adhesive (Adper Single Bond Plus, 3 M ESPE, St. Paul, USA, batch number N531785) using a double application layer according to the manufacturer’s instructions. A composite resin (Filtek Z350, 3 M ESPE, St Paul, MN, USA) was used to create a build-up in two 2-mm increments and each one was light-cured for 40 s using an LED light curing (1200 mW/cm 2 ; Radii-cal, SDI Limited, Bayswater, Victoria, Australia). A radiometer (Demetron LED Radiometer, Kerr Sybron Dental Specialties, Middleton, WI, USA) was used to check the light intensity after every five restorations had been completed.
2.3
Resin–dentin microtensile bond strength (μTBS)
After water storage for 24 h at 37 °C, the restored teeth were longitudinally sectioned in both mesio-to-distal and buccal-to-lingual directions across the bonded interface, using a diamond saw mounted in a cutting machine (Isomet 1000, Buehler, Lake Bluff, USA). This procedure was performed to obtain resin–dentin sticks with a cross-sectional area of approximately 1 mm 2 . They were either tested immediately of after 6 months of storage in distilled water for 37 °C. The distilled water was changed weekly to maximize the degradation process .
The cross-sectional area of each stick was measured with a digital caliper (Absolute Digimatic, Mitutoyo, Tokyo, Japan) to the nearest 0.01 mm. Each bonded stick was attached to a jig for microtensile testing with cyanoacrylate resin (Super Bonder Gel, Loctite, São Paulo, Brazil) and subjected to a tensile force in a universal testing machine (Model 5565, Instron, Canton, OH, USA) at a crosshead speed of 0.5 mm/min. The failure modes were evaluated under stereomicroscopy at 100× magnification and classified as cohesive (within dentin or resin composite), adhesive (failure at resin–dentin interface, or adhesive/mixed (failure at resin–dentin interface with partial cohesive failure of the neighboring substrates).
2.4
Nanoleakage evaluation
Two resin-bonded sticks from each tooth at each storage period, and not used for microtensile testing, were randomly selected for nanoleakage evaluation. The sticks were immersed in 50 wt% ammoniacal silver nitrate solution in total darkness for 24 h. Thereafter, they were rinsed thoroughly in distilled water, and immersed in a photo-developing solution for 8 h under fluorescent light to reduce silver ions into metallic silver grains within voids along the bonded interface. Specimens were polished using 1000-, 1500-, 2000- and 2500-grit SiC papers and 1 and 0.25 μm diamond paste (Buehler Ltd., Lake Bluff, IL, USA) on polishing clothes. They were ultrasonically cleaned, air-dried, mounted on stubs and coated with evaporated carbon (MED 010, Balzers Union, Balzers, Liechtenstein).
The interfaces were observed in a scanning electron microscope (SEM) in the backscattered mode at 12 kV (VEGA 3 TESCAM, Shimadzu, Tokyo, Japan). Three images were taken from each specimen. The first image was obtained in the center of the stick, while the further two were obtained 0.3 mm left and 0.3 mm right from the first picture. A total of six images were obtained per tooth at each period (3 images × 2 bonded sticks). Thus, for each experimental condition, 30 images were evaluated per group (6 images × 5 teeth) . A blinded author to the experimental conditions took the pictures. The relative percentage of silver nitrate uptake within the hybrid layer was measured in all pictures using the ImageTool 3.0 software (Department of Dental Diagnostic Science, University of Texas Health Science Center, San Antonio, USA).
2.5
In situ zymography by CLSM
A dye quenched MMP substrate based on gelatin was prepared by means of a fluorescein isothiocyanate (FITC) hypersaturated gelatine. Five mg of FITC was dissolved in 2 mL 0.1 M sodium carbonate/bicarbonate buffer (pH 9.0, Sigma–Aldrich, Milwakee, WI, USA). This reactant was added dropwise to a 1 mg/mL gelatine solution in the dark and was incubated at room temperature for 2 h. The reacted FITC-gelatine conjugate was isolated from unbound FITC by means of a G-25 M Sephadex column. The fluorescein to protein ratio of >15 was confirmed from absorbance readings at 495 nm and 280 nm, respectively. This MMP-substrate was dissolved (0.3 wt%) distilled water, and this solution was actively applied for 60 s on the acid-etched dentin before the application of the bonding agents. Three teeth per group ( n = 3) were bonded as previously described and cut into 1 mm thick resin–dentin slabs and the interfaces were observed by confocal laser scanning microscopy (CLSM) similarly to a previous study . The specimens were examined using a CLSM (Leica SP5 CLSM, Heidelberg, Germany) equipped with a 63×/1.4 NA oil immersion lens using 468-nm laser illumination. The z-stack scans (one at each micrometer up to 20 μm below the surface) were compiled into single projections. Each resin–dentin interface was entirely characterized and the images were captured representing the MMP-activity observed along the bonded interfaces.
2.6
Resin–enamel microshear bond strength (μSBS)
Prior to applying the adhesive, each enamel specimen was mounted in a polyvinyl chloride ring filled with acrylic resin (AutoClear, DentBras, Pirassununga, São Paulo, Brazil). So that the sanded enamel surface was on the top of the cylinder. An acid-resistant, double-faced adhesive tape (Adelbras Ind e Com Adesivos Ltda, São Paulo, Brazil) was perforated with a Hygenic Ainsworth-style rubber-dam punch with a known surface area (Coltene, Alstätten, Switzerland) and bonded to the enamel surface Shimaoka et al. .
The enamel surfaces were acid-etched with their respective etchants, rinsed with water, and the adhesive applied according to the manufacturer’s directions. Then, polyethylene Tygon tubes (Tygon Medical Tubing Formulations 54-HL, Saint Gobain Performance Plastics, Akron, OH, USA) with an internal diameter of 0.8 mm and a height of 0.5 mm were positioned over the double-faced tape with the lumen coincident with the perforations. An operator trained in the μSBS technique positioned seven to nine tubes per surface, using magnifying loupes. Resin composite was carefully packed inside each tube, pressed gently into place and light-cured for 40 s using an LED light-curing (1200 mW/cm 2 ; Radii-cal, SDI Limited, Bayswater, Victoria, Australia).
After 24 h of storage in distilled water at 37 °C, the Tygon tubes and the double-faced adhesive tape were carefully removed with a surgical blade to expose the composite resin cylinders. Each specimen was examined under a stereomicroscope at 10× magnification. Specimens with evidence of air bubbles or gaps at the interface were discarded.
The specimens were attached to a shear-testing fixture (Odeme Biotechnology) so that each composite resin cylinder was tested in a universal testing machine (Kratos IKCL 3-USB, Kratos Equipamentos Industriais Ltda, Cotia, São Paulo, Brazil). A thin wire (0.2 mm diameter) was looped around the base of each composite cylinder to maintain the setup aligned (resin–enamel interface, the wire loop, and the center of the load cell) and ensure correct orientation of the shear forces . The shear load was applied at a crosshead of 1 mm/min until failure. One half of specimens were tested immediately and another half after 6 months of storage, as described previously for μTBS. The μSBS values were calculated by dividing the load at failure by the surface area (mm 2 ).
2.7
Statistical analysis
The μTBS values from the same dentin surface at each storage period and the μSBS values from the same enamel surface were averaged, so that the statistical unit was the tooth, not stick. Specimens that showed cohesive failures as well as the premature failures were not included in the tooth mean due to the low frequency of this fracture mode. The percentage of nanoleakage observed in specimens from the same tooth at each storage period was also averaged and only one value per tooth was taken to the statistical analysis.
The Kolmogorov–Smirnov test was performed to assess whether the data followed a normal distribution. The Barlett’s test was performed to evaluate the equality of variances. After observing the normality of the data distribution and the equality of the variances, the μTBS and the μSBS (MPa) and nanoleakage (%) data were submitted to a two-way repeated measures ANOVA and Tukey’s test. For all tests, the level of significance was pre-set in 5%.
2
Material and methods
2.1
Specimen preparation
A total of 26 extracted, caries-free, human third molars were used. The teeth were collected after obtaining the patients’ informed consent under a protocol approved by the Ethics Committee Review Board from the State University of Ponta Grossa (Parana, Brazil). The teeth were disinfected in 0.5% chloramine, stored in distilled water, and used within 6 months after extraction.
In 16 teeth, a flat occlusal dentin surface was exposed after wet grinding the occlusal enamel with #180-grit silicon-carbide (SiC) paper for 60 s. The exposed dentin surfaces were further polished with wet #600-grit SiC paper for 60 s to standardize the smear layer. Ten teeth were used for evaluation of the resin–dentin microtensile bond strength (μTBS) and nanoleakage, while the remaining six was used for the evaluation of the MMP-activity.
In 10 teeth, the roots of all teeth were removed by sectioning at the enamel-cementum junction. The dental crowns were then sectioned parallel to the long axis of the teeth to produce four enamel specimens (buccal, lingual, and proximals). Forty enamel specimens were ground wet with # 180 and 600-grit SiC paper for 60 s each. The unsanded surfaces were potted in acrylic resin to stabilize the specimens that were used for the evaluation of resin–enamel microshear bond strength (μSBS).
2.2
Experimental groups and restorative procedure
The tooth specimens were randomly distributed into the control and experimental groups by a person not involved in the research protocol using computer-generated tables. In the control group, the dentin and enamel surfaces of all specimens were conditioned with a 35% phosphoric acid gel for 15 s (Scotchbond etchant, 3 M ESPE, St. Paul, USA, batch number N261433). In the experimental group, an experimental etchant made of 2% PA, containing 10% phosphoric acid was prepared by mixing GSE powder, ethanol, distilled water, and 85% phosphoric acid to final concentrations (weight percentage with respect to total mass) of 2% PA-rich GSE, 20% ethanol and 10% phosphoric acid . The chemicals were purchased from Sigma–Aldrich (St. Louis, MO, USA), except for the GSE which was purchased from Mega Natural Gold (Polyphenolics, Madera, CA, USA). The experimental etchant was then applied to dentin or enamel for 30 s and then rinsed with water.
After conditioning, the etched surfaces were rinsed with distilled water for 30 s, air-dried for 5 s and kept slightly moist for the application of the two-step etch-and-rinse adhesive (Adper Single Bond Plus, 3 M ESPE, St. Paul, USA, batch number N531785) using a double application layer according to the manufacturer’s instructions. A composite resin (Filtek Z350, 3 M ESPE, St Paul, MN, USA) was used to create a build-up in two 2-mm increments and each one was light-cured for 40 s using an LED light curing (1200 mW/cm 2 ; Radii-cal, SDI Limited, Bayswater, Victoria, Australia). A radiometer (Demetron LED Radiometer, Kerr Sybron Dental Specialties, Middleton, WI, USA) was used to check the light intensity after every five restorations had been completed.
2.3
Resin–dentin microtensile bond strength (μTBS)
After water storage for 24 h at 37 °C, the restored teeth were longitudinally sectioned in both mesio-to-distal and buccal-to-lingual directions across the bonded interface, using a diamond saw mounted in a cutting machine (Isomet 1000, Buehler, Lake Bluff, USA). This procedure was performed to obtain resin–dentin sticks with a cross-sectional area of approximately 1 mm 2 . They were either tested immediately of after 6 months of storage in distilled water for 37 °C. The distilled water was changed weekly to maximize the degradation process .
The cross-sectional area of each stick was measured with a digital caliper (Absolute Digimatic, Mitutoyo, Tokyo, Japan) to the nearest 0.01 mm. Each bonded stick was attached to a jig for microtensile testing with cyanoacrylate resin (Super Bonder Gel, Loctite, São Paulo, Brazil) and subjected to a tensile force in a universal testing machine (Model 5565, Instron, Canton, OH, USA) at a crosshead speed of 0.5 mm/min. The failure modes were evaluated under stereomicroscopy at 100× magnification and classified as cohesive (within dentin or resin composite), adhesive (failure at resin–dentin interface, or adhesive/mixed (failure at resin–dentin interface with partial cohesive failure of the neighboring substrates).
2.4
Nanoleakage evaluation
Two resin-bonded sticks from each tooth at each storage period, and not used for microtensile testing, were randomly selected for nanoleakage evaluation. The sticks were immersed in 50 wt% ammoniacal silver nitrate solution in total darkness for 24 h. Thereafter, they were rinsed thoroughly in distilled water, and immersed in a photo-developing solution for 8 h under fluorescent light to reduce silver ions into metallic silver grains within voids along the bonded interface. Specimens were polished using 1000-, 1500-, 2000- and 2500-grit SiC papers and 1 and 0.25 μm diamond paste (Buehler Ltd., Lake Bluff, IL, USA) on polishing clothes. They were ultrasonically cleaned, air-dried, mounted on stubs and coated with evaporated carbon (MED 010, Balzers Union, Balzers, Liechtenstein).
The interfaces were observed in a scanning electron microscope (SEM) in the backscattered mode at 12 kV (VEGA 3 TESCAM, Shimadzu, Tokyo, Japan). Three images were taken from each specimen. The first image was obtained in the center of the stick, while the further two were obtained 0.3 mm left and 0.3 mm right from the first picture. A total of six images were obtained per tooth at each period (3 images × 2 bonded sticks). Thus, for each experimental condition, 30 images were evaluated per group (6 images × 5 teeth) . A blinded author to the experimental conditions took the pictures. The relative percentage of silver nitrate uptake within the hybrid layer was measured in all pictures using the ImageTool 3.0 software (Department of Dental Diagnostic Science, University of Texas Health Science Center, San Antonio, USA).
2.5
In situ zymography by CLSM
A dye quenched MMP substrate based on gelatin was prepared by means of a fluorescein isothiocyanate (FITC) hypersaturated gelatine. Five mg of FITC was dissolved in 2 mL 0.1 M sodium carbonate/bicarbonate buffer (pH 9.0, Sigma–Aldrich, Milwakee, WI, USA). This reactant was added dropwise to a 1 mg/mL gelatine solution in the dark and was incubated at room temperature for 2 h. The reacted FITC-gelatine conjugate was isolated from unbound FITC by means of a G-25 M Sephadex column. The fluorescein to protein ratio of >15 was confirmed from absorbance readings at 495 nm and 280 nm, respectively. This MMP-substrate was dissolved (0.3 wt%) distilled water, and this solution was actively applied for 60 s on the acid-etched dentin before the application of the bonding agents. Three teeth per group ( n = 3) were bonded as previously described and cut into 1 mm thick resin–dentin slabs and the interfaces were observed by confocal laser scanning microscopy (CLSM) similarly to a previous study . The specimens were examined using a CLSM (Leica SP5 CLSM, Heidelberg, Germany) equipped with a 63×/1.4 NA oil immersion lens using 468-nm laser illumination. The z-stack scans (one at each micrometer up to 20 μm below the surface) were compiled into single projections. Each resin–dentin interface was entirely characterized and the images were captured representing the MMP-activity observed along the bonded interfaces.
2.6
Resin–enamel microshear bond strength (μSBS)
Prior to applying the adhesive, each enamel specimen was mounted in a polyvinyl chloride ring filled with acrylic resin (AutoClear, DentBras, Pirassununga, São Paulo, Brazil). So that the sanded enamel surface was on the top of the cylinder. An acid-resistant, double-faced adhesive tape (Adelbras Ind e Com Adesivos Ltda, São Paulo, Brazil) was perforated with a Hygenic Ainsworth-style rubber-dam punch with a known surface area (Coltene, Alstätten, Switzerland) and bonded to the enamel surface Shimaoka et al. .
The enamel surfaces were acid-etched with their respective etchants, rinsed with water, and the adhesive applied according to the manufacturer’s directions. Then, polyethylene Tygon tubes (Tygon Medical Tubing Formulations 54-HL, Saint Gobain Performance Plastics, Akron, OH, USA) with an internal diameter of 0.8 mm and a height of 0.5 mm were positioned over the double-faced tape with the lumen coincident with the perforations. An operator trained in the μSBS technique positioned seven to nine tubes per surface, using magnifying loupes. Resin composite was carefully packed inside each tube, pressed gently into place and light-cured for 40 s using an LED light-curing (1200 mW/cm 2 ; Radii-cal, SDI Limited, Bayswater, Victoria, Australia).
After 24 h of storage in distilled water at 37 °C, the Tygon tubes and the double-faced adhesive tape were carefully removed with a surgical blade to expose the composite resin cylinders. Each specimen was examined under a stereomicroscope at 10× magnification. Specimens with evidence of air bubbles or gaps at the interface were discarded.
The specimens were attached to a shear-testing fixture (Odeme Biotechnology) so that each composite resin cylinder was tested in a universal testing machine (Kratos IKCL 3-USB, Kratos Equipamentos Industriais Ltda, Cotia, São Paulo, Brazil). A thin wire (0.2 mm diameter) was looped around the base of each composite cylinder to maintain the setup aligned (resin–enamel interface, the wire loop, and the center of the load cell) and ensure correct orientation of the shear forces . The shear load was applied at a crosshead of 1 mm/min until failure. One half of specimens were tested immediately and another half after 6 months of storage, as described previously for μTBS. The μSBS values were calculated by dividing the load at failure by the surface area (mm 2 ).
2.7
Statistical analysis
The μTBS values from the same dentin surface at each storage period and the μSBS values from the same enamel surface were averaged, so that the statistical unit was the tooth, not stick. Specimens that showed cohesive failures as well as the premature failures were not included in the tooth mean due to the low frequency of this fracture mode. The percentage of nanoleakage observed in specimens from the same tooth at each storage period was also averaged and only one value per tooth was taken to the statistical analysis.
The Kolmogorov–Smirnov test was performed to assess whether the data followed a normal distribution. The Barlett’s test was performed to evaluate the equality of variances. After observing the normality of the data distribution and the equality of the variances, the μTBS and the μSBS (MPa) and nanoleakage (%) data were submitted to a two-way repeated measures ANOVA and Tukey’s test. For all tests, the level of significance was pre-set in 5%.
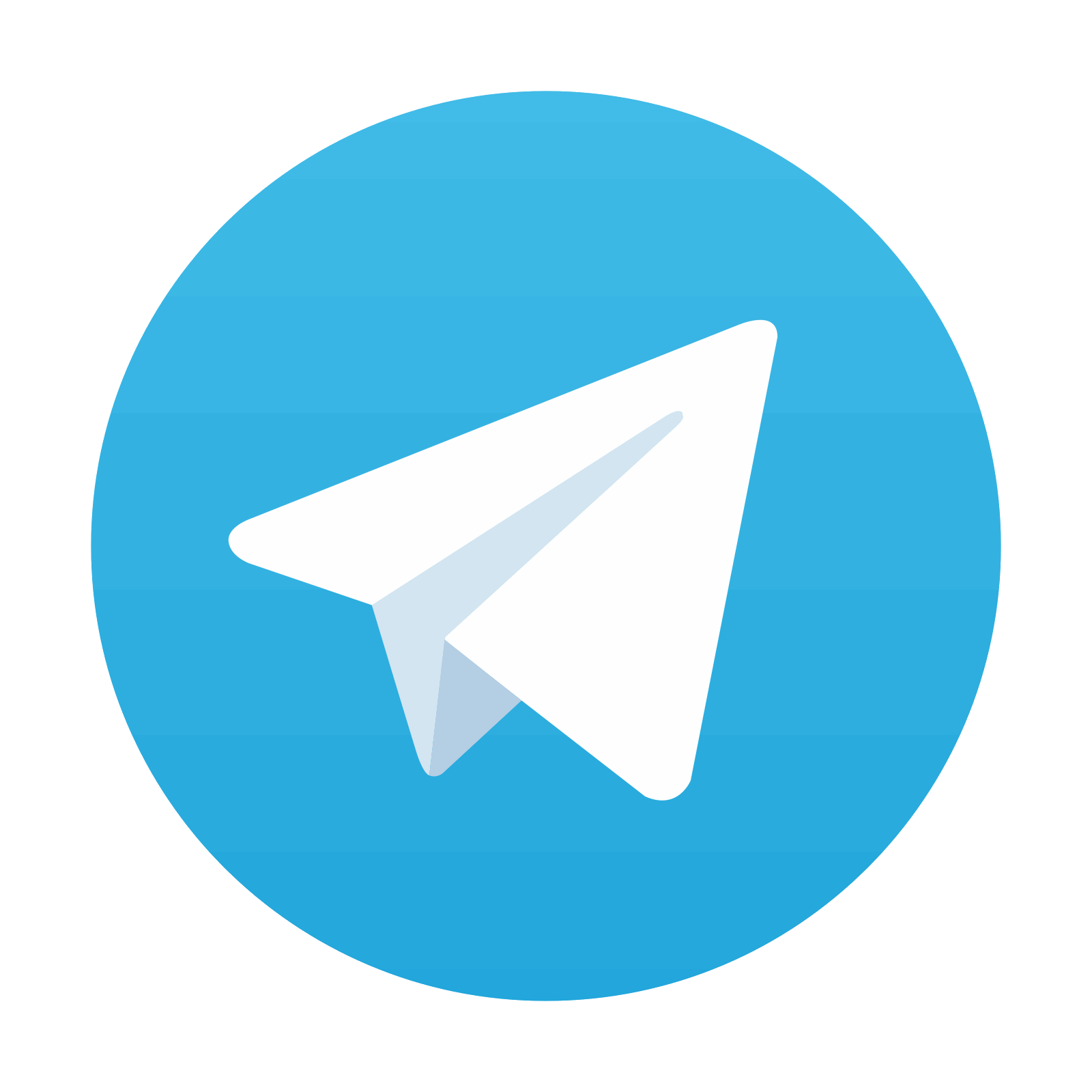
Stay updated, free dental videos. Join our Telegram channel

VIDEdental - Online dental courses
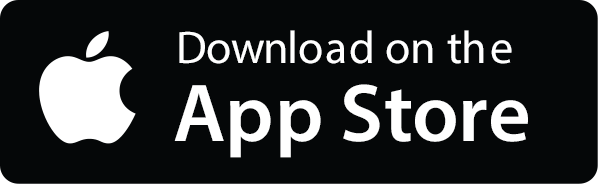
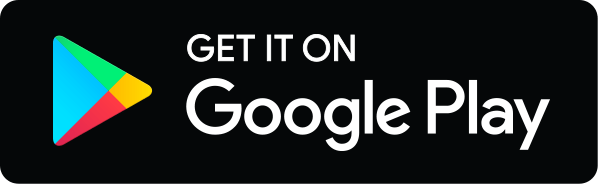