2
The Bioactive Properties of Dentine and Molecular Advances in Pulp Regeneration
Henry F. Duncan and Paul R. Cooper
Summary
A thorough understanding of the biology of the dentine-pulp complex is essential to underpin new regenerative treatments and maximise clinical impact within Endodontics. After microbial or traumatic insult, the dentine-pulp will mount a complex response, with a balance between infection control, inflammation, and host defence responses critical to determining tissue outcomes. Assessment of the regenerative capacity of the damaged pulp can be challenging as biomarkers of interest may exhibit functionality in both inflammation and repair. This balance is evident during reactionary and reparative tertiary dentinogenesis, which is driven by the release of bioactive dentine matrix components (DMCs) from the damaged dentine. DMCs contain a range of cytokines, chemokines, and growth factors, which are released following injury and orchestrate the recruitment, migration, proliferation, and differentiation of pulpal progenitor cells critical for the formation of newly deposited dentine. The field of regenerative endodontics embraces the repair, replacement, and regeneration of dentine-pulp tissue and needs to combine basic pulp biology research aimed at understanding molecular interactions in the tooth as well as clinical therapies, such as vital pulp therapy, revitalisation, and stem cell-based therapies. This chapter discusses current views of the molecular and cellular processes underlying both reparative and regenerative responses in the dentine-pulp complex.
2.1 Introduction
The concept of regenerative endodontics to replace damaged and lost tissues of the dentine-pulp complex is a topical and exciting clinical focus for the dental profession. In these procedures, the biology of the remaining pulp, dentine, and surrounding tissues is exploited to optimise the healing response following injury [1]. Regenerative strategies for the dentine-pulp complex whilst being exciting require careful consideration and further developments in our understanding of the biological processes that occur to enable us to advance beyond the traditionally reparative processes evidenced in vital pulp treatment (VPT) [2] and revitalisation [3].
The pulp is located at the centre of the tooth and is a dynamic connective tissue encased by an outer layer of mineralised dentine, enamel, and cementum. At the periphery of the pulp lies the odontoblast, a secretory cell responsible for formation of primary dentine during tooth development and also the slower production of secondary dentine throughout the life of the tooth. Beyond its formative role, the odontoblast also provides an ongoing protective role to the pulp, being able to upregulate its activity after challenge principally by caries, but also by trauma, chemical irritation and microbial leakage to form reactionary tertiary dentine at the site immediately beneath the insult [4]. This process enables the ‘walling off’ of the advancing irritation; however, if the stimulus is allowed to proceed, such as during a carious infection, it can overwhelm the tooth’s defences, leading to progressively severe inflammation [5], odontoblast death, and irreversible pulpitis, which ultimately leads to pulp necrosis. Notably, if the inflammatory process has not advanced significantly, the pulp possesses the ability to recover and repair, by removing the threat, potentially via clinical intervention, accompanied by the placement of a suitable sealing dental restoration [6]. Subsequently, the dead odontoblasts can be replaced by a new population of odontoblast-like cells, which are differentiated in a complex process from progenitor cells that likely reside within the dental pulp (including stem cell populations) in a process termed reparative dentinogenesis [7] (Figure 2.1).

Figure 2.1 Representation of the two types of tertiary dentine formation, reactionary and reparative dentinogenesis, formed in response to carious or traumatic lesions of differing intensities.
The control of the carious disease and pulpitis forms the basis of Operative Dentistry; however, the treatment of extremely deep caries and advanced pulpitis has traditionally been regarded as unpredictable and as a result, root canal treatment (RCT) has been indicated [8]. Although RCT preserves the tooth, removal of all the irreversibly damaged pulp tissue is a destructive and technically difficult process [8], that removes any inherent characteristics of the pulp such as perception, immunity, and repair, and leads to a greater risk of tooth fracture and loss [9]. As a result of this uncertainty, considerable attention has been diverted to new strategies aimed at modulating pulpal inflammation in cases of advanced pulpitis [8, 10, 11], as well as techniques to regenerate new pulp tissue [12], which can mimic the original dentine-pulp complex.
The aim of this chapter is to analyse current evidence on regenerative endodontics and reparative responses within the dentine-pulp complex, focussing on the role of dentinal components and molecular interactions, which occur during regenerative responses.
2.2 Regenerative Endodontics
Developing biological strategies to replace vital tissue in the root canal space has attracted significant attention under the term ‘regenerative endodontics’ [12, 13]. A working definition of regenerative endodontics was endorsed by the American Association of Endodontists (AAE) as ‘biologically based procedures designed to replace damaged structures, including dentine and root structures, as well as cells of the pulp-dentine complex’ [13]. This stresses treatments that replace dentine and root structures, which by definition exclude VPT procedures and experimental stem cell-based pulp regeneration therapies (see section 5.2). At present, according to this definition at least, regenerative endodontics only includes the cell-homing ‘revitalisation’ technique (see section 5.1), a cell-free technique in which endogenous host cells are ‘homed’ into the root canal system by chemotactically active growth factors (GFs) to re-establish a vital tissue [14]. Indeed, no consensus exists in pulp biology as to what reflects pulp regeneration; however, it appears illogical to lessen the clinical translation of regenerative endodontic procedures (REPs), by limiting the scope of this subject. Identifying a translational pathway where regeneration of a true physiological pulp-like tissue is the ultimate goal, but in the interim the stimulation of wound healing responses that satisfy some of the clinical issues in the dentine-pulp complex post-injury and would provide acceptable biologically based solutions appears appropriate [15]. The concept of VPT is long-established and encourages natural wound healing repair in the pulp. Other regenerative approaches, including revitalisation and experimental cell-based techniques, also promote pulp wound healing and may at present also be more reparative than regenerative in nature [3]. Encompassing a broad range of vital pulp preservation and regenerative approaches as part of regenerative endodontics can only be of benefit to the specialty and mirrors the approach taken in the wider field of regenerative medicine. Regenerative medicine has sought to be a broadly inclusive discipline as evidenced by statements from major bodies such as the United Kingdom’s Medical Research Council (MRC), which defined regenerative medicine ‘as an interdisciplinary field that seeks to develop the science and tools that can help repair or replace damaged or diseased human cells or tissues to restore normal function, and holds the promise of revolutionising treatment in the 21st century’ [16]. It would be helpful in the future to consider a working definition of regenerative endodontics which includes ‘the repair, replacement and regeneration of dentine-pulp lost due to age disease, trauma or congenital defects to restore normal function.’ [15].
Core Points
- Regenerative endodontics encompasses techniques to replace lost and diseased tissue of the dentine-pulp complex.
- At present, REPs only refer to the clinical procedure of revitalisation.
- Suggestions have been made that as the response is principally reparative the scope should be extended to include VPT.
- REPs also include experimental techniques in which harvested stem cells are re-implanted as part of a tissue engineering approach.
2.3 The Role of Dentine in Pulpar Repair and Regeneration
During VPT and cell homing procedures there is an opportunity for the operator to dynamically interact with the dentine matrix, which has been damaged and exposed by carious or traumatic injury. Mechanical contact with burs and instruments, chemical exchange using irrigating solutions, dental restorative materials, or scaffolds will not only affect cell populations but also interact with the dentine surface. Dentine contains a vast reservoir of bioactive dentine matrix components (DMCs) fossilised during matrix development [1]. Many bioactive groups have been identified including bone morphogenetic proteins (BMPs), transforming growth factor-beta (TGF-β), neurotrophins, vascular growth factors (e.g. vascular endothelial growth factor [VEGF], platelet-derived growth factor [PDGF]), and tissue proteases [17–19], with the therapeutic harnessing of these molecules critical to the process of dentine-pulp repair [1]. Indeed, the release of bioactive components, including angiogenic, metabolic, and chemotactic cytokines, is likely key to enabling regenerative and reparative processes following caries or trauma, or during revitalisation procedures [19, 20, 21]. In support of this, the ability of ethylenediaminetetraacetic acid (EDTA) [22, 23], mineral trioxide aggregate (MTA) (Dentsply Tulsa Dental, Tulsa, OK, USA) [24], calcium hydroxide [Ca(OH)2] [25], dental adhesives [26], ultrasonic activation [27], and epigenetic modifiers (histone deacetylase inhibitors) [28] to facilitate the release of DMCs and augment the regenerative response, has been demonstrated.
The use of irrigants such as EDTA in preference to sodium hypochlorite (NaOCl) during chemical disinfection, may provide additional benefit during REPs due to its stimulation of the release of a range of DMCs including GFs beneficial to cell migration, proliferation, and differentiation [29, 30]. Notably, irrigating with a 17% EDTA solution can stimulate the release of TGF-β family members from the extracellular matrix of dentine [25], whereas conversely NaOCl, particularly in high concentrations (>1.5%), may have a deleterious effect on stem cell survival and differentiation ability, leading to a recommendation that the final rinse required to enable cell-homing procedures should be with a 17% EDTA solution [31]. DMCs are capable of inducing odontoblast-like cell differentiation [32], and two GFs in particular, bFGF and/or VEGF, both contained in dentine, have been shown to promote formation of a pulp-like tissue in a cell-homing procedure [33]. Other studies have highlighted that although angiogenic GFs are present in dentine [34], they are insufficient to induce endothelial differentiation and vascular development in vivo [35].This stimulates the question as to whether the supplementation of exogenous GFs may be beneficial to the success of in REPs.
Core Points
- Dentine matrix contains a diverse pool of bioactive molecules, which are released during injury and therapeutic procedures to facilitate reparative processes.
- Release of these molecules can be stimulated during REPs by irrigation, medicament, and materials placement.
- EDTA is conducive to DMC release, whereas NaOCl has a negative effect on stem cell recruitment processes.
- Endogenous GFs are essential and can be harnessed for the healing response in REPs.
2.4 Infection, Inflammation, and Stem Cells Interaction in Pulp Regeneration
2.4.1 Immune Response
In early stage carious disease, enamel and dentine become infected and the bacterial acids produced demineralise and soften the hard tissues. Due to the porous and tubular nature of the dentine, bacterial products and components can traverse the dentine resulting in a localised pulpal inflammatory response beneath the site of the lesion [36]. As the carious infection progresses, the composition of the invading bacterial microflora evolves and becomes predominantly anaerobic within deeper carious lesions [37, 38]. Concomitantly, the localised inflammatory response also becomes amplified. Ultimately, the carious infection, if untreated, will reach the pulpal tissue and this can lead to irreversible pulpitis, pulp necrosis, and eventually apical periodontitis [5].
During the infectious process, the molecular response within the soft pulpal tissue is complex due to the signalling that occurs during the host’s defensive reaction. Cells within the tissues detect the bacterial components and products and initially respond in ways aimed at limiting and eliminating the infectious agents. Odontoblasts and core pulpal cells, such as fibroblasts, stem cells, neurones and endothelial cells, are known to express a range of receptors able to detect bacterial components, including bacterial wall components such as lipopolysaccharides (LPS) and lipoteichoic acids (LTA), as well as bacterial DNA (Figure 2.2). Members of the Toll-like receptor (TLR) family, of which there are nine in man (TLRs 1–9), are the most well-characterised receptor family which play a role in detecting the infection and orchestrating the inflammatory response. Once the bacterial ligands are bound to the receptors, downstream intracellular signalling pathways are invoked, such as those involving nuclear factor kappa B (NF-kB) and mitogen activated protein (MAP) kinase [39]. These pathways result in the cellular release of antimicrobial peptides (AMPs) and inflammation-regulating cytokines. The released cytokines signal a range of host tissue responses involving immune cell recruitment, extravasation, activation, differentiation, and antibody production. Examples of cytokines shown to be elevated during pulpal disease include interleukins (IL)-1α, -1β, -4, -6, -8, -10, and tumour necrosis factor-alpha (TNF-α). Only after the infection is removed, following clinical intervention (as an example), the levels of these cytokines will be decreased and restored to those present during tissue homeostasis. Conversely, chronic inflammation will persist within the tooth if the infection is not cleared, leading to swelling, pain, and tissue necrosis, and this will limit the likelihood of any tissue repair mechanisms occurring [40, 41].

Figure 2.2 A schematic representation of cellular and molecular processes involved in dentine-pulp complex immune response to infection. (A) Bacteria colonize and infect the wound and are subsequently detected by tissue resident cells, including immune cells such as (Bi) dendritic cells and (Bii) mast cells. Vasoactive molecules and chemokines/cytokines are released to generate signals and gradients to enable further immune cell populations, such as (C) neutrophils, to be recruited from the bloodstream. Subsequently, neutrophils mediate (D) bacterial killing. The cycle of the inflammatory response continues until the infection is cleared. Macrophages will remove bacteria and cellular debris and drive resolution of inflammation, and, subsequently, wound regenerative processes will be invoked to enable tissue healing. Reproduced from Wei et al. [43] / with permission from Elsevier.
2.4.2 Inflammation and Regeneration
It is notable that the pulpal inflammatory response is double-edged. During chronic infection, with relatively high levels of inflammation ensuing, collateral host tissue damage occurs as immune cells traverse the pulpal tissue to localise to the site of infection. Notably, immune cells such as neutrophils are well reported to release tissue degrading enzymes, such as matrix metalloproteinases (MMPs), that are necessary for their migration. Furthermore, for antimicrobial killing, these cells generate significant amounts of reactive oxygen species (ROS). These molecules, whilst targeted at the infecting bacteria, also cause significant collateral tissue damage due to their deleterious effects on cellular structures, DNA, and proteins. Locally produced ROS can also stimulate further cytokine release via activation of key proinflammatory intracellular signalling mediated by the previously mentioned p38 MAP kinase and NF-kB pathways, and this process perpetuates the inflammatory cycle [42, 43]. The relatively novel bacterial killing and containment mechanism, termed neutrophil extracellular traps (NETs), which involves the release of intracellular DNA decorated with AMPs targeted at the infected bacteria, may also cause collateral host tissue damage as well as exacerbate the pulpal inflammatory response and even cause local pulp cell death [44, 45].
It has become apparent that persistent and chronic inflammation directly impedes tissue repair processes, and the currently accepted paradigm is that pulp healing can only occur after removal of the infection along with a significant dampening of the inflammatory process [46–48]. Indeed, both in vitro and in vivo studies have demonstrated the biphasic effects of proinflammatory mediators [21, 46, 48–52]. At relatively low levels of pro-inflammatory mediators, such as cytokines TNF-α and TGF-β, as well as ROS and bacterial LPS, stimulation of dental cell-mediated repair process occurs. However, when these same molecules are present at relatively high levels, for example during persistent and chronic infection and inflammation, deleterious effects on pulpal repair processes occur, such as induction of cell and tissue death. In addition, it is reported that stem cell differentiation processes necessary for tissue repair can be directly inhibited by relatively high levels of local pro-inflammatory mediators [39]. It is therefore likely that without appropriate clinical intervention, the tooth will be lost due to the ensuing infection and associated chronic inflammation. Indeed, if dental disease endures, then the pulp will become necrotic and require urgent remedial dental treatment; indeed, RCT aimed at preserving the tooth’s functionality may be necessary. Notably, such procedures are destructive, relatively expensive, and weaken the tooth structure, which will likely limit the life span of the tooth [53]. Indeed, while RCT is relatively frequently performed as a dental procedure, in the long term 10–50% of cases eventually fail, with a greater percentage being unsuccessful in general dental practice [54]. Underlying risk factors are likely due to a compromised efficacy of chemo-mechanical debridement as well as certain patients having an innate adverse response to the treatment [55].
2.4.3 Opportunities for Clinical Translation
Historically, it has been proposed that calcium hydroxide-based dental materials may stimulate dental repair responses due to their ‘irritation’ of the pulp tissue [56]. Indeed, such a response would likely involve a resolving sterile inflammation as the tissue ultimately returns to a homeostatic state. Interestingly, the mechanism of action of hydraulic calcium silicate materials, such as MTA (Dentsply Tulsa Dental, Tulsa, OK, USA), have been attributed to their ability to stimulate a localised cytokine burst, including release of IL-1α, IL-1β, IL-2, IL-6, and IL-8, from odontoblasts and osteoblasts. This relatively mild and acute material-induced inflammatory may therefore provide an explanatory mechanism as to how this class of material enables dental tissue repair [57–59]. Some studies also attempt to exploit the positive interaction between inflammation and regeneration by developing materials that modulate these processes. The tricalcium silicate-based material BiodentineTM (Septodont, Saint-Maur-des-Fossés, France) has been shown in vitro to exert anti-inflammatory effects in terms of growth factor and cytokine signalling in pulp fibroblasts, while processes relating to tissue healing were promoted [60]. Although, the mechanism responsible for this modulation was not identified, this type of study indicates that the development of smart materials capable of working with complex host cell and tissue responses could be used to drive beneficial restorative outcomes.
In addition, the use of naturally derived therapeutic modulators of inflammation are being explored for use adjunctively or by incorporation into dental treatment protocols to facilitate tissue healing and aid restoration longevity. Some studies have reported that dental resin restorative procedures, which contain powerful antioxidants such as N-acetylcysteine (NAC), may protect pulp cells from the deleterious and proinflammatory effects of locally produced ROS. Interestingly, NAC may also have antibacterial activity targeted against multispecies endodontic biofilms [61, 62]. Combined, these data indicate that materials which combat infection and minimise inflammation may create a conducive environment for dental tissue repair. Numerous studies have assessed the anti-inflammatory effects of naturally derived compounds within the pulp [63–67]. For example, pachymic acid, derived from the mushroom Fomitopsis niagra, not only exerts an anti-inflammatory effect but also promotes odontoblast-like cell differentiation in vitro [68].
Core Points
- The immune and inflammatory responses within the dental pulp begin early, once the dental hard tissue becomes infected with cariogenic bacteria due to the communication created by the tubular structure of the dentine.
- The molecular and cellular immune responses within the dental pulp are complex and aim to defend the tissue to enable repair processes to occur.
- The host’s defence and inflammatory responses can have deleterious effects on the pulp tissue as immune cells traverse the tissue and combat the infecting bacteria.
- Relatively low levels of inflammation can invoke dentine-pulp complex repair processes while relatively high levels of inflammatory mediator will inhibit tissue repair.
- Following disease resolution and appropriate clinical intervention, the dentine-pulp complex can invoke the tooth tissues’ innate repair responses.
2.5 Regenerative Endodontic Procedures (REPs)
2.5.1 Cell-Homing
Currently the only REP available for clinicians is revitalisation, which is a treatment for immature teeth with non-vital pulps and an open apex. Management of pulp necrosis in mature teeth requires RCT; however, if root formation is not complete, the combination of thin dentine walls and open apices make completion of conventional RCT impossible [69]. Furthermore, immature teeth that have lost pulp vitality are also more vulnerable to injury, losing the ability to sense environmental change, and are therefore more prone to root fracture than mature teeth [70, 71]. From a biological perspective, the treatment of immature teeth with non-vital pulps requires the same level of disinfection and removal of necrotic tissue as mature teeth with non-vital pulps, albeit with a reduced or absence of root canal instrumentation. The revitalisation procedure is a cell-honing therapy, which is advocated as a replacement for apexification and root-end closure techniques and as a result those procedures are briefly summarised for comparison.
2.5.1.1 Apexification and Root-end Closure
Traditional treatment of immature teeth is by apexification, a technique that, over several months, induces an apical mineralised barrier using non-setting Ca(OH)2 materials. During the apexification procedure, the necrotic tissue is removed using chemo-mechanical disinfection, the canal dried and dressed with a non-setting Ca(OH)2 paste placed carefully to the tooth apex with an endodontic plugger, spiral filler or root canal file in order to ensure appropriate contact with the apical tissues. The Ca(OH)2 is generally changed after 1 month, and thereafter every 3 months until the formation of the apical barrier is complete. This apical barrier is usually formed of poor quality cementum-like tissue, which is visible on radiographic views and can be confirmed by gentle palpation with a paper point. This process takes anywhere from 6 to 18 months to complete and once successful treatment is confirmed the canals can be filled with thermoplastic gutta-percha and an appropriate sealer. From a practical perspective, apexification is clearly time-consuming, technically difficult [72], and costly for both patients and dentists, while the predictable creation of a mineralised barrier is hard to achieve [73]. Notably, root fracture is significantly increased with long-term Ca(OH)2 dressing in immature teeth [70], a discovery that has stimulated research into finding alternative therapeutic approaches.
Root-end closure techniques, which involve placing a hydraulic calcium silicate matrix (e.g. Biodentine, MTA) at the apex of the tooth in one or two visits [74, 75], are preferred as they significantly reduce treatment time, limiting the risk of loss of patient compliance or root fracture. Although not essential, the use of magnifying loupes or the operating microscope facilitates the ease of performing this treatment, which at least initially is carried out in an identical manner to apexification using Ca(OH)2. A hydraulic calcium silicate, ideally possessing a radio-opacity greater than dentine, is placed carefully with an endodontic plugger or specialised MTA carrier, until a 5 mm thickness of the material is achieved from the radiographic root apex [75]. Thereafter, the root canal can be dressed with a moist cotton pellet or paper point prior to the placement of thermoplastic gutta-percha at a subsequent visit in a two-stage process. Alternatively, the canal can be filled in a single visit if the hydraulic calcium silicate material sets. Notably, treatment outcome comparisons have highlighted a similar or improved response with root-end closure using MTA over traditional apexification techniques using Ca(OH)2 [76, 77]. However, both these techniques are reparative and are not designed to induce extension of root length or width [78, 79] (Figure 2.3). As a result, a poor long-term prognosis is generally expected due to cervical root fracture [80]. Therefore, strategies to either maintain the healthy pulp or stimulate the development of new biological tissue are paramount, not only to promote minimally invasive solutions but also to retain or reinstate the capacity of the pulp to generate tertiary dentine and respond to injurious stimuli [81].

Figure 2.3 (A) Open-apex maxillary left central incisor (21) with an apical radiolucency. (B) Intra-appointment check radiographic view after root-end closure carried out using a commercial hydraulic calcium silicate material matrix. (C) Backfill with thermoplastic gutta-percha carried out and coronal restoration placed. (D) At review 6 months later, there is no evidence of continued root formation or root thickening as is the case with root-end closure procedures.
2.5.1.2Revitalisation
Pulp revitalisation procedures have become popular under the umbrella of REPs [13], while cell-based therapies remain a largely experimental technique (see section 5.2) [12] (Figure 2.4). The concept of a biological revascularisation of the empty root canal space is, however, not new with previously successful revascularisation procedures carried out in immature teeth with necrotic pulps in the 1970s [82]. Although that pioneering work demonstrated the potential for a pulp revascularisation procedure to induce apical closure and continuous root formation in immature human teeth [82], it was not until nearly 30 years later that interest in revitalisation was rekindled after human studies reported the ingrowth of connective tissues, blood vessels, dentine, and cementum-like tissues following application of pulp revitalisation procedures [83]. Indeed, Shimizu and colleagues [83] highlighted the potential of this revitalisation to regenerate biological tissue if normal periapical tissues containing Hertwig’s epithelial root sheath (HERS) and the apical papilla remained healthy. Notably, if there was an abscess present, then the repair was cementum-bone-like and reparative in nature [3]. Clinically, the attraction of a cell-homing strategy in clinical endodontics in comparison with a cell-based therapy is clear, as there is no requirement for exogenous stem cells to be used within the tissue engineering strategy [84]. Stem cell transplantation into an empty root canal space has several inherent problems including regulatory difficulties, source and isolation of stem cells, high-cost of storage and expansion, and biological risk such as immune-rejection and tumorigenesis [85], as well as finding a suitable way to deliver the cells effectively into the root canal space. That said, revitalisation predictability is also compromised by a lack of cells; however, recent reports demonstrated pulp-like regeneration using a cell-homing technique employing cocktails of GF morphogens in an ex vivo model [33]. Indeed, the use of GFs and functionalised bioactive scaffolds may provide an appropriate therapeutic strategy by which pulp revitalisation may be achieved in the future.

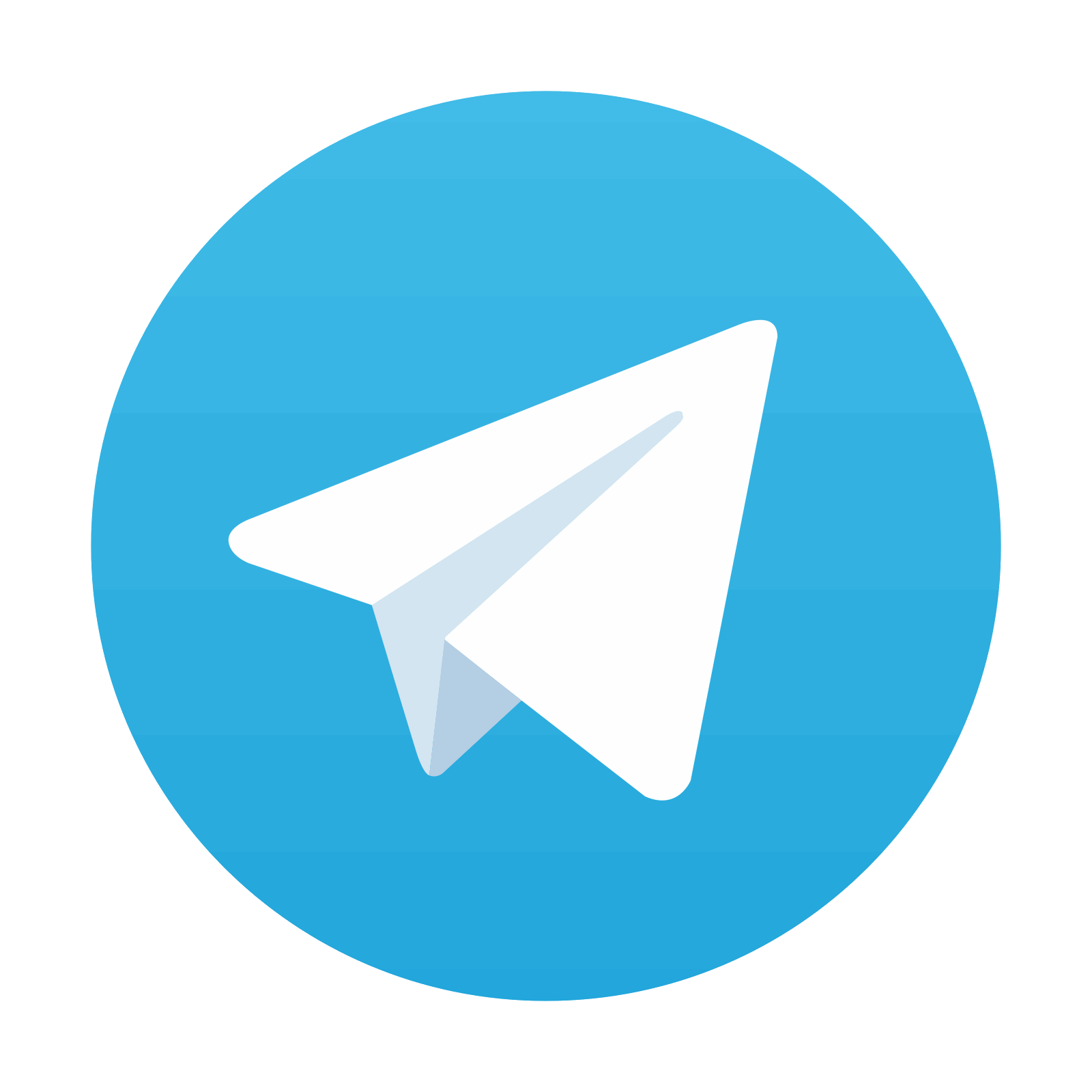
Stay updated, free dental videos. Join our Telegram channel

VIDEdental - Online dental courses
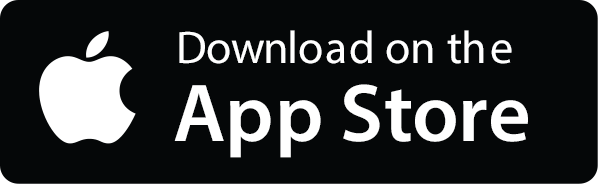
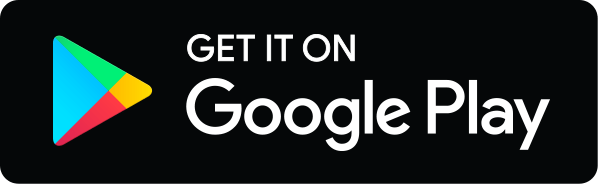