Graphical abstract

Abstract
Objective
To evaluate the effect of load-cycle aging and/or 6 months artificial saliva (AS) storage on bond durability and interfacial ultramorphology of resin-modified glass ionomer cement (RMGIC) applied onto dentine air-abraded using Bioglass 45S5 (BAG) with/without polyacrylic acid (PAA) conditioning.
Methods
RMGIC (Ionolux, VOCO) was applied onto human dentine specimens prepared with silicon-carbide abrasive paper or air-abraded with BAG with or without the use of PAA conditioning. Half of bonded-teeth were submitted to load cycling (150,000 cycles) and half immersed in deionised water for 24 h. They were cut into matchsticks and submitted immediately to microtensile bond strength (μTBS) testing or 6 months in AS immersion and subsequently μTBS tested. Results were analysed statistically by two-way ANOVA and Student–Newman–Keuls test (α = 0.05). Fractographic analysis was performed using FE-SEM, while further RMGIC-bonded specimens were surveyed for interfacial ultramorphology characterisation (dye-assisted nanoleakage) using confocal microscopy.
Results
RMGIC applied onto dentine air-abraded with BAG regardless PAA showed no significant μTBS reduction after 6 months of AS storage and/or load cycling (p > 0.05). RMGIC–dentine interface showed no sign of degradation/nanoleakage after both aging regimens. Conversely, interfaces created in PAA-conditioned SiC-abraded specimens showed significant reduction in μTBS (p < 0.05) after 6 months of storage and/or load cycling with evident porosities within bonding interface.
Conclusions
Dentine pre-treatment using BAG air-abrasion might be a suitable strategy to enhance the bonding performance and durability of RMGIC applied to dentine. The use of PAA conditioner in smear layer-covered dentine may increase the risk of degradation at the bonding interface.
Clinical significance
A combined dentine pre-treatment using bioglass followed by PAA may increase the bond strength and maintain it stable over time. Conversely, the use of PAA conditioning alone may offer no significant contribute to the immediate and prolonged bonding performance.
1
Introduction
Conventional rotary instruments equipped with tungsten-carbide, carbon-steel or diamond burs are used routinely in clinical practice for dental cavity preparation. In minimally invasive dentistry (MID), the underlying tenet is to preserve sound dental hard tissues and minimise the unnecessary alteration of healthy tooth structure [ ]. Air-abrasion has been advocated to be a suitable approach to reduce the risk for unnecessary removal of sound dental tissues [ ], although the choice of powders used may affect the quality and durability of the tooth-restoration interface [ ]. Bioglass 45S5 (BAG), a calcium/sodium phosphate-phyllosilicate glass, is used in air-abrasion with several advantages including the absence of pain during the operative procedure and the opportunity to leave cavities with rounded internal line angles, thus minimising the contraction stress of resin composites [ ]. Moreover, BAG will embed into the dentine surface so creating a bioactive smear layer [ ] that can react with body fluids, encouraging mineral deposition through formation of hydroxyapatite [Ca 10 (PO 4 ) 6 (OH) 2 ] [ ].
The stabilisation of the interface between tooth and restorative material, as well as the creation of in loco conditions that might protect and/or repair the retained demineralised dental hard tissues are of particular importance in MID [ ]. The use of fluoride-releasing restorative materials such as glass ionomer cements (GIC) or resin-modified glass ionomer cements (RMGIC) may contribute to interfacial protection because of their buffering ability and their fluoride ion release/re-charge [ ]. Moreover, since GIC-based materials have the aptitude to induce crystal growth [ ] within the interface of the restoration after long-term storage in water, with a chemical composition similar to that of dental hard tissues [ ], it is hypothesised that the combination of dentine pre-treatment with BAG air-abrasion and subsequent restoration using GIC-based materials could be a suitable strategy to achieve longer-lasting bonding interfaces that can resist degradation over time.
RMGICs combine the therapeutic properties of GICs with the mechanical properties of resin polymers [ ]. The setting process of RMGIC is based on free-radical polymerisation as well as the acid–base reaction between polyalkenoic acids and fluoroaluminosilicate glass [ ]. The self-adhesive mechanism of GIC-based materials to dentine is the micromechanical interlocking achieved by shallow hybridisation of the micro-porous collagen network. There is a chemical reaction that occurs through the formation of ionic bonds between the carboxyl groups of the polyalkenoic acids and calcium of hydroxyapatite-coated collagen fibrils [ ]. Polyacrylic acid (PAA) is the most common conditioner used in enamel/dentine pre-treatment to remove the smear layer prior to the application of GIC-based restorative materials onto dentine and enamel. However, concerns exist regarding its use, application times and concentrations as these factors may interfere with the overall bonding performance. Indeed, a high number of adhesive failures between a RMGIC and resin composite have been reported when a polyalkenoic conditioner was used on smear-layer covered dentine [ ].
Milly et al., [ ] used a combination of PAA-BAG powder in air-abrasion as a pre-treatment of white spot lesions in enamel (WSL). It was showed that surface pre-conditioning was able to enhance the remineralisation of WSL. Indeed, there were reported increased mineral content, improved mechanical properties and alterations in the enamel ultrastructure. To the present authors’ knowledge, there are no previous published studies assessing the effect of dentine pre-treatments using BAG air-abrasion and/or by PAA conditioning on the interfacial characteristics of resin-modified glass ionomer cements submitted to different aging regimes.
The aim of this study was to test the microtensile bond strength (MTBS), after load-cycle aging and/or 6 months storage in artificial saliva (AS), of RMGIC applied to dentine air-abraded using Bioglass 45S5 (BAG) with or without subsequent surface pre-conditioning using 10% polyacrylic acid (PAA). Fractographic analysis and interfacial dye-assisted nanoleakage assessment of the bonded interfaces were evaluated using field-emission scanning electron microscopy (FE-SEM) and confocal laser-scanning microscopy (CLSM), respectively. The tested null hypotheses were that the durability of RMGIC applied with or without the use of PAA conditioner onto a BAG air-abraded dentine surface would not be affected by: (i) 6-month aging in artificial saliva (AS); (ii) load-cycle aging only; (iii) load cycling followed by 6-month AS aging.
2
Material and methods
2.1
Preparation of dentine specimens
Sound human molars (from 20- to 40-yr-old subjects) extracted for periodontal or orthodontic reasons under a protocol approved by an Institutional Review Board (n°CEI16/020), were used in this study. The teeth were stored in deionised water at 4 °C for no longer than 1 month. The roots were removed 1 mm beneath the cemento–enamel junction (CEJ) using a diamond-embedded blade (high concentration XL 12205; Benetec, London, UK) mounted on a hard-tissue microtome (Remet evolution, REMET, Casalecchio di Reno, Italy). A subsequent parallel cut was performed to remove the occlusal enamel and expose mid-coronal dentine. This flat dentine surface was polished with silicon-carbide paper (SiC #320-grit) for 1 min under continuous water irrigation to simulate the creation of a smear layer that would be created clinically after rotary dentine preparation. The specimens were divided into experimental groups and subgroups as shown in Table 1 .
Subgroups: [number of specimens for MTBS/Confocal/FE-SEM] | |||||
---|---|---|---|---|---|
Main groups [8 teeth each] | Dentine etching (10% PAA gel)* | CTR: 24 h AS | LC: Load cycling in AS | AS: 6-month in AS | AS + LC: Load cycling + 6-month in AS |
SiC paper | NO | [8/8/5] | [8/8/5] | [8/8/5] | [8/8/5] |
SiC paper | YES | [8/8/5] | [8/8/5] | [8/8/5] | [8/8/5] |
Air-abrasion BAG | NO | [8/8/5] | [8/8/5] | [8/8/5] | [8/8/5] |
Air-abrasion BAG | YES | [8/8/5] | [8/8/5] | [8/8/5] | [8/8/5] |
2.2
Experimental design: dentine pre-treatments and aging protocols
The experimental design required that half of the dentine specimens were air-abraded with BAG (Sylc, VELOPEX International, London, UK) under water irrigation. An Aquacare air-abrasion unit (VELOPEX International) was used with an air pressure of 4 bar (400 MPa) for 1 min at a distance of 1 cm from the dentine surface and with continuous mesio-distal and bucco-lingual movements. Subsequently, the air-abraded dentine surface were conditioned with 10% PAA gel (GC Fuji conditioner, Newport Pagnell, UK) for 20 s and rinsed with water for 20 s, or left unconditioned.
Overall, four primary groups (n = 32 specimens/group) were created for this experimental design based on the preparation of the dentine substrate:
Group 1. Specimens abraded using 320-grit SiC abrasive paper (1 min) under continuous irrigation, followed by a water rinse (20 s), air-drying (2 s) and restored with a light-cured RMGIC (no PAA conditioning).
Group 2. Specimens abraded with 320-grit SiC abrasive paper (1 min), conditioned with 10% PAA gel for 20 s rinsed with water (20 s), dried, and restored with a light-cured RMGIC (PAA conditioning).
Group 3. Specimens abraded using 320-grit SiC abrasive paper (1 min) under continuous irrigation and then air-abraded with BAG particles under a continuous water shroud (1 min), rinsed with water (20 s), dried, and restored with a light-cured RMGIC (BAG-no conditioning).
Group 4. Specimens abraded using 320-grit SiC abrasive paper (1 min) under continuous irrigation, air-abraded with BAG particles under a continuous water shroud (1 min), rinsed with water (20 s), conditioned with 10% PAA (20 s), rinsed with water (20 s), dried, and restored with a light-cured RMGIC (BAG-PAA conditioning).
The restorative procedure was performed by applying the content of two mono-dose capsules of a commercial RMGIC (Ionolux; Voco GmbH, Cuxhaven, Germany), mixed for 10 s in a trituration unit and applied in bulk on to the dentine surface and light-cured for 30 s with a light-curing unit (Radii plus, SID Ltd, Bayswater VIC, Australia) with a LED light source ( > 1000 mW/cm 2 ).
Each main group was subsequently subdivided in four sub-groups (n = 8 specimens) based on the aging protocol: 1) CRT: no aging (control, 24 h in deionised water); 2) LC: Load cycling (150,000 cycles in artificial saliva); 3) AS: prolonged water storage (6 months in artificial saliva); 4) LC + AS: Load cycling (150,000 cycles) + AS storage (6 months).
The composition of the artificial saliva was (AS: 0.103 g l −1 of CaCl 2 , 0.019 g l −1 of MgCl 2 ·6H 2 O, 0.544 g l −1 of KH 2 PO 4 , 30 g l −1 of KCl, and 4.77 g l −1 HEPES (acid) buffer, pH 7.4] at 37 °C for 24 h or 6 months) [ ]. The subgroup specimens LC and LC + AS were mounted in plastic rings with dental stone for load cycle testing (150,000 cycles; 3 Hz; 70 N). A compressive load was applied to the flat surface of the RMGIC using a 5-mm diameter spherical stainless steel plunger attached to a cyclic loading machine (model S-MMT-250NB; Shimadzu, Tokyo, Japan) while immersed in AS [ ].
2.3
Micro-tensile bond strength (MTBS) and fracture analysis (FE-SEM)
The specimens were sectioned using a hard-tissue microtome (Remet evolution, REMET) in both X and Y planes across the dentine-RMGIC interface, obtaining approx. 20 matchstick-shaped specimens from each tooth with cross-sectional areas of 0.9 mm 2 . These were stored in AS for 24 h or 6 months and then tested for their MTBS. The latter was performed using a microtensile bond strength device with a stroke length of 50 mm, peak force of 500 N and a displacement resolution of 0.5 mm. Modes of failure were classified as a percentage of adhesive (A), mixed (M) or cohesive (C) failures when the failed interfaces were examined at 30× magnification by stereoscopic microscopy. Five representative fractured specimens from each sub-group were critical-point dried and mounted on aluminium stubs with carbon cement. The specimens were gold-sputter-coated and imaged using field-emission scanning electron microscopy (FE-SEM S-4100; Hitachi, Wokingham, UK) at 10 kV and a working distance of 15 mm.
The normality of MTBS data was evaluated using Shapiro-Wilk test (p > .05). Homogeneity of variance was calculated using the Brown-Forsythe test. For all tests, the variances were homoscedastic (p > .05). Data were analysed statistically by two-way ANOVA including interactions between factors, using MTBS as a dependent variable. Dentine surface treatment and aging method were considered as independent variables. Post-hoc multiple comparisons were performed using the Student–Newman–Keuls test. Statistical significance was set at α = 0.05.
2.4
Ultramorphology of the bonded-dentine interfaces – confocal microscopy evaluation
One dentine-bonded slab sample (Ø 0.9 mm 2 ) was selected from each experimental sub-group (n = 8) during the cutting procedures of match-sticks. These were coated with a fast-setting nail varnish, applied 1 mm from the bonded interface. They were immersed in a Rhodamine B (Sigma Chemicals) water solution (0.1 wt%) for 24 h. Subsequently, the specimens were ultrasonicated with distilled water for 5 min and then polished for 30 s per side with a 2400-grit SiC paper. The specimens were finally ultrasonicated again with distilled water for 5 min and submitted for confocal microscopy analysis. Using a confocal scanning microscope (Olympus FV1000, Olympus Corp., Tokyo, Japan), equipped with a 63X/1.4 NA oil-immersion lens and a 543 nm LED illumination, reflection and fluorescence images were obtained with a 1-μm z-step to section optically the specimens to a depth of up to 20 μm below the surface [ ]. The z-axis scan of the interface surface was pseudo-coloured arbitrarily for improved visualisation and compiled into both single and topographic projections using the CLSM image-processing software (Fluoview Viewer, Olympus). The configuration of the system was standardised and used at constant settings for the entire investigation. Each dentine interface was investigated completely and then five optical images were randomly captured. Micrographs representing the most common morphological features observed along the bonded interfaces were captured and recorded [ ].
2
Material and methods
2.1
Preparation of dentine specimens
Sound human molars (from 20- to 40-yr-old subjects) extracted for periodontal or orthodontic reasons under a protocol approved by an Institutional Review Board (n°CEI16/020), were used in this study. The teeth were stored in deionised water at 4 °C for no longer than 1 month. The roots were removed 1 mm beneath the cemento–enamel junction (CEJ) using a diamond-embedded blade (high concentration XL 12205; Benetec, London, UK) mounted on a hard-tissue microtome (Remet evolution, REMET, Casalecchio di Reno, Italy). A subsequent parallel cut was performed to remove the occlusal enamel and expose mid-coronal dentine. This flat dentine surface was polished with silicon-carbide paper (SiC #320-grit) for 1 min under continuous water irrigation to simulate the creation of a smear layer that would be created clinically after rotary dentine preparation. The specimens were divided into experimental groups and subgroups as shown in Table 1 .
Subgroups: [number of specimens for MTBS/Confocal/FE-SEM] | |||||
---|---|---|---|---|---|
Main groups [8 teeth each] | Dentine etching (10% PAA gel)* | CTR: 24 h AS | LC: Load cycling in AS | AS: 6-month in AS | AS + LC: Load cycling + 6-month in AS |
SiC paper | NO | [8/8/5] | [8/8/5] | [8/8/5] | [8/8/5] |
SiC paper | YES | [8/8/5] | [8/8/5] | [8/8/5] | [8/8/5] |
Air-abrasion BAG | NO | [8/8/5] | [8/8/5] | [8/8/5] | [8/8/5] |
Air-abrasion BAG | YES | [8/8/5] | [8/8/5] | [8/8/5] | [8/8/5] |
2.2
Experimental design: dentine pre-treatments and aging protocols
The experimental design required that half of the dentine specimens were air-abraded with BAG (Sylc, VELOPEX International, London, UK) under water irrigation. An Aquacare air-abrasion unit (VELOPEX International) was used with an air pressure of 4 bar (400 MPa) for 1 min at a distance of 1 cm from the dentine surface and with continuous mesio-distal and bucco-lingual movements. Subsequently, the air-abraded dentine surface were conditioned with 10% PAA gel (GC Fuji conditioner, Newport Pagnell, UK) for 20 s and rinsed with water for 20 s, or left unconditioned.
Overall, four primary groups (n = 32 specimens/group) were created for this experimental design based on the preparation of the dentine substrate:
Group 1. Specimens abraded using 320-grit SiC abrasive paper (1 min) under continuous irrigation, followed by a water rinse (20 s), air-drying (2 s) and restored with a light-cured RMGIC (no PAA conditioning).
Group 2. Specimens abraded with 320-grit SiC abrasive paper (1 min), conditioned with 10% PAA gel for 20 s rinsed with water (20 s), dried, and restored with a light-cured RMGIC (PAA conditioning).
Group 3. Specimens abraded using 320-grit SiC abrasive paper (1 min) under continuous irrigation and then air-abraded with BAG particles under a continuous water shroud (1 min), rinsed with water (20 s), dried, and restored with a light-cured RMGIC (BAG-no conditioning).
Group 4. Specimens abraded using 320-grit SiC abrasive paper (1 min) under continuous irrigation, air-abraded with BAG particles under a continuous water shroud (1 min), rinsed with water (20 s), conditioned with 10% PAA (20 s), rinsed with water (20 s), dried, and restored with a light-cured RMGIC (BAG-PAA conditioning).
The restorative procedure was performed by applying the content of two mono-dose capsules of a commercial RMGIC (Ionolux; Voco GmbH, Cuxhaven, Germany), mixed for 10 s in a trituration unit and applied in bulk on to the dentine surface and light-cured for 30 s with a light-curing unit (Radii plus, SID Ltd, Bayswater VIC, Australia) with a LED light source ( > 1000 mW/cm 2 ).
Each main group was subsequently subdivided in four sub-groups (n = 8 specimens) based on the aging protocol: 1) CRT: no aging (control, 24 h in deionised water); 2) LC: Load cycling (150,000 cycles in artificial saliva); 3) AS: prolonged water storage (6 months in artificial saliva); 4) LC + AS: Load cycling (150,000 cycles) + AS storage (6 months).
The composition of the artificial saliva was (AS: 0.103 g l −1 of CaCl 2 , 0.019 g l −1 of MgCl 2 ·6H 2 O, 0.544 g l −1 of KH 2 PO 4 , 30 g l −1 of KCl, and 4.77 g l −1 HEPES (acid) buffer, pH 7.4] at 37 °C for 24 h or 6 months) [ ]. The subgroup specimens LC and LC + AS were mounted in plastic rings with dental stone for load cycle testing (150,000 cycles; 3 Hz; 70 N). A compressive load was applied to the flat surface of the RMGIC using a 5-mm diameter spherical stainless steel plunger attached to a cyclic loading machine (model S-MMT-250NB; Shimadzu, Tokyo, Japan) while immersed in AS [ ].
2.3
Micro-tensile bond strength (MTBS) and fracture analysis (FE-SEM)
The specimens were sectioned using a hard-tissue microtome (Remet evolution, REMET) in both X and Y planes across the dentine-RMGIC interface, obtaining approx. 20 matchstick-shaped specimens from each tooth with cross-sectional areas of 0.9 mm 2 . These were stored in AS for 24 h or 6 months and then tested for their MTBS. The latter was performed using a microtensile bond strength device with a stroke length of 50 mm, peak force of 500 N and a displacement resolution of 0.5 mm. Modes of failure were classified as a percentage of adhesive (A), mixed (M) or cohesive (C) failures when the failed interfaces were examined at 30× magnification by stereoscopic microscopy. Five representative fractured specimens from each sub-group were critical-point dried and mounted on aluminium stubs with carbon cement. The specimens were gold-sputter-coated and imaged using field-emission scanning electron microscopy (FE-SEM S-4100; Hitachi, Wokingham, UK) at 10 kV and a working distance of 15 mm.
The normality of MTBS data was evaluated using Shapiro-Wilk test (p > .05). Homogeneity of variance was calculated using the Brown-Forsythe test. For all tests, the variances were homoscedastic (p > .05). Data were analysed statistically by two-way ANOVA including interactions between factors, using MTBS as a dependent variable. Dentine surface treatment and aging method were considered as independent variables. Post-hoc multiple comparisons were performed using the Student–Newman–Keuls test. Statistical significance was set at α = 0.05.
2.4
Ultramorphology of the bonded-dentine interfaces – confocal microscopy evaluation
One dentine-bonded slab sample (Ø 0.9 mm 2 ) was selected from each experimental sub-group (n = 8) during the cutting procedures of match-sticks. These were coated with a fast-setting nail varnish, applied 1 mm from the bonded interface. They were immersed in a Rhodamine B (Sigma Chemicals) water solution (0.1 wt%) for 24 h. Subsequently, the specimens were ultrasonicated with distilled water for 5 min and then polished for 30 s per side with a 2400-grit SiC paper. The specimens were finally ultrasonicated again with distilled water for 5 min and submitted for confocal microscopy analysis. Using a confocal scanning microscope (Olympus FV1000, Olympus Corp., Tokyo, Japan), equipped with a 63X/1.4 NA oil-immersion lens and a 543 nm LED illumination, reflection and fluorescence images were obtained with a 1-μm z-step to section optically the specimens to a depth of up to 20 μm below the surface [ ]. The z-axis scan of the interface surface was pseudo-coloured arbitrarily for improved visualisation and compiled into both single and topographic projections using the CLSM image-processing software (Fluoview Viewer, Olympus). The configuration of the system was standardised and used at constant settings for the entire investigation. Each dentine interface was investigated completely and then five optical images were randomly captured. Micrographs representing the most common morphological features observed along the bonded interfaces were captured and recorded [ ].
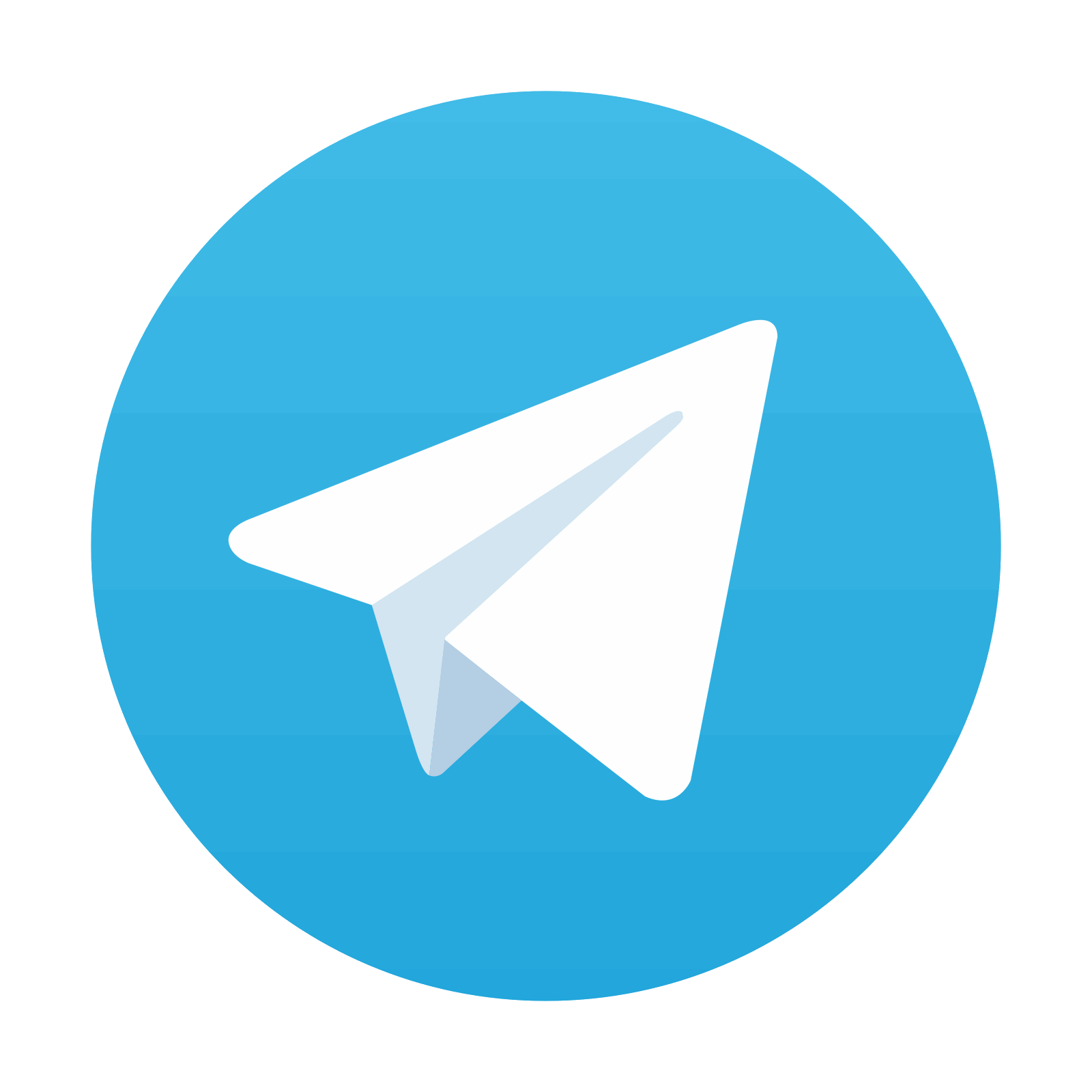
Stay updated, free dental videos. Join our Telegram channel

VIDEdental - Online dental courses
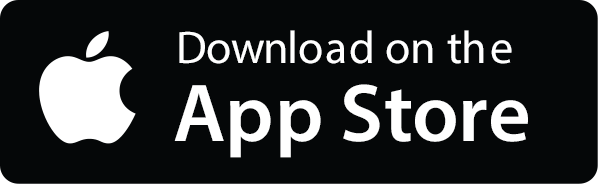
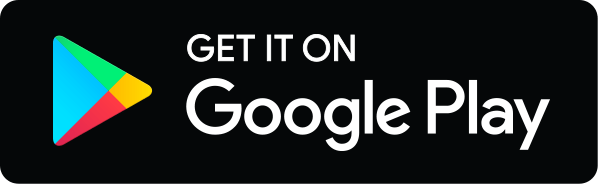