The purpose of this article is to synthesize different technologies that are available for the creation of a virtual patient, “the digital clone” because the data can be used for diagnosis as well as treatment planning. The role of facial scans, 3-dimensional intraoral scans as well as the cone beam computed tomography in the creation of a digital clone is discussed in detail. A step-by-step guide is created for the reader for integration of the intraoral scan data with the cone beam computed tomography Dicom data to create a digital clone.
Key points
- •
With the improvement of optical scanning technology, the study of facial morphology using 3-dimensional face scanners has gained importance and reached a new level.
- •
Intraoral scanners are typically connected to mobile carts as a complete unit with the computer and monitor. More recent versions of scanners are connected wireless to laptop computers.
- •
The typical printable file format in 3-dimensional imaging is in standard triangulation language and the images are standard triangulation language image files.
- •
Integrating cone beam computed tomography data with digital impressions and digital wax-ups with the prosthetic plan is the gold standard for prosthetically driven implantology and interdisciplinary treatment planning.
Facial scanners and their role in the creation of a virtual dental patient
The recognition of the facial morphology is necessary for the preoperative, postoperative evaluation, and symmetry assessment. It has significant applications in dentistry. Facial morphology and analysis are very critical in various disciplines of dentistry such as craniofacial surgery, maxillofacial surgery, orthodontics, craniofacial orthodontics, prosthodontics, and forensics. Facial scan analysis is presently a new highlight area of research. Conventional strategies for assessing facial morphology were based entirely on 2-dimensional (2D) methods. , In the past, using 2D imaging and photographs, mathematical instruments were used to obtain the facial analysis data.
With the improvement of optical scanning technology, the study of facial morphology using 3-dimensional (3D) face scanners has gained traction and reached to a new level from 2D to 3D. , One such example is shown in Fig. 1 , where a facial scan is displayed via a design software(InLab by Dentsply-Sirona).

A facial scanner is a noninvasive measuring tool that can acquire 3D facial models in an open data format with real skin texture color and a scanning process that is typically very short. The literature increasingly reported that 3D facial scanners could be used in the dental clinics, with the 3D facial samples acquired by scanners being used for 3D quantitative diagnostics and treatment assessment.
With the advancements in technology, the volumetric data obtained from the CBCT scans, intraoral scans may be superimposed to create a 3D virtual patient. This virtual patient can help in preoperative procedures, treatment planning, and patient outcomes. The data points such as facial landmarks can be obtained using the 3D face scanning using the scanner.
The advancements in technology have not only improved the speed and accuracy of the treatment but also has enhanced outcomes in the facial esthetics, facial profile and in creating the digital smile design. Greater accuracy improves the quality of the data recorded from the face scanner, which ultimately improves the outcome.
There are 2 fundamental types of facial scanners: laser scanners and white light scanners. Although the equipment is different, both types of scanning systems use the principle of triangulation to produce a 3D image of the face. Laser scanners project multiple dots or lines on the patient’s face, forming a triangle between the laser (typically 200 FPS infrared class I Laser compliant), light sensor (usually a camera), and the patient. The laser pattern moves over the face to scan the surface area and create a 3D image. The distance of the different surface levels on the patient’s face is determined by triangulation. In contrast, white light scanners project light patterns onto the face, forming a triangle between the projector, light sensor (ie, camera), and the patient. In addition, there are different types of scanning systems including fixed and moveable systems. Fixed scanners, such as the OBI Scanner (Viale Lombardia 6, 20133 Milano, Italy) are supported by a tripod placed directly in front of the seated patient. The patient is prompted to make specific head movements (ie, right, left, upward, downward) by the software. Moveable scanners, such as the Artec Evan Scan, are portable and moved around the face to acquire a 3D scan.
Three-dimensional scans are easily obtained using a smartphone with a dedicated application and the corresponding software. Face scans use fast, noncontact scanning and are generally well-tolerated by the patient. Laser stripe is safe for the human eye and unperceived by the patient except when it passes directly over the pupils. However, some face scanners flash a series of bright lights to capture the 3D image, which could cause slight discomfort for some patients. To ensure a constant state of facial expression, the patient is directed to close his or her eyes during the light flashes. Each scanning system has a different range that the patient must stay within for the duration of the scanning process. To ensure that the patient’s head is still and in its natural position, support of the head and neck can be used. In addition, hair should be secured away from the face to decrease noise data and facial expressions should be kept unchanged during the scanning process. Moreover, controlled indoor lighting is preferred to avoid image distortion owing to the sensitivity of the light sensors. Some interferences may be seen owing to the reflective surfaces of teeth. However, this could be corrected after image capture.
To obtain a holistic and more accurate 3D image, multiple scans can be taken in different facial positions (rest position, smiling position, and cheek retracted position). The scans are then aligned using the forehead to obtain more information on the patient’s smile line, tooth display, and soft tissue. Integrating 3D facial scans with intraoral and extraoral scans provides valuable information for treatment planning. If the operator wishes to scan the teeth separately, facial scanning software allows for the implementation of virtual facebow transfer technique. To use the virtual facebow technique, markers (round opaque stickers) are attached to the margin of the left or right eye orbit and the Beyron points on both sides.
After scanning is complete, the files are saved and imported into the software, which allows for size and lighting adjustments, display from various angles, and merging of 3D images. If the accuracy of the scan is perceived as less than optimal, the scanning software is used for postcapture manipulation including: smoothing skin, improving mesh quality, and filling holes in scan. After the completion of the necessary edits, the 3D scanned image is used for various treatment plans, such as orthodontic treatment and the fabrication of dental prostheses.
Role of intraoral scanners in the creation of a virtual patient
Different technologies are used to obtain a digital impression of teeth and surrounding intraoral tissues. These technologies are constantly updated and refined, so are the actual scanners to make them more user and patient friendly as well as easy to navigate and handle in the oral cavity. A strategic scanning technique that subsequently captures all areas and angles has to be used and become routine for maximum scanning efficiency and quality outcomes. Current intraoral scanners do not require the once-necessary antireflective powder and have to ability to scan. Besides eliminating all the uncomfortable aspects of a conventional impression, one of the great advantages of intraoral scanning is that select areas, which may not have been adequately captured, can simply be rescanned without having to retake the entire impression.
Intraoral scanners function by projecting structured light (white, red, or blue), which is recorded as individual images or video and compiled by the software after recognition of specific points of interest. A 3D model is then generated by matching the points of interest taken under different angles. Extreme points can also be eliminated statistically to decrease noise.
The time needed for taking a digital impression is less than that for a conventional impression. In addition, patients clearly prefer the digital scan. Digital full-arch impressions taken with an intraoral scanner seem to have slight deviations in respect to cross-arch accuracy. Other researchers have found similar accuracy levels between scanners. A recent literature review concluded that, currently, the literature does not support the use of intraoral scanners for long-span restorations on teeth or implants and there remain areas in respect to digital impressions of dental implants that need further investigation. However, new intraoral scanning technologies such as photogrammetric imaging allow for high accuracy even for full-arch implant restorations.
Scanning technique and sequence also play a major role. When properly applied, the digital impression technique seems to be more accurate than a conventional impression. , A surgical guide in preparation in a proprietary design software is shown in Fig. 2 (CoDiagnostix, Dental Wings GmbH, Düsseldorfer, Germany).

Traditionally, intraoral scanners were connected to mobile carts as a complete unit with the computer and monitor. More recent versions of scanners are connected wirelessly to laptop computers. Companies that produce scanners as well as dental chairs offer the option of incorporating the scanner into the dental chair.
CAD/CAM Design Software
The ability to visualize and analyze digital impressions immediately after scanning is one of the key advantages of the chairside workflow. Unlike conventional impressions, where errors are often only detected after fabricating the mastercast, an erroneous or deficient digital impression can be analyzed and corrected immediately.
Different software is available for each type of CAD/CAM system: clinical or laboratory. The reason is that restorations and materials for chairside CAD/CAM are more limited owing to time restrictions. The main objective of the chairside workflow is to complete the final ceramic restoration in 1 visit, avoiding the need for a temporary restoration and a second appointment for delivery. With the developments in high-strength ceramic materials and implant prosthetic solutions, the latest chairside CAD/CAM software versions are now able to produce fixed dental prosthesis and implant supported restorations. Dental restoration design software has become increasingly user friendly, with many features like preparation finish line detection and tooth digital “wax up” now automated. The clinician can select from digital libraries of natural tooth shapes and morphologies or create a mirror image of an existing tooth in the patient’s mouth. With these features, truly esthetic and natural tooth shapes that are not handmade by a dental technician can be applied based on the individual esthetic needs and desires of the specific patient. The .STL or other files and tooth libraries can be imported from other sources. Advanced options include digital smile design features and even face scan technology to optimize esthetic outcomes. ,
Several clinicians who want to incorporate digital impressions in their practice but do not want to mill and finish the restorations in house can select a semichairside workflow, which includes the intraoral scan without the design and milling of restorations. After review of the scan images and definition of the preparation margins, the digital impression is sent electronically to the dental laboratory where the restorations are fabricated. System-specific software is available for that feature (eg, Sirona connect, Dentsply Sirona). The laboratory can then use compatible laboratory-specific software for the design and manufacturing (eg, exocad, Exocad GmbH).
Archiving stone study models and mastercasts requires ample digital storage space. Digital scans and datasets can be stored and archived virtually on a designated server. It is important, however, to understand and follow patient data privacy rules and regulations when deciding where to store patient data and scan files. With most systems, CAD data are handled and transmitted in an .STL format, which has become the standard file format in 3D printing and rapid prototyping. Other formats that are currently used are .PLY, .DCM, and .UDX. To communicate with a milling machine, these file formats are “translated” into “millable” data file formats (CNC). The .STL files describe only the surface geometry of a 3D object without any representation of color, texture, or other common CAD model attributes.
The nitty gritty of digital intraoral scanning (optical scanners)
The pixel size, pixel dimension, or pixel resolution all refer to one thing only and that is the number of pixels in the image defined as X × Y , where X represents the number of pixel columns and Y represents the number of pixel rows. To obtain the value on megapixels, the X × Y value has to be divided by 1 million. Interaction with human–machine interfaces such as 2D and 3D printers, scanners, cameras, and computer monitors bring in a form of spatial resolution called DPI resolution, where DPI stands for dots per inch. It is sometimes referred to PPI or pixels per inch. If the DPI of an image 72, there will be 72 × 72 pixels in each square inch of the printed image if seen at full zoom, seen on screen and measured using virtual rulers. A change in the DPI can change everything in terms of printing. The following formula has to be kept in mind before any adjustments can be done.
The pixel size and DPI should maintain a constant relationship if the printed size has to remain constant. If the DPI is changed (and often can be while converting the format of the images), then it affects the printable quality of the image. This factor has applications in both digital radiography and digital photography.
The typical printable file format in 3D imaging is in standard triangulation language (STL) and the images are. STL image files. If a digital radiographic or photographic volume are to be printed, the DICOM (.DCM) image files have to be converted to .STL files. It is also possible now that a DICOM radiographic file and a .STL digital intraoral 3D photographic file can be matched and merged for the creation of a virtual patient.
Digital intraoral scanning generates a point cloud and these points join together and form an unfiltered image. Through interpolation, final image takes shape. Errors, known as Chord errors happen during the interpolation, leading to changes in the point density (resolution) generated by the scanner. The numbers of points generated in a digital intraoral scan would determine the scan resolution; the higher the point number, the higher the resolution. The resolution is obtained by a ratio of the number of points as noted in the .STL file and the surface are of the area examined and it is represented as points per millimeter squared (ppmm).
In a study looking at the various resolutions of intraoral scanners, it was noted that Omnicam from Sirona had the highest resolution (>79 ppmm)followed by TrueDefinition by 3M (>54 ppmm), Trios by 3Shape (>41ppmm) and iTero by Cadent (>34 ppmm). Each of the scanners have their own method of image capture from simple light oscillation or continuous 3D video capture to more complicated multiple colored beams forming a single white beam of light capturing single images and measuring out of plane coordinates of object points by sampling with continuous image acquisition.
Digital radiography (cone beam computed tomography imagers)
Resolutions in digital radiographic data can be isotropic or anisotropic depending on the closeness of the voxel to an isotropic cube. The word isotropic literally means, “equal direction.” The green prefix “an” makes it “imperfect” or “un-equal.” Hence, anisotropic means “un-equal directions or dimensions.” Digital radiographic data can be interpreted in physical dimensions either in pixel/voxel dimensions (micrometers) or spatial dimensions represented in line pairs per millimeter (lppm).
The spatial resolution of CBCT images is approximately 1 order magnitude lower than that of intraoral radiographs. The actual resolution would be closer to 1 lppm after accounting for the scatter effects, patient movement, and so on.
Cone beam computed tomography: the creation of virtual skeletal data
CBCT volume acquisition enables the dentist to be able to see the maxillofacial structures 3-dimensionally for not only diagnosis of dentoalveolar pathology, but also to plan the dental treatment including the restorations and prosthodontic care. Since the introduction of root form implants in dentistry, placement of dental implants are enabled by an accurate depiction of the mandibular and maxillary anatomy. The quality of the image depicted depends on the pixel resolution, which can be controlled to a certain extent by the operator. This would be contingent upon the type of CBCT machine and the manufacturer-set pixel resolutions based on the fields of view (FOV) chosen for the patient.
Essentially, there are 3 distinct broad categories that affect the final outcome of the CBCT volume and its diagnostic quality.
Category 1: Machine Properties
-
FOV chosen, voxel size, focal spot, noise, dynamic range, machine column stability, kV, mA, native acquisition software, and scanner calibration.
Category 2: Patient Related
-
Patient positioning, stability, density of skeletal structures and amount and density of restorative materials in the teeth.
Category 3: Software for Interpretation
-
Software design, ease of manipulation of the data, multimodal reformation with ease, 3D reconstruction and ability to read DICOM formatted basis images from several independent CBCT machines.
The Cone Beam Computed Tomography Revolution
CBCT is technology has been introduced to dental practice a little more than 2 decades ago, although conceptually the technology existed even longer. The first CBCT machines approved by the US Food and Drug Administration were commercially available in the United States since 2000. NewTom DVT9000 was the first CBCT unit (Quantitative Radiology srl, Verona, Italy) that was available for clinical use. Other machines were approved by the US Food and Drug Administration in quick succession as many US and foreign manufacturers started manufacturing similar units with various modes of acquisition and with optional FOV settings. Today, there are more than 20 different CBCT machines that are available commercially.
Cone Beam Computed Tomography Scan Acquisition
CBCT acquisition can be done with the patient in 3 possible positions, namely, standing, sitting, or supine. All 3 positions have advantages and disadvantages but in both sitting and standing positions, the patients’ head is restrained so that the movement is minimized. The restrain also is pertinent to supine position but the anticipated movement is very minimal if patients are instructed well and have a good neck support within the machine. The scan times vary and in general more than that needed for a panoramic acquisition.
Scan Volumes
CBCT acquisition is task dependent and there are several modes of acquisition ( Fig. 3 ).
-
Small volumes limited to area within 1 jaw (≤5 cm)
-
Singe arch (maxilla or mandible) (5–7 cm)
-
Medium volumes (both maxilla and mandible including skull base) (10–15 cm)
-
Large volumes (maxilla and mandible, skull base, and most of the cranium) (>15 cm)

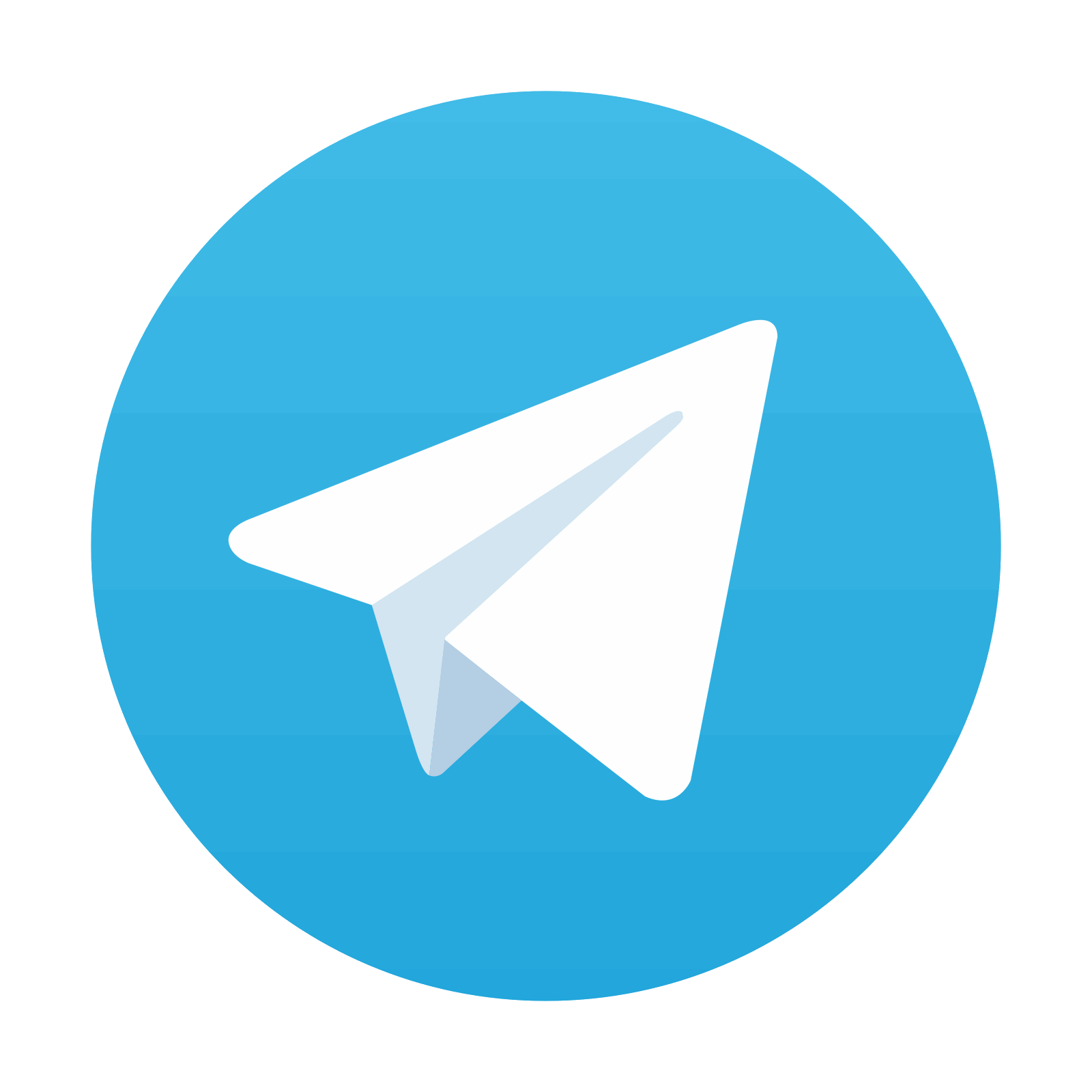
Stay updated, free dental videos. Join our Telegram channel

VIDEdental - Online dental courses
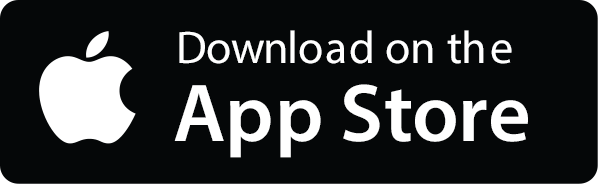
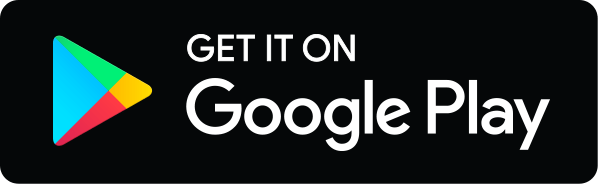
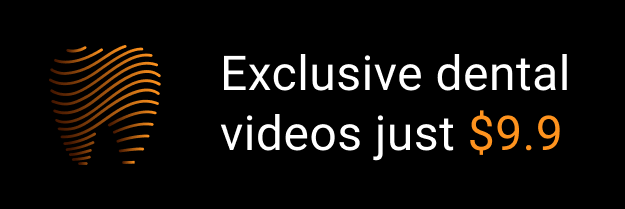