Abstract
Objective
The aim of the present study was to evaluate the effect of Electro Discharge Machining (EDM) on the corrosion resistance of two types of dental alloys used for fabrication of implant retained superstructures.
Methods
Two groups of specimens were prepared from a Co–Cr (Okta-C) and a grade II cpTi (Biotan) alloys respectively. Half of the specimens were subjected to EDM with Cu electrodes and the rest were conventionally finished (CF). The corrosion resistance of the alloys was evaluated by anodic polarization in Ringer’s solution. Morphological and elemental alterations before and after corrosion testing were studied by SEM/EDX. Six regions were analyzed on each surface before and after corrosion testing and the results were statistically analyzed by paired t -test ( a = 0.05).
Results
EDM demonstrated inferior corrosion resistance compared to CF surfaces, the latter being passive in a wider range of potential demonstrating higher polarization resistance and lower I corr values. Morphological alterations were found before and after corrosion testing for both materials tested after SEM analysis. EDX showed a significant decrease in Mo, Cr, Co, Cu (Co–Cr) and Ti, Cu (cpTi) after electrochemical testing plus an increase in C.
Significance
According to the results of this study the EDM procedure decreases the corrosion resistance of both the alloys tested, increasing thus the risk of possible adverse biological reactions.
1
Introduction
Electro Discharge Machining (EDM), known also as spark erosion, was introduced in dentistry to improve passive fitting of implant retained overdentures . Compared to other conventional corrective techniques (i.e. soldering, recasting, etc.), EDM provides the best fit to implant abutments and minimizes the stresses transferred , contributing thus to their clinical success .
EDM is a thermoelectric method, where a workpiece is immersed in a liquid (the dielectric fluid) comprising the cathode of an electrochemical cell, with Cu implant analogues serving as the anode. After cell charging, material is removed from the workpiece by melting and evaporation in single sparks . Surfaces subjected to EDM demonstrate important changes in morphology and composition . Despite that EDM is being used for more than two decades in dentistry, there is only one published study addressing these issues . According to the above study, EDM treated surfaces showed a rough texture with craters and pores, assigned to the collapse of plasma columns during the procedure. In addition, a recast layer of some microns in thickness has been observed on these surfaces, enriched with C and Cu from the decomposition of the dielectric fluid and the Cu electrodes respectively.
Considering that surface morphology and composition of dental alloys dominate their corrosion behavior, the changes induced by EDM may adversely affect the corrosion resistance of dental alloys. Although many studies have been performed on the corrosion behavior of dental alloys, no information exists, yet, on the electrochemical activity of dental alloys after EDM treatment.
The aim of this study was to evaluate the effect of EDM on the corrosion properties of two representative types of dental alloys. The testing hypothesis was that no statistically significant differences exist in the corrosion properties tested before and after EDM treatment.
2
Materials and methods
A completed cast model of an arch that received four dental implants was used for the preparation of four wax patterns, divided into two groups of two patterns each. The wax patterns of the first group were invested with a graphite-free conventional phosphate bonded silica-based investment material and cast in a centrifugal casting machine with a Co–Cr alloy ( Table 1 ). The wax patterns of the second group were invested with a magnesia-based investment material and cast with a grade II cpTi, in a two-chamber arc melting casting machine (Dor-A-Matic; Schütz Dental, Rosbach, Germany). Castings were sandblasted with 110 μm Al 2 O 3 at 3.0 atm pressure. One casting of each group was subjected to EDM procedure, whereas the second was conventionally ground and polished (conventional finishing, CF). For EDM treatment a system was used (EDM 2000, SAE Dental Products Inc, Bremerhaven, Germany), employing Cu electrodes, operated at 90 V voltage and 0.5–1.5 A current. During the final stage of the EDM procedure, the current was set at 0.5 A. In all stages the dielectric fluid used was kerosene.
Alloy | Type | Composition (wt%) a | Manufacturer |
---|---|---|---|
Okta-C | Co–Cr | Co: 61.1, Cr: 32.0, Mo: 5.5, Si: <1.0, Mn: <1.0 | SAE Dental Products Inc, Bremerhaven, Germany |
Biotan | cpTi grade II | Ti: balance, O: <0.2, N: <0.06, C: <0.08, H: <0.013, Fe: <0.25 | Schütz Dental, Rosbach, Germany |
All the castings were cut in separate crown analogues and embedded in an epoxy resin so that the only exposed surface was the circular cross section. The corrosion resistance of the specimens was evaluated by linear sweep voltammetry, and cyclic voltammetry using a mini cell system setup (MCS). The choice of the MCS was made due to the small dimensions of the samples and the need to test the material without any further preparation, to better simulate the clinical conditions . Each specimen was subjected to three measurements for verification purposes using Ringer’s solution (9 g NaCl, 0.24 g CaCl 2 ·6H 2 O, 0.43 g KCl, 0.2 g NaHCO 3 in 1000 ml distilled water). The system was connected to a potentiostat. A saturated calomel electrode (SCE, E o = 241 mV vs standard hydrogen electrode—SHE) was used as a reference electrode. The scan rate was set at 10 mV/s, the potential ranged between −1 V and +1.5 V (vs SHE) and the cross-sectional area was 0.008 cm 2 . All measurements were performed at room temperature. The data were collected and analyzed by using the Corrware and Corrview software respectively (Scribner Associates Inc., Southern Pines, NC, USA). From the current density–potential curves, the following electrochemical parameters were determined: polarization resistance ( R p ), exchange current density ( I o ) and corrosion potential ( E corr ). In order to create a mean curve for each material, the experimental curves were treated by the Origin software (Microcal Software Inc, Northampton, MA, USA).
The morphology and elemental composition of CF and EDM treated surfaces of all specimens, before (reference) and after corrosion testing, was studied under a SEM (Quanta 200, FEI, Hillsboro, OR, USA) equipped with an energy-dispersive X-ray spectrometer (Sapphire CDU, EDAX Int, Mahwah, NJ, USA). The morphological features were examined by recording secondary electron (SE) images under high vacuum (10 −6 mbar), at 20 kV accelerating voltage, 90 μA beam current and 1000× original magnification on non-coated specimens. For the elemental composition, backscattered electron (BE) images were acquired, employing a solid-state detector (SSD), to identify regions with different mean atomic numbers. These regions were then examined by energy-dispersive X-ray microanalysis (EDX), using a liquid N 2 -cooled Si(Li) detector with a super-ultrathin Be window (SUTW+). Spectra were collected from six regions per surface employing area scan mode (130 μm × 130 μm sampling area) under 20 kV accelerating voltage, 110 μA beam current, 1000× magnification, 500 s acquisition time and 30–34% detector dead-time. In addition, spot analysis measurements (one per region) were carried out on high and low mean atomic number phases, to identify the regional composition. The elemental quantitative analysis was performed by Genesis software (v 5.2, EDAX Int.) under a nonstandard mode, using ZAF correction methods. Multi-element X-ray mapping was performed to identify the elemental distribution on specimens’ surfaces. The results of the quantitative analysis were statistically analyzed by Student’s t -test ( α = 0.05).
2
Materials and methods
A completed cast model of an arch that received four dental implants was used for the preparation of four wax patterns, divided into two groups of two patterns each. The wax patterns of the first group were invested with a graphite-free conventional phosphate bonded silica-based investment material and cast in a centrifugal casting machine with a Co–Cr alloy ( Table 1 ). The wax patterns of the second group were invested with a magnesia-based investment material and cast with a grade II cpTi, in a two-chamber arc melting casting machine (Dor-A-Matic; Schütz Dental, Rosbach, Germany). Castings were sandblasted with 110 μm Al 2 O 3 at 3.0 atm pressure. One casting of each group was subjected to EDM procedure, whereas the second was conventionally ground and polished (conventional finishing, CF). For EDM treatment a system was used (EDM 2000, SAE Dental Products Inc, Bremerhaven, Germany), employing Cu electrodes, operated at 90 V voltage and 0.5–1.5 A current. During the final stage of the EDM procedure, the current was set at 0.5 A. In all stages the dielectric fluid used was kerosene.
Alloy | Type | Composition (wt%) a | Manufacturer |
---|---|---|---|
Okta-C | Co–Cr | Co: 61.1, Cr: 32.0, Mo: 5.5, Si: <1.0, Mn: <1.0 | SAE Dental Products Inc, Bremerhaven, Germany |
Biotan | cpTi grade II | Ti: balance, O: <0.2, N: <0.06, C: <0.08, H: <0.013, Fe: <0.25 | Schütz Dental, Rosbach, Germany |
All the castings were cut in separate crown analogues and embedded in an epoxy resin so that the only exposed surface was the circular cross section. The corrosion resistance of the specimens was evaluated by linear sweep voltammetry, and cyclic voltammetry using a mini cell system setup (MCS). The choice of the MCS was made due to the small dimensions of the samples and the need to test the material without any further preparation, to better simulate the clinical conditions . Each specimen was subjected to three measurements for verification purposes using Ringer’s solution (9 g NaCl, 0.24 g CaCl 2 ·6H 2 O, 0.43 g KCl, 0.2 g NaHCO 3 in 1000 ml distilled water). The system was connected to a potentiostat. A saturated calomel electrode (SCE, E o = 241 mV vs standard hydrogen electrode—SHE) was used as a reference electrode. The scan rate was set at 10 mV/s, the potential ranged between −1 V and +1.5 V (vs SHE) and the cross-sectional area was 0.008 cm 2 . All measurements were performed at room temperature. The data were collected and analyzed by using the Corrware and Corrview software respectively (Scribner Associates Inc., Southern Pines, NC, USA). From the current density–potential curves, the following electrochemical parameters were determined: polarization resistance ( R p ), exchange current density ( I o ) and corrosion potential ( E corr ). In order to create a mean curve for each material, the experimental curves were treated by the Origin software (Microcal Software Inc, Northampton, MA, USA).
The morphology and elemental composition of CF and EDM treated surfaces of all specimens, before (reference) and after corrosion testing, was studied under a SEM (Quanta 200, FEI, Hillsboro, OR, USA) equipped with an energy-dispersive X-ray spectrometer (Sapphire CDU, EDAX Int, Mahwah, NJ, USA). The morphological features were examined by recording secondary electron (SE) images under high vacuum (10 −6 mbar), at 20 kV accelerating voltage, 90 μA beam current and 1000× original magnification on non-coated specimens. For the elemental composition, backscattered electron (BE) images were acquired, employing a solid-state detector (SSD), to identify regions with different mean atomic numbers. These regions were then examined by energy-dispersive X-ray microanalysis (EDX), using a liquid N 2 -cooled Si(Li) detector with a super-ultrathin Be window (SUTW+). Spectra were collected from six regions per surface employing area scan mode (130 μm × 130 μm sampling area) under 20 kV accelerating voltage, 110 μA beam current, 1000× magnification, 500 s acquisition time and 30–34% detector dead-time. In addition, spot analysis measurements (one per region) were carried out on high and low mean atomic number phases, to identify the regional composition. The elemental quantitative analysis was performed by Genesis software (v 5.2, EDAX Int.) under a nonstandard mode, using ZAF correction methods. Multi-element X-ray mapping was performed to identify the elemental distribution on specimens’ surfaces. The results of the quantitative analysis were statistically analyzed by Student’s t -test ( α = 0.05).
3
Results
Mean polarization curves obtained from the alloys tested are presented in Figs. 1 and 2 . Independently from the alloy type, comparable shapes of the I – E curves were observed, although each alloy demonstrated a unique profile. Fig. 1 shows the mean cyclic voltammetry curves for the Co–Cr alloy. Conventionally finished (CF) specimens were passive in a wider potential range, than the ones after EDM. The latter showed a shift of corrosion potential values to an anodic direction in comparison to CF ( E oEDM : −0.30 V, E oCF : −0.56 V), and a passivity breakdown limit at 0.50 V to an anodic direction. Fig. 2 presents the mean cyclic voltammetry curves for the cpTi alloy. A shift of the corrosion potential after the EDM procedure ( E oEDM : −0.30 V, E oCF : −0.55 V) is observed, as well. The shape of the I vs the E curve for CF treated alloys corresponds to a typical passive behavior, but after EDM it changes to active, demonstrating an oxidation current to an anodic direction. Table 2 shows the electrochemical parameters calculated from the polarization curves. The EDM treated alloys showed a higher current density.
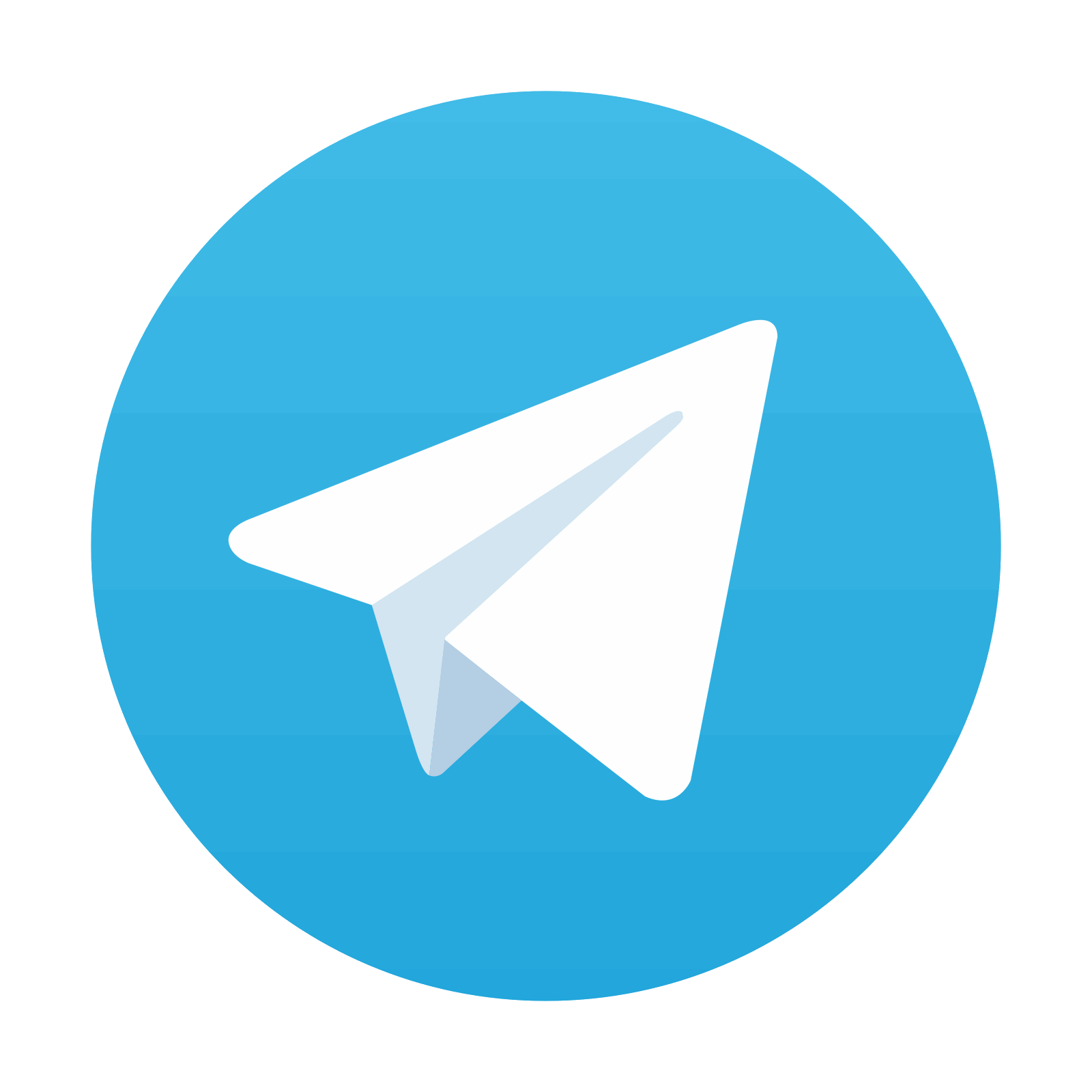
Stay updated, free dental videos. Join our Telegram channel

VIDEdental - Online dental courses
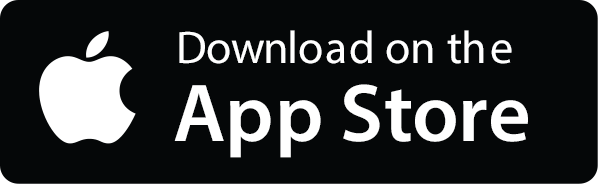
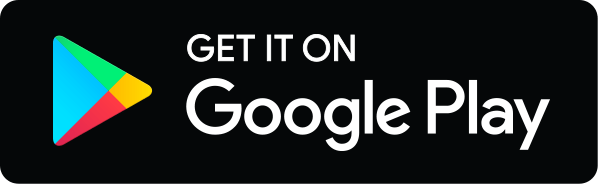
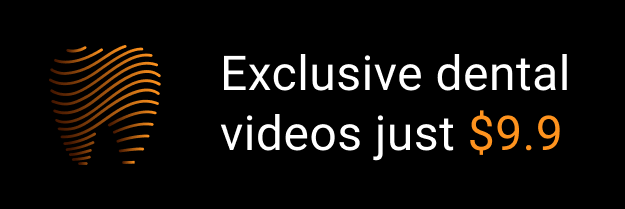