Abstract
Objectives
The purpose of this study was to evaluate the effect of preparation order on the crystal structure of yttria-stabilized tetragonal zirconia polycrystal (Y-TZP) and the shear bond strength of dental resin cements.
Methods
One-hundred fifty pre-sintered Y-TZP cylinders ( Ø 9 mm × 13.5 mm) were prepared and divided into three groups (control group, SBS group and SAS group). Specimens in control group were not treated. Specimens in SBS group were sandblasted and then densely sintered, and specimens in SAS group were sintered in advance, and then sandblasted. The specimens were analyzed by X-ray diffractometry, confocal laser scanning microscopy and Energy dispersive X-ray spectroscopy before and after sandblasting. All specimens were embedded in polytetrafluoroethylene (PTFE) molds using PMMA and each group was divided into five subgroups. The mixed resin cements (Clearfil SA luting cement, Zirconite, Superbond C&B, Rely-X Unicem, and Multilink) were placed onto the Y-TZP surfaces using PTFE molds with Ø 3 mm × 3 mm, followed by storage in distilled water at 37 °C for 24 h, and thermocycling (5000 cycles at 5 °C and 55 °C with a 30 s dwelling time). All specimens were tested for the shear bond strengths with a universal testing machine, and fractured surfaces were evaluated by SEM. Statistical analysis was performed using one-way ANOVA and Scheffé comparison with α = .05.
Results
Sandblasting of the zirconia significantly increased shear bond strength of resin cements, but the preparation order had no significant influence on the shear bond strength in both test groups. In SEM observation, the natures of the surface faceting of the zirconia grains were totally different between SBS and SAS groups. SBS group exhibited less monoclinic structures than SAS group.
Significance
Sandblasting of pre-sintered Y-TZP and then sintering may induce favorable proportion of tetragonal structures. This might have positive effect on the clinical performance of zirconia restorations.
1
Introduction
Fixed partial denture materials are changing from porcelain fused to metal to all-ceramic systems due to their biocompatibility and esthetics . The changes have been encouraged by the advent of zirconia with high fracture toughness . Pure zirconia exists as a monoclinic ( m ) crystal structure at room temperature and transforms to tetragonal ( t ) structure at 1173 °C and cubic structure at 2370 °C. When zirconia is cooled down, its volume increases with the cubic or tetragonal to monoclinic transformation. This induces very large stresses within the material, and causes the zirconia to crack . For dental applications, zirconia can be stabilized by the addition of 3 mol% yttrium oxide. As a results, yttria-stabilized tetragonal zirconia polycrystalline (Y-TZP) is metastable in its tetragonal crystalline at room temperature . However, when the high stresses are applied to Y-TZP, the tetragonal phase is converted to monoclinic with 4–5% volume increase .
Sintered zirconia surface should be treated for the micromechanical retention of dental resin cements. Unlike glass ceramics, zirconia is not prone to hydrofluoric acid etching for surface treatment due to the polycrystalline and glass-free structures . Thus, air-particle abrasion (sandblasting) has been suggested to roughen the zirconia surface . However, due to the metastability of tetragonal zirconia, the surface treatment is responsible to trigger the transformation from tetragonal to monoclinic structure. This causes the strength reduction and the enhanced fracture tendency in zirconia because of the missing transformation capacity during critical loading. This is related to the amount of transformation, and may also damage the long-term lifespan of zirconia ceramics .
In general, the surface treatment for cementation is carried out after sintering of the zirconia. This may induce increased monoclinic phase of the zirconia. In order to reduce the monoclinic phase, an additional heat treatment should be done. Hence, it could be suggested that sandblasting of the pre-sintered zirconia in advance and then sintering procedure might generate less amount of monoclinic structures than sandblasting after sintering procedure. The purpose of this in vitro study was to evaluate the effect of preparation order on the crystal structure of zirconia and on the shear bond strengths of resin cements to zirconia ceramics. The two null hypotheses were that there is no difference (i) in the composition of crystal structures and (ii) in the shear bond strength of resin cements by preparation order on zirconia.
2
Materials and methods
2.1
Preparations of the specimens
The schematic test protocol used in this study is shown in Fig. 1 . Specimens of 150 pre-sintered Y-TZP cylinders – which consisted of 88–96% ZrO 2 , 4–6% Y 2 O 3 and <1% Al 2 O 3 – (Rainbow, Dentium, Seoul, Korea) of 9 mm diameter and 13.5 mm height were prepared and randomly divided into 3 groups. Control group (No Sandblasting; NS group) was not treated. One test group (Sandblasting Before Sintering; SBS group) was prepared with Al 2 O 3 sandblasting in advance and then densely sintered at 1500 °C for 2 h. The other test group (Sandblasting After Sintering; SAS group) was densely sintered in advance at the same condition, and then sandblasted. Air-borne particle abrasion was done making circular movements at a standoff distance of 10 mm with 3 bar pressure for 10 s. SAS group was sandblasted with 50 μm grain size, but SBS group was done with 70 μm grain size for the similar surface characteristics of the test groups, because pre-sintered Y-TZP tend to undergo their linear shrinkage of up to circa 20% during sintering . All specimens were cleaned ultrasonically with distilled water in 5 min and rinsed for 1 min. The size of Y-TZP cylinder after densely sintering was 7 mm in diameter and 11 mm in height.

2.2
X-ray diffractometry
Before and after the sandblasting, the specimens were examined by X-ray diffractometry (XRD, Rigaku/JP-D/MAX-2500H, Berlin, Germany). XRD data was collected with 2 diffractometer using the Cu-Kα radiation. Diffractogram was obtained from 20° to 70°, at a scan speed of 5°/min and a step size of 0.004° covering the location of the highest peaks of t and m ZrO 2 phases. The relative amount of transformed m structure ( X M ) on the zirconia specimens’ surfaces was calculated according to the method of Garvie and Nicholson .
2.3
Bonding procedure
Specimens of each group were randomly divided into 5 subgroups depending on each kind of resin cement applied ( N = 150, n = 10). The abbreviations of the experimental groups, preparation order and the used resin luting cements are presented in Table 1 .
Group | Preparation order | Subgroup code | Resin cement |
---|---|---|---|
NS (Control) | No sandblasting | NSC | Clearfil SA luting cement |
NSZ | Zirconite | ||
NSR | Rely-X Unicem | ||
NSS | Superbond C&B | ||
NSM | Multilink | ||
SBS | Sandblasting before sintering | SBSC | Clearfil SA luting cement |
SBSZ | Zirconite | ||
SBSR | Rely-X Unicem | ||
SBSS | Superbond C&B | ||
SBSM | Multilink | ||
SAS | Sandblasting after sintering | SASC | Clearfil SA luting cement |
SASZ | Zirconite | ||
SASR | Rely-X Unicem | ||
SASS | Superbond C&B | ||
SASM | Multilink |
Five commercial resin cements were used ( Table 2 ). Three of them were dual-curing resin cements: Clearfil SA luting cement, Zirconite and Rely X Unicem and two were chemical-curing resin cements: Superbond C&B and Multilink.
Material | Type | Zirconia primer | Characteristics | Batch No. | Manufacturer |
---|---|---|---|---|---|
Clearfil SA luting cement | Dual-curing | × | Paste A: Bis-GMA, TEGDMA, MDP, DMA, Silanated barium glass filler, Silanated colloidal silica | ||
Paste B: Bis-GMA, DMA, silanated barium glass filler, silanated colloidal silica, surface treated sodium fluoride | 143BA | Kuraray Medical Co., Osaka, Japan | |||
Zirconite | Dual-curing | × | Paste A: DMA, Methacrylated phosphoric acid esters, MPTMS, Fumed Silica | 4146HQBARCZ | BJM Lab.,Or-Yehuda, Israel |
Paste B: Bis-GMA, TEGMA, 4-META | |||||
Rely-X Unicem | Dual-curing | × | Powder: glass particles, initiators, silica, substituted pyrimidine, calcium hydroxide, peroxide composite and pigment | 3922517 | 3 M ESPE, St Paul, MN, USA |
Liquid: methacrylate phosphoric acid ester, DMA, acetate, stabilizer and initiator. | |||||
Superbond C&B | Self-curing | Porcelain Liner M | Powder: PMMA, Liquid: MMA, 4-META | RE1 | Sun Medical, Shiga, Japan |
Catalyst: TBB | |||||
+ | |||||
Liquid A: MMA, 4-META | TG1 | ||||
Liquid B: MMA, TMSPM | (Primer) | ||||
Multilink | Self-curing | Metal/zirconia primer | Paste A: DMA, HEMA, fillers, BPO | NO7559 | |
Paste B: DMA, HEMA, fillers, t-amine | IvoclarVivadent, Schaan, Lichtenstein | ||||
+ | M63563 | ||||
DMA, phosphonic acid acrylate, initiator and stabilizer | (Primer) |
All specimens were embedded in polytetrafluoroethylene (PTFE) molds (9 mm in inner diameter, 20 mm in outer diameter, and 11 mm in height) using polymethyl methacrylate (PMMA; Vertex-Dental, Dentimex, Zeist, Netherlands), constructing that one surface of the zirconia cylinder remained uncovered for adhering to the resin cement ( Fig. 2 a) .

A PTFE ring with a hole (3 mm in inner diameter and 3 mm in height) was positioned on the uncovered zirconia surface. Base and catalyst of the resin cement were mixed and packed into the hole of the PTFE ring and then cured according to the manufacturers’ recommendations. For specimens using Superbond C&B and Multilink, the corresponding primers (Porcelain Liner M and Metal/Zirconia Primer, respectively) were used. The primer was coated in a layer as thin as possible with a disposable brush. The PTFE ring was gently separated one side from the other 30 min after mixing the resin cement ( Fig. 2 b).
All specimens were stored in distilled water for 24 h at 37 °C, followed by thermocycling (5000 cycles at 5 °C and 55 °C, with a 30 s dwelling time in each bath and 2 s of transferring time) .
2.4
Shear bond strength test
Specimens were mounted in the jig of a universal testing machine (Model 3345, Instron, Canton, MA, USA) and were loaded in shear at a constant crosshead speed of 0.5 mm/min until fracture. Maximum load to failure was recorded by the corresponding software in Newtons, and the shear bond strength was calculated in MPa.
2.5
Scanning electron microscopy (SEM), confocal laser scanning microscopy (CLSM) and energy dispersive X-ray spectroscopy (EDX)
Randomly selected specimens from all groups were gold-coated with a sputter coater (SC7620 Mini Sputter Coater, Polaron, Schwalbach, Germany) and then mounted on coded brass stubs and examined using the scanning electron microscope (VEGA II LSH, TESCAN, Brno, Czech Republic) at 180× and 5000× magnification and 20 kV accelerating voltage. Pre-sintered Y-TZP surfaces, sandblasted surfaces with 70 μm Al 2 O 3 , and surfaces after sintering were examined. In addition, sandblasted specimens with 50 μm Al 2 O 3 after sintering were also examined, and were compared with former specimens.
Confocal laser scanning microscopy (CLSM, LSM 5 Pascal, Carl Zeiss Microscopy, Göttingen, Germany) was performed to confirm the similarity of the depths and widths of the scratches in last specimens of SBS group and SAS group. A 543 nm (1 mW) HeNe laser was used as a light source, and the specimens were observed at 200× magnification. The measuring area was 450 μm × 450 μm, and the height of the z -stack was 30 μm in 1 μm intervals.
The fractured interfacial surfaces of the specimens were also examined using SEM at 50× and 2000× magnification, and micro-analyzed by Energy dispersive X-ray spectroscopy (EDX, Horiba EX-250, Tokyo, Japan).
2.6
Statistical analysis
Statistical analyses were performed by using the statistical software (PASW Statistics 18.0 IBM Acquires SPSS Inc., Chicago, IL, USA). One-way analysis of variance and multiple comparison Scheffé post hoc tests were performed to verify the effect of preparation order, and two-sample t -tests were used to detect the differential effect of cements. The test was performed at a significance level of 0.05.
2
Materials and methods
2.1
Preparations of the specimens
The schematic test protocol used in this study is shown in Fig. 1 . Specimens of 150 pre-sintered Y-TZP cylinders – which consisted of 88–96% ZrO 2 , 4–6% Y 2 O 3 and <1% Al 2 O 3 – (Rainbow, Dentium, Seoul, Korea) of 9 mm diameter and 13.5 mm height were prepared and randomly divided into 3 groups. Control group (No Sandblasting; NS group) was not treated. One test group (Sandblasting Before Sintering; SBS group) was prepared with Al 2 O 3 sandblasting in advance and then densely sintered at 1500 °C for 2 h. The other test group (Sandblasting After Sintering; SAS group) was densely sintered in advance at the same condition, and then sandblasted. Air-borne particle abrasion was done making circular movements at a standoff distance of 10 mm with 3 bar pressure for 10 s. SAS group was sandblasted with 50 μm grain size, but SBS group was done with 70 μm grain size for the similar surface characteristics of the test groups, because pre-sintered Y-TZP tend to undergo their linear shrinkage of up to circa 20% during sintering . All specimens were cleaned ultrasonically with distilled water in 5 min and rinsed for 1 min. The size of Y-TZP cylinder after densely sintering was 7 mm in diameter and 11 mm in height.
2.2
X-ray diffractometry
Before and after the sandblasting, the specimens were examined by X-ray diffractometry (XRD, Rigaku/JP-D/MAX-2500H, Berlin, Germany). XRD data was collected with 2 diffractometer using the Cu-Kα radiation. Diffractogram was obtained from 20° to 70°, at a scan speed of 5°/min and a step size of 0.004° covering the location of the highest peaks of t and m ZrO 2 phases. The relative amount of transformed m structure ( X M ) on the zirconia specimens’ surfaces was calculated according to the method of Garvie and Nicholson .
2.3
Bonding procedure
Specimens of each group were randomly divided into 5 subgroups depending on each kind of resin cement applied ( N = 150, n = 10). The abbreviations of the experimental groups, preparation order and the used resin luting cements are presented in Table 1 .
Group | Preparation order | Subgroup code | Resin cement |
---|---|---|---|
NS (Control) | No sandblasting | NSC | Clearfil SA luting cement |
NSZ | Zirconite | ||
NSR | Rely-X Unicem | ||
NSS | Superbond C&B | ||
NSM | Multilink | ||
SBS | Sandblasting before sintering | SBSC | Clearfil SA luting cement |
SBSZ | Zirconite | ||
SBSR | Rely-X Unicem | ||
SBSS | Superbond C&B | ||
SBSM | Multilink | ||
SAS | Sandblasting after sintering | SASC | Clearfil SA luting cement |
SASZ | Zirconite | ||
SASR | Rely-X Unicem | ||
SASS | Superbond C&B | ||
SASM | Multilink |
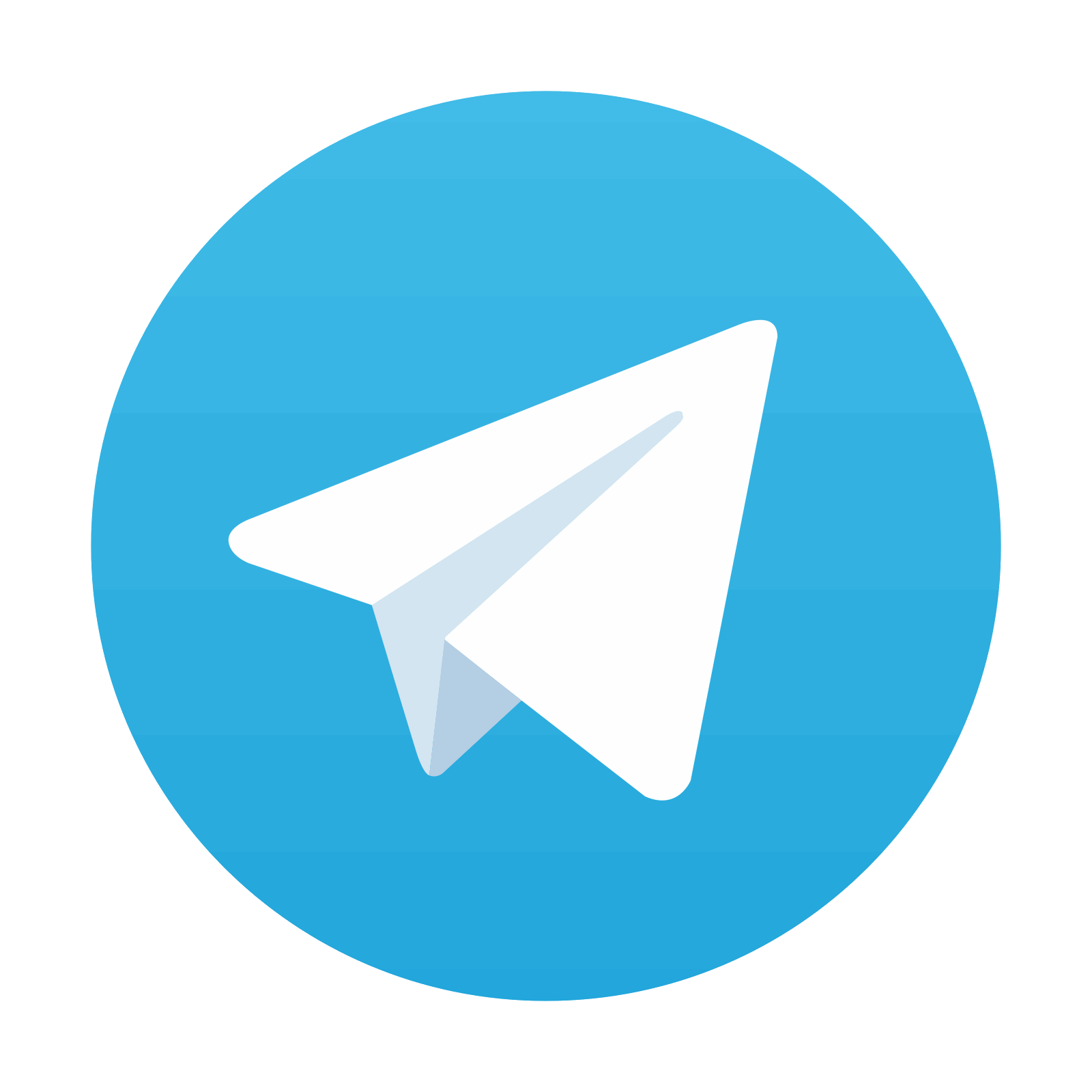
Stay updated, free dental videos. Join our Telegram channel

VIDEdental - Online dental courses
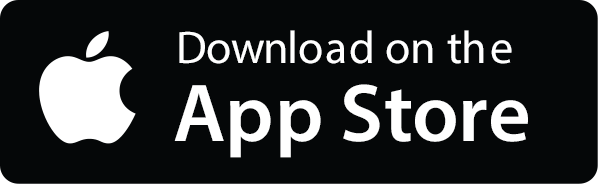
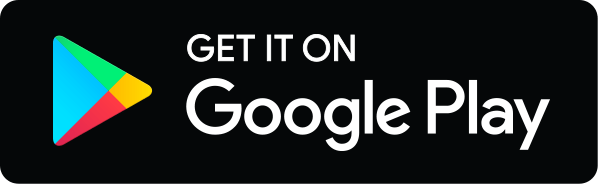
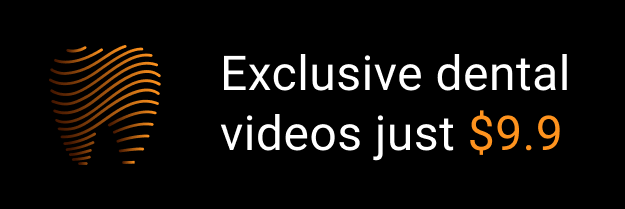