Abstract
Computer-guided piezocision can be used to overcome the disadvantages of corticotomy in accelerating orthodontic tooth movement. The aim of this clinical pilot study was to determine the accuracy of this technique. STROBE guidelines were followed. Ten patients were selected and treated. Using new dedicated planning software, the piezocision cuts were properly positioned in virtual models. A surgical guide was designed and printed with slots to guide the scalpel blade first and then the piezoelectric micro-saw. The slots limit the cortical incisions coronally and apically, and also limit the depth of penetration of the piezoelectric micro-saw. The patients underwent CBCT before and immediately after surgery, and the pre- and postoperative images were matched. Using software, the planned piezocisions were compared to the actual piezocisions, and the entry point and depth deviations were measured. Descriptive statistics, kappa statistics, and the t -test were used for the data analysis. The mean deviation at the entry point was 0.67 mm (range 0.06–1.44 mm, standard deviation 0.31). The mean depth deviation was 0.54 mm (range 0.17–0.80 mm, standard deviation 0.21). The limits of the use of computer-guided piezocision are set by the maximum deviation observed; thus a safety distance of 1.5 mm should be considered, which confirms that this innovative technique is clinically applicable.
Corticotomy is an effective technique to accelerate orthodontic tooth movement, but it is characterized by significant postoperative discomfort . The original technique requires the elevation of mucoperiosteal flaps and a long surgical time, resulting in a reluctance to employ this technique among both patients and the dental community .
To overcome the disadvantages of the corticotomy, Dibart et al. introduced the concept of ‘piezocision’ . This procedure is characterized by small mucoperiosteal vertical incisions, minimal piezoelectric osseous cuts to the buccal cortex only, and bone or soft tissue grafting . Even more recently, an innovative, minimally invasive, flapless procedure combining piezoelectric surgery cortical micro-incisions with the use of a three-dimensionally printed computer-aided design and computer-aided manufacturing (CAD/CAM) surgical guide has been reported in the literature (computer-guided piezocision) . This computer-guided piezocision procedure offers ‘static’ guidance via a surgical guide that prevents changes to the surgical plan at the time of surgery . The slots of the template allow for rigidly-guided and highly-controlled piezocision . Using three-dimensional (3D) planning software, the surgeon can, after consulting the orthodontist, provide a guide representing the planned proper positions of the virtual piezocision cuts . When the planned piezocision is incorporated into the computed tomography (CT) images, the planning can take into account both the jawbone anatomy and the roots of the teeth . Using this technique it is possible to decrease the length of time of orthodontic treatment without periodontal tissue damage .
The limited deterioration in postoperative oral health-related quality of life (OHRQoL) and the effectiveness of computer-guided piezocision are encouraging and may prompt the more widespread clinical use of this technique; however, the accuracy of this technique has not yet been evaluated . In computer-guided surgery, the risk of deviation (transfer error from the software planning stage to the surgical field) is significant . As in computer-guided implantology, computer-guided piezocision involves a sequence of diagnostic and therapeutic events, and errors can be introduced at different stages .
The primary objective of this clinical pilot study was to determine the deviations in 3D directions between the virtual planned piezocisions and the actual piezocisions using the computer-guided technique (i.e., its accuracy). The frequency of surgical complications and unexpected events was also recorded in order to assess the application of this static computer-guided technique in clinical practice. It was hypothesized that a deviation would exist between the planned piezocisions and actual piezocisions, but that the accuracy measurement would allow an appropriate safety distance to be determined. It was also hypothesized that there would be no surgical complications or unexpected events.
Materials and methods
This prospective clinical pilot study was conducted in the department of orthodontics of the study institution in Rome, Italy, between March 2015 and July 2016. Two hundred and thirty patients were assessed for eligibility. Two hundred and twenty patients were not eligible, as they did not meet the inclusion criteria, detailed below. Thus, 10 patients were assigned to receive corticotomy-facilitated orthodontic treatment using computer-guided piezocision. The first patient was enrolled on 9 March 2015, and the final patient was enrolled on 1 June 2016. No patient was excluded at any stage of the study. The STROBE guidelines were followed in this study (Strengthening the Reporting of Observational Studies in Epidemiology). Written consent was obtained from each participant. The investigation was reviewed and approved by the local ethics committee.
The eligibility criteria were as follows: systemically healthy patient; no clinical evidence of dental caries; good oral hygiene; skeletal and molar class I relationships; crowding of both arches (4 mm or more of discrepancy); willingness to participate in the study .
Dedicated software and a modified 3D-printed CAD/CAM surgical guide were used in the present study, as described below.
Planning phase
First, a preliminary impression of the dental arches was taken. A customized impression tray was manufactured, and a second impression was then taken. Using a thermoplastic impression compound in stick form to mould the border, the impression tray was extended as far as possible into the vestibular fornix. The final impression was taken using a silicone material (polyvinylsiloxane). The cast was poured and the model digitally acquired using a 3D scanner (Easy Optical 3D Scanner; Open Technologies, Rezzato, BS, Italy). The cast scanner images were saved as stereolithography (STL) files and stored.
The patient then underwent cone beam computed tomography (CBCT) using a personalized radiological tray (radiological matching tray) with radiopaque landmarks to obtain a perfect overlap of the jaw and cast STL files ( Fig. 1 ). Digital Imaging and Communications in Medicine (DICOM) images were acquired using software (Cortex; Media Lab, Milano, Italy), allowing the segmentation of 3D medical images. Images of the jaws were transformed into 3D models and saved as STL files. With the same software (Cortex; Media Lab, Milano, Italy) and using the radiological matching tray as reference, the STL files of the jaw and cast were matched.

Using the 3D planning software (Cortex; Media Lab, Milano, Italy), the piezocision cuts were appropriately positioned in the virtual models ( Fig. 2 ). When the cast images were matched with the CT images, the planning took into account both the jawbone anatomy and the extension of the guide (Note: it is not possible to extend the surgical guide beyond the limits determined by the impression taken, i.e. beyond the limits of the fornix that the plaster cast defines). The software (Cortex; Media Lab, Milano, Italy) permitted a view of the 3D model from different perspectives and planes with perfect rendering. The space between the roots of each tooth was evaluated from the right second molar to the left second molar, and corticotomy cuts were drawn with the longitudinal axis parallel to each root. Following the direction of this pre-determined longitudinal axis, a template was designed, characterized by the presence of guide slots of a predetermined height ( Fig. 3 ).


The slots delimit a space of 1 mm and limit the cortical incisions coronally and apically. The width of the slot is proportional to the thickness of the piezoelectric cutting insert (0.55 mm).
The slots were designed to be 2 mm from the papilla (coronal limit preventing damage to the periodontal tissues) and 2 mm above the apex of the teeth (this apical limit is the minimum extension of the cortical bone incision required to achieve effective acceleration of orthodontic movement). The height of the slots was determined in order to allow the piezoelectric micro-saw to penetrate at most 10 mm into the slot, so that the depth of the piezocision would correspond to the virtual project.
A 3D model of the guide was then transformed (i.e., printed) into a surgical guide with the slots designed to guide the scalpel blade first and then the piezoelectric cutting insert (OT7). The 3D STL model of the surgical guide was printed using an Objet30 OrthoDesk 3D Printer for medical devices (Stratasys, Eden Prairie, MN, USA) ( Fig. 4 ).

Planning phase
First, a preliminary impression of the dental arches was taken. A customized impression tray was manufactured, and a second impression was then taken. Using a thermoplastic impression compound in stick form to mould the border, the impression tray was extended as far as possible into the vestibular fornix. The final impression was taken using a silicone material (polyvinylsiloxane). The cast was poured and the model digitally acquired using a 3D scanner (Easy Optical 3D Scanner; Open Technologies, Rezzato, BS, Italy). The cast scanner images were saved as stereolithography (STL) files and stored.
The patient then underwent cone beam computed tomography (CBCT) using a personalized radiological tray (radiological matching tray) with radiopaque landmarks to obtain a perfect overlap of the jaw and cast STL files ( Fig. 1 ). Digital Imaging and Communications in Medicine (DICOM) images were acquired using software (Cortex; Media Lab, Milano, Italy), allowing the segmentation of 3D medical images. Images of the jaws were transformed into 3D models and saved as STL files. With the same software (Cortex; Media Lab, Milano, Italy) and using the radiological matching tray as reference, the STL files of the jaw and cast were matched.
Using the 3D planning software (Cortex; Media Lab, Milano, Italy), the piezocision cuts were appropriately positioned in the virtual models ( Fig. 2 ). When the cast images were matched with the CT images, the planning took into account both the jawbone anatomy and the extension of the guide (Note: it is not possible to extend the surgical guide beyond the limits determined by the impression taken, i.e. beyond the limits of the fornix that the plaster cast defines). The software (Cortex; Media Lab, Milano, Italy) permitted a view of the 3D model from different perspectives and planes with perfect rendering. The space between the roots of each tooth was evaluated from the right second molar to the left second molar, and corticotomy cuts were drawn with the longitudinal axis parallel to each root. Following the direction of this pre-determined longitudinal axis, a template was designed, characterized by the presence of guide slots of a predetermined height ( Fig. 3 ).
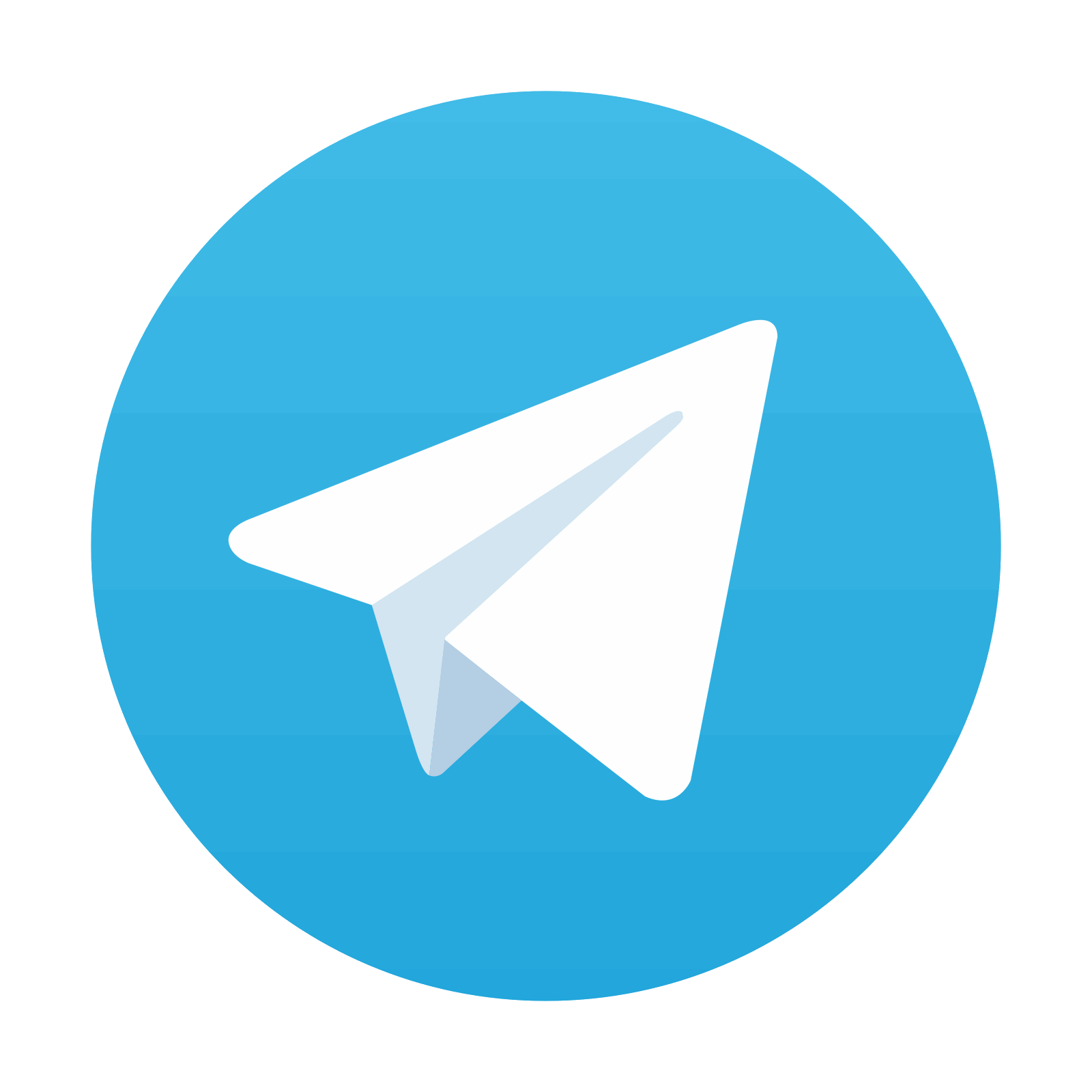
Stay updated, free dental videos. Join our Telegram channel

VIDEdental - Online dental courses
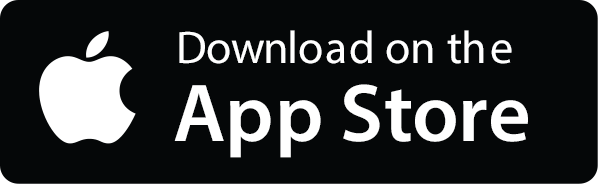
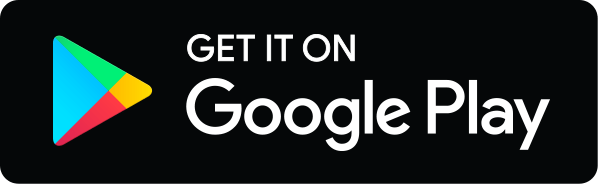
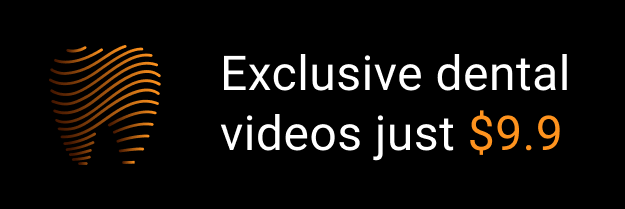