Abstract
Objective
To evaluate the efficacy on flexural properties of flowable dental resin composite reinforced with short glass fiber of different aspect ratios (ARs) and volume percent loadings. It is hypothesized that with the addition of randomly oriented fibers it is possible to significantly improve flexural strength and modulus while maintaining flowability.
Methods
Ten groups of samples with varying glass fiber volume loads (0, 5%, 10%, 20%, 40% and 60%) and three different ARs (5.2, 68 and 640) were tested in three point bending to fracture according to ISO 4049. A flowable resin composite was used as the control and also as the filled resin composite that was subsequently reinforced with fibers. Load deflection results were used to calculate flexural strength and flexural modulus. SEM images were used to determine the mode(s) of failure, to describe surface features of reinforcement and were correlated with force displacement graphs. All results were statistically analysed using ANOVA followed by post hoc Tukey’s test. Level of significance was set at 0.05.
Results
When compared to the “sculptable” control (68.6 vol% filler loaded) results for flexural strength varied from a mean reduction of 42% ( p > 0.05) for the low AR group to an increase of 77% ( p < 0.001) for the high AR samples. Flexural modulus results varied from a low of 6.6 [0.67] GPa for the non reinforced spatulated control to 20.3 [1.31] GPa ( p < 0.001) for the 60% loaded low AR group. The low fiber loaded mid AR group was still flowable with 49% total loading (5% fiber/44% filler) but gave strength values (181.2 [33.5] MPa) 30% higher than the “sculptable” control ( p > 0.05) and comparable modulus.
Significance
This study shows that short and very short glass fibers can significantly reinforce flowable dental composite. The fiber’s aspect ratio was shown to be more important than volume loading for flexural strength. It appears possible to produce a light cured short glass fiber reinforced flowable material with superior flexural properties compared to conventional universal composites.
1
Introduction
Whether driven by public esthetic demands, concerns over amalgam’s toxicity or trends toward conservative management of dentitions, dental composites have become the material most often employed in the restoration of teeth. These materials are composed of varying amounts of inorganic radio opaque particulate fillers dispersed in a relatively weak organic resin matrix. Bi-functional silane compounds are used to create the interphase between the two. These materials are manipulated directly in the mouth and are set with curing lights of approximately 470 nm wavelength. Their use in stress bearing areas of posterior teeth is becoming an acceptable option but brittleness and a tendency to edge fracture remain important limitations .
There has been a long and successful history of high performance fiber reinforcement of industrial composites. This cannot be said of dental composites. Although relatively uncommon clinically, the introduction of fibers have been studied in fixed and removable dental prostheses . These fiber systems though are usually continuous and are indirectly manufactured . Compared with direct procedures these restoratives require more aggressive tooth preparation. Other limiting factors include significant laboratory fees and the increased probability of handling and processing errors. Chair side reinforcement with continuous fiber composite is still less common. This direct procedure involves combining regular dental composite with sections cut from straight or woven ultra high molecular weight polyethylene fibers. Not only has fiber reinforcement been shown to strengthen and toughen dental composite but direct reinforcing was also found to strengthen restored teeth . Additionally, the fracture type changes from a catastrophic brittle pattern to a more graceful controlled one.
Least common are dental composites reinforced with randomly oriented short fibers. These materials claim higher stiffness, fracture toughness and flexural strength. For example: 16 GPa, 3.2 MN/m 3/2 and 176 MPa respectively for a 71% volume filled, 20–800 μm long E glass rod material . They also claim very low polymerization shrinkage but have been found to exhibit poor wear properties due to “plucking” of the relatively large surface exposed fibers and fillers .
Variables such as fiber position and orientation have been studied . Using chopped glass fibers in denture polymers Karacaer found significant correlation between fiber volume with both flexural modulus and impact strength. Callaghan found that increasing the length of the fibers increased the wear resistance of reinforced specimens.
The intent of this study was to evaluate the effect of different fiber aspect ratios and fiber volume loads on the flexural strength and modulus of low viscosity or flowable dental composites. Fiber aspect ratio (AR) is simply the relationship of length to diameter of a fiber given as a number. Some studies have quantified AR as a variable for mechanical properties of dental materials but overall there exists very little research in this field and none that we know of with regards to flowable dental materials. The hypothesis evaluated was that a flowable dental composite may be significantly reinforced by incorporating randomly distributed short and very short glass fibers. Further it was hypothesized that, the amount of fiber required to increase the flexural behavior is small thereby retaining low viscosity and flowability.
2
Materials and methods
Ten groups (A–J) with ten samples per group were formed. Three control groups A, B and C were not reinforced while groups D–J formed the fiber reinforced samples ( Table 1 ). Group A consisted of a universal high viscosity or “sculptable” dental composite (Beautifil II, Shofu, Kyoto, Japan) while groups B and C consisted of a low viscosity flowable composite (Beautifil F03 Flow Plus). Groups D–F were formed by combining this flowable material (F03 Flow Plus) with low (5.2) aspect ratio fibers of different volume load fractions (20, 40 and 60%). Groups G, H and I combined F03 Flow Plus with mid (68) aspect ratio fibers of three different loads (5, 10 and 20%) while group J utilized high aspect ratio (640) fibers with 10% loading.
2.1
Fibers
Glass fibers with three different aspect ratios (ARs) were used ( Fig. 1 ). Low AR fibers presented as unsized milled E glass powder from Owens Corning, Ohio, USA. SEM analysis revealed an average length of 89 ± 62 μm (range 14–300, n = 400), an average diameter of 17 μm and an AR of 5.2 ± 3.65. They were used for three groups (D–F). High AR glass fibers presented as S glass ( S-2 Glass ) chopped strands from AGY, South Carolina, USA and consisted of numerous 10 μm diameter parallel filaments cut into short 6.4 mm lengths. These fibers have an AR of 640 without deviation and were used for group J. These S glass strands were also cut (average length 679, range 60–2400, n = 400) to create the third AR of 68 ± 43.5 used in 3 groups (G–I).

Both E glass and S glass fibers were used as the availability of very short cut or milled glass fibers is very limited. The prefix E and S stands for electrical grade and structural grade. Their physical properties are as follows: tensile strengths 3.4 GPa vs. 4.9 GPa, moduli 72 GPa vs. 87 GPa and densities of 2.58 g/cc vs. 2.46 g/cc respectively. Both fibers are predominantly aluminosilicate glasses. E glass is high in calcium while S glass is high in magnesium with no boron . Given the similarity in composition, physical properties and surface chemistry it was decided to use milled E glass as a low AR baseline. This material is readily available and is used by manufacturers of “packable” DRCs. The S glass fibers were used uncut in the higher AR sample group. The major difference between the two glasses was thus considered to be diameter and a sufficient spread of aspect ratios was achieved.
All fibers were first etched in a 4% potassium dichromate(IV)(K 2 Cr 2 O 7 )/89% sulphuric acid (H 2 SO 4 ) aqueous solution (Australian Scientific, NSW, Australia) for 4 h then thoroughly washed (until pH returned to 7) in deionized water followed by rinsing in ethanol and drying in an oven at 50 °C for 8 h. Fibers were then immersed in a hydrolyzed silane solution consisting of 2% 3-(Trimethyoxysilyl) propyl methacrylate silane (Z-6030 Sigma–Aldrich, MO, USA) in 93% ethanol and 5% deionized water. This solution was titrated to pH 5 with acetic acid. The fibers in solution were stirred for 2 min every 5 min for a total of 44 min before being rinsed several times in deionized water. In order to remove any physisorbed and some of the weakly chemisorbed layers the fibers were then conditioned in 100 °C deionized water for 10 min and then dried at 50 °C for 24 h .
2.2
Dental resin composite
The dental composite used in this study Beautifil is classified as a giomer and is composed of BisGMA/TEGDMA resin and pre-reacted glass-ionomer (S-PRG) fluoroaluminosilicate glass filler particles ranging in size from 0.01 to 4.0 (ave. 0.8) μm. Manufacturers of these materials claim fluoride release and recharge capabilities while maintaining superior physical properties to poly acid modified resin composites (compomers) .
This material is manufactured with five viscosities depending on filler ratio ranging from 68.6% volume (83.3% by weight) loaded “sculptable” Beautifil II (group A) to the very low viscosity Beautifil Flowable F10 (<47% by volume). Beautifil Flow Plus F03 (shade A2 lot number 111004) with 46.3% by volume (67.3% weight) filler loading and mid viscosity was chosen as the main control (group B) and also as the resin composite to be reinforced with glass fiber as it exhibited good strength at low viscosity.
2.3
Sample preparation
All samples were prepared according to ISO 4049. A split brass mold (2 mm × 2 mm × 25 mm) with glass top and base was used. The samples were cured using a Flash Max P3 460 (CMS Dental ApS, Copenhagen, Denmark) curing light according to manufacturer’s instruction (3 s) with six overlapping cures from both top and bottom slides. A standard light output meter (range 0–2000 mW) showed light output to be above 2 W. The packable control was dispensed onto a paper mixing pad before packing into the mold. The flowable composite was dispensed directly from the manufacturers syringe dispensing tip (internal Ø = 600 μm) into the split metal mold while all reinforced samples were mixed individually on the paper mixing pad with a metal spatula for 20 s prior to packing into the mold. To assess the effect of mixing on the samples another control group C was prepared by first dispensing the flowable resin composite F03 onto the pad and then spatulating for 10 s before loading into the mold.
The weight of fiber needed for each sample was found by using the densities of the two fibers used and also the DRC (1.80 g/cc). Density ( ρ ) equals weight in grams divided by volume in cubic centimeters. Therefore,
Volume = Weight Density
and since,
% V f = V f V f + V r
where V f + V r = Total Sample Volume
Therefore,
% V f = W f / ρ f Total Sample Volume
Fibers for each test sample were thus weighed according to the following formula:
W f = % V f × ρ f × Total Sample Volume ( TSV )
(where W f is the weight of fiber in grams, % V f is the percentage volume fiber in sample and ρ f is the density of fiber in grams/cubic centimeter, TSV = 2 mm × 2 mm × 25 mm = 0.1 cm 3 ) before being mixed into the individually weighed flowable composite. Samples were stored in deionized water at 37 °C for 24 h prior to testing.
2
Materials and methods
Ten groups (A–J) with ten samples per group were formed. Three control groups A, B and C were not reinforced while groups D–J formed the fiber reinforced samples ( Table 1 ). Group A consisted of a universal high viscosity or “sculptable” dental composite (Beautifil II, Shofu, Kyoto, Japan) while groups B and C consisted of a low viscosity flowable composite (Beautifil F03 Flow Plus). Groups D–F were formed by combining this flowable material (F03 Flow Plus) with low (5.2) aspect ratio fibers of different volume load fractions (20, 40 and 60%). Groups G, H and I combined F03 Flow Plus with mid (68) aspect ratio fibers of three different loads (5, 10 and 20%) while group J utilized high aspect ratio (640) fibers with 10% loading.
2.1
Fibers
Glass fibers with three different aspect ratios (ARs) were used ( Fig. 1 ). Low AR fibers presented as unsized milled E glass powder from Owens Corning, Ohio, USA. SEM analysis revealed an average length of 89 ± 62 μm (range 14–300, n = 400), an average diameter of 17 μm and an AR of 5.2 ± 3.65. They were used for three groups (D–F). High AR glass fibers presented as S glass ( S-2 Glass ) chopped strands from AGY, South Carolina, USA and consisted of numerous 10 μm diameter parallel filaments cut into short 6.4 mm lengths. These fibers have an AR of 640 without deviation and were used for group J. These S glass strands were also cut (average length 679, range 60–2400, n = 400) to create the third AR of 68 ± 43.5 used in 3 groups (G–I).
Both E glass and S glass fibers were used as the availability of very short cut or milled glass fibers is very limited. The prefix E and S stands for electrical grade and structural grade. Their physical properties are as follows: tensile strengths 3.4 GPa vs. 4.9 GPa, moduli 72 GPa vs. 87 GPa and densities of 2.58 g/cc vs. 2.46 g/cc respectively. Both fibers are predominantly aluminosilicate glasses. E glass is high in calcium while S glass is high in magnesium with no boron . Given the similarity in composition, physical properties and surface chemistry it was decided to use milled E glass as a low AR baseline. This material is readily available and is used by manufacturers of “packable” DRCs. The S glass fibers were used uncut in the higher AR sample group. The major difference between the two glasses was thus considered to be diameter and a sufficient spread of aspect ratios was achieved.
All fibers were first etched in a 4% potassium dichromate(IV)(K 2 Cr 2 O 7 )/89% sulphuric acid (H 2 SO 4 ) aqueous solution (Australian Scientific, NSW, Australia) for 4 h then thoroughly washed (until pH returned to 7) in deionized water followed by rinsing in ethanol and drying in an oven at 50 °C for 8 h. Fibers were then immersed in a hydrolyzed silane solution consisting of 2% 3-(Trimethyoxysilyl) propyl methacrylate silane (Z-6030 Sigma–Aldrich, MO, USA) in 93% ethanol and 5% deionized water. This solution was titrated to pH 5 with acetic acid. The fibers in solution were stirred for 2 min every 5 min for a total of 44 min before being rinsed several times in deionized water. In order to remove any physisorbed and some of the weakly chemisorbed layers the fibers were then conditioned in 100 °C deionized water for 10 min and then dried at 50 °C for 24 h .
2.2
Dental resin composite
The dental composite used in this study Beautifil is classified as a giomer and is composed of BisGMA/TEGDMA resin and pre-reacted glass-ionomer (S-PRG) fluoroaluminosilicate glass filler particles ranging in size from 0.01 to 4.0 (ave. 0.8) μm. Manufacturers of these materials claim fluoride release and recharge capabilities while maintaining superior physical properties to poly acid modified resin composites (compomers) .
This material is manufactured with five viscosities depending on filler ratio ranging from 68.6% volume (83.3% by weight) loaded “sculptable” Beautifil II (group A) to the very low viscosity Beautifil Flowable F10 (<47% by volume). Beautifil Flow Plus F03 (shade A2 lot number 111004) with 46.3% by volume (67.3% weight) filler loading and mid viscosity was chosen as the main control (group B) and also as the resin composite to be reinforced with glass fiber as it exhibited good strength at low viscosity.
2.3
Sample preparation
All samples were prepared according to ISO 4049. A split brass mold (2 mm × 2 mm × 25 mm) with glass top and base was used. The samples were cured using a Flash Max P3 460 (CMS Dental ApS, Copenhagen, Denmark) curing light according to manufacturer’s instruction (3 s) with six overlapping cures from both top and bottom slides. A standard light output meter (range 0–2000 mW) showed light output to be above 2 W. The packable control was dispensed onto a paper mixing pad before packing into the mold. The flowable composite was dispensed directly from the manufacturers syringe dispensing tip (internal Ø = 600 μm) into the split metal mold while all reinforced samples were mixed individually on the paper mixing pad with a metal spatula for 20 s prior to packing into the mold. To assess the effect of mixing on the samples another control group C was prepared by first dispensing the flowable resin composite F03 onto the pad and then spatulating for 10 s before loading into the mold.
The weight of fiber needed for each sample was found by using the densities of the two fibers used and also the DRC (1.80 g/cc). Density ( ρ ) equals weight in grams divided by volume in cubic centimeters. Therefore,
Volume = Weight Density
and since,
% V f = V f V f + V r
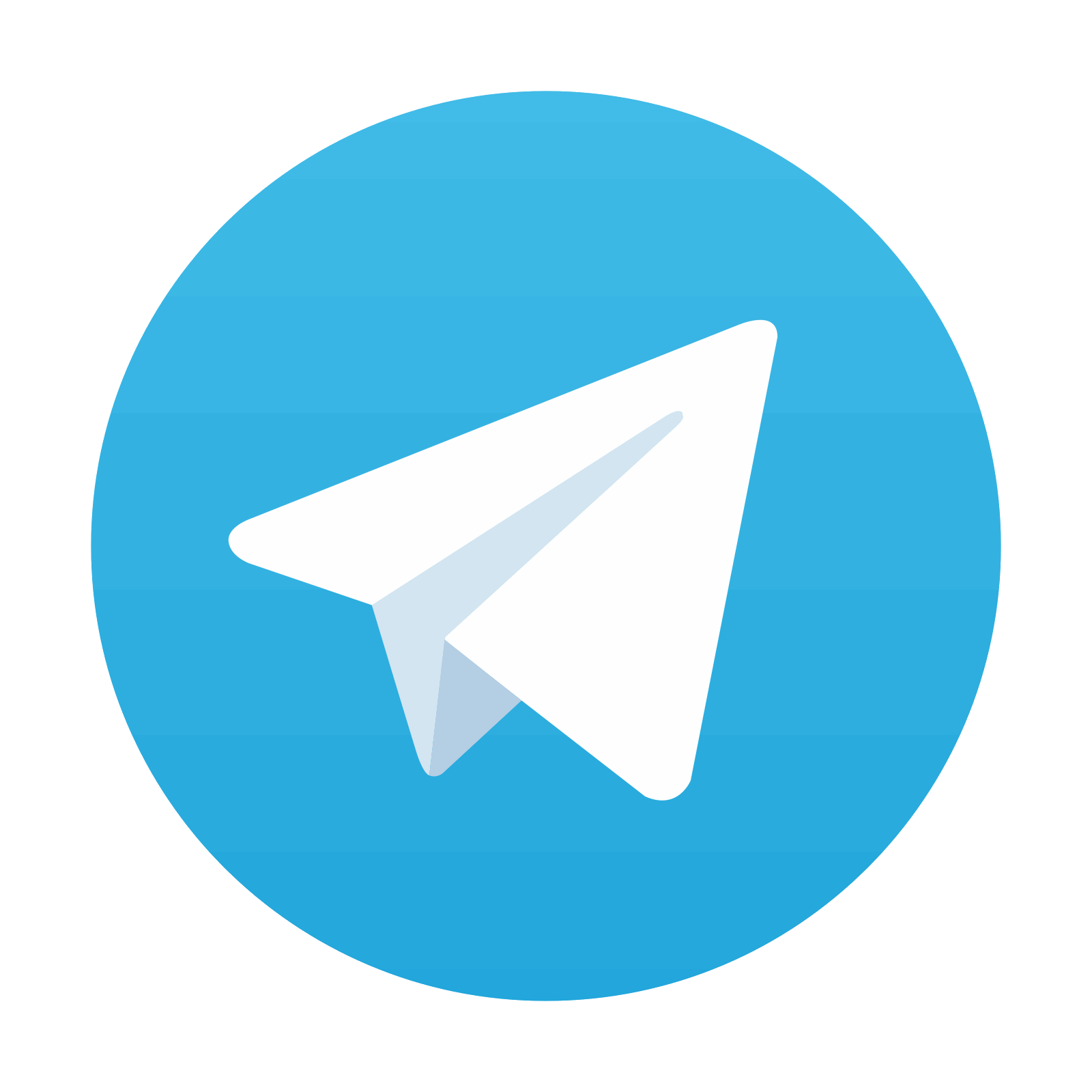
Stay updated, free dental videos. Join our Telegram channel

VIDEdental - Online dental courses
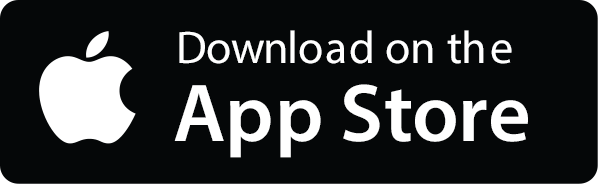
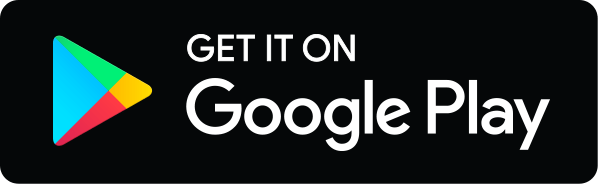