Abstract
Objectives
Despite their various advantages, such as good esthetic properties, absence of mercury and adhesive bonding to teeth, modern dental composites still have some drawbacks, e.g., a relatively high rate of secondary caries on teeth filled with composite materials. Recent research suggests that microstructured biomaterials surfaces may reduce microbial adhesion to materials due to unfavorable physical material–microbe interactions. The objectives of this study were, therefore, to test the hypotheses that (i) different surface microstructures can be created on composites by a novel straightforward approach potentially suitable for clinical application and (ii) that these surface structures have a statistically significant effect on microbial adhesion properties.
Methods
Six different dental composites were initially tested for their suitability for microstructuring by polydimethylsiloxane (PDMS) templates. Each composite was light-cured between a glass slide and a microstructured PDMS template. The nano-hybrid composite Grandio Flow was the only tested composite with satisfying structurability, and was therefore used for the bacterial adhesion tests. Composites samples were structured with four different microstructures (flat, cubes, linear trapezoid structures, flat pyramids) and incubated for 4 h in centrifuged saliva. The bacterial adherence was then characterized by colony forming units (CFUs) and scanning electron microscopy (SEM).
Results
All four microstructures were successfully transferred from the PDMS templates to the composite Grandio Flow. The CFU-test as well as the quantitative analysis of the SEM images showed the lowest bacterial adhesion on the flat composite samples. The highest bacterial adhesion was observed on the composite samples with linear trapezoid structures, followed by flat pyramids and cubes. The microstructure of dental composite surfaces statistically significantly influenced the adhesion of oral bacteria.
Significance
Modifying the composite surface structure may be a clinically suitable approach to control the microbial adhesion and thus, to reduce the risk of secondary caries at dental composite restorations. Smaller composite surface structures may be useful for accomplishing this.
1
Introduction
Since the introduction of resin-based dental materials around the middle of the last century, composite restorations in dentistry became indispensable because of the patients’ esthetic demands and ease of composite processing . Composites are a mixture of organic and/or inorganic fillers surrounded by a monomer matrix which can be set-on-command by photopolymerization e.g. with blue LED lamps . Depending on their filler particles sizes composites can for example be categorized into four different groups: macro-, micro-, hybrid- and nanofiller composites. In addition, they can be categorized according to their rheological properties into flowable and non-flowable composites.
Despite their advantages, such as good esthetic properties, absence of mercury and adhesive bonding to teeth, dental composites still have some drawbacks, such as for example polymerization shrinkage, their tendency to absorb water and the onset of secondary caries caused by microbes on teeth filled with composite materials .
A challenge arises through the surface treatment of composites. Inappropriate finishing procedures may result in increased surface roughness . An important property in relation to the structural surfaces roughness of dental composites is the adhesion of oral microbes to the composites. Surface roughness influences bacterial colonization , particularly on composite materials . Smooth surfaces are preferred clinically, because of their relatively low bacteria adherence . Carlén et al. reported, however, a polished hybrid composite to accumulate more bacteria than the unpolished one .
Some investigations showed that microbes adhere stronger on composites surfaces than to the natural tooth covered by a pellicle or in comparison to other dental materials. A threshold level of composite surface roughness of R a = 0.2 μm has been discussed, below which no further reduction in microbial accumulation could be expected , however, no convincing explanation for this has been given. Although surface roughness seems to be an important factor for microbial accumulation on dental composites, materials properties, such as filler-size shape and content , composite surface tension , chemical surface composition , protein adsorption and others seem to be important factors as well.
It has been predicted that future commercial dental composites will possess antimicrobial properties and the quantity of scientific literature addressing this subject has grown strongly over the last years . Approaches to equip resin based dental materials with antimicrobial properties include silver or zinc oxide nano particles , silver-supported antibacterial materials , zinc oxide eugenol , quaternary ammonium functionalities , alkylated ammonium chloride derivatives , chlorhexidine diacetate (CHXA) , carolacton and others. The addition of antimicrobial agents to composites, however, may lead to reduced mechanical properties of the composites and in many cases the antimicrobial effect of the composites is not sustainable .
A new and promising approach to reduce the microbial adhesion to different biomaterials surfaces uses specific micro or nano surface topographies or patterns . The microbial adhesion reduction mechanisms of these materials surfaces are still an enigma, but some authors assume that an unfavorable physical interaction between microbes and the materials surface is responsible for their antimicrobial effect .
Reducing microbial adhesion to materials with this approach has a number of advantages since it uses neither antibiotics nor other chemical antimicrobial agents or compounds. Hence these materials cannot lead to antibiotic resistance of microbes or negative side effects of drug release, such as cytotoxicity to body cells.
Based on these findings, the question arises if a surface structuring approach is also feasible for reducing microbial adhesion to dental materials, such as dental composites. Little is known about the interaction of microbes and flowable composites. Due to their rheological properties flowables seem to be, however, the ideal materials for surface patterning.
It was, therefore, the aim of this current study to test the hypotheses that (i) different surface microstructures can be created on composites by a novel straightforward approach potentially suitable for clinical application and (ii) that these surface structures have a statistically significant effect on microbial adhesion properties when compared with flat control samples of the same composite. To the best of our knowledge, neither has been attempted previously and may, if successful, lay the foundations for a new way of functional surface structuring of dental composites.
2
Materials and methods
2.1
Dental composites
Six different composites were first tested for their structurability by polydimethylsiloxane (PDMS) templates: the nanohybride composites CLEARFIL MAJESTY Posterior (CMP; Kuraray Europe GmbH, Frankfurt, Germany), Grandio Flow (GF; Voco GmbH, Cuxhaven, Germany), Premise (P; Kerr Corporation, Orange, USA), Tetric EvoFlow (TEF; Ivoclar Vivadent AG, Schaan, Liechtenstein), Venus Diamond Flow (VDF; Heraeus Kulzer GmbH, Hanau, Germany) and the microhybrid composite XFlow (XF; Dentsply International, York, USA). All composites used in the current study were of the shade A2. The properties of the composites, as obtained from manufacturers data sheets, are listed in Table 1 .
Composite | Company | Filler content | Filler size | Filler material |
---|---|---|---|---|
CLEARFIL MAJESTY Posterior (CMP) | Kuraray Europe GmbH | 92% (w/w) 82% (v/v) |
20 nm–1.5 μm | Aluminum oxide, glass |
Grandio Flow (GF) | Voco GmbH | 80% (w/w) 65.6% (v/v) |
40 nm–1 μm | Glass, SiO 2 |
Premise (P) | Kerr Corporation | 84% (w/w) 70% (v/v) |
20 nm/0.4 μm/30 μm | Prepolymerized fillers, glass, SiO 2 |
Tetric EvoFlow (TEF) | Ivoclar Vivadent AG | 57.5% (w/w) 30.7% (v/v) |
550 nm | Glass, ytterbium trifluoride, prepolymerized fillers, mixed oxides |
Venus Diamond Flow (VDF) | Heraeus Kulzer GmbH | 65% (w/w) 41% (v/v) |
20 nm–5 μm | Glass, SiO 2 , YbF 3 |
XFlow (XF) | Dentsply International | 60% (w/w) 38% (v/v) |
1.6 μm | Glass, SiO 2 |
2.2
PDMS template assisted microstructuring
Cut pieces of silicon (Si) wafers, with a size of 10 mm × 10 mm, and a thickness of 0.5 mm with three different surface structures created by photolithography (cubes, linear trapezoid structures, flat pyramids) and flat native Si as control were used as initial master pattern samples. The exact dimensions of the structures can be found in the supplementary part. Initially only the cube structures were used for testing the structurability of the different composites.
The structures were transferred from the Si masters to polydimethylsiloxane (PDMS) templates made of PDMS Sylgard 184 (Dow Corning Corporation, Midland, USA). The ratio between pre-polymer and curing agent was 10:1 wt./wt. This liquid was poured on the Si masters and subsequently cured for 24 h at 75 °C resulting in a flexible PDMS template. Flat PDMS templates cured on flat, unstructured Si were used for the creation of flat control samples.
Approximately 0.25 ml of the composite material was deposited on a glass slide. Subsequently, the composite was covered with the structured PDMS template (size 10 mm × 10 mm), and a slight pressure was applied to the template by hand. Afterwards, the composite was polymerized for 30 s by an Elipar FreeLight 2 LED light curing unit (LCU; 3M ESPE AG, Seefeld, Germany) by subsequently curing overlapping areas covered by the light guide of the LCU. This procedure was applied to different composites. The thickness of the so structured and cured composite samples was approximately 0.5 mm. The different steps of the PDMS template assisted microstructuring of the dental composites are illustrated schematically in Fig. 1 .
For microbial adhesion tests in the well plates, the composite samples were structured from both sides to avoid an influence of the unstructured bottom side of the samples on microbial adhesion results.
The surface structure of the microstructured composites was characterized using a Zeiss Auriga 60 scanning electron microscope (SEM; Zeiss AG, Oberkochen, Germany). The pattern reproduction at the composites surfaces was qualitatively judged by eye from the SEM micrographs.
2.3
Rheological characterization of dental composites
The Advanced Rheometric Expansion System (ARES, TA Instruments Inc., New Castle, USA) was used for the rheological characterization of the composites in this study. The temperature during all measurements was 25 °C. The resin composites were squeezed on the lower part of a parallel plates viscometer module (diameter 25 mm). The gap between the two plates was fixed to 1 mm. Strain sweep measurements were performed on each material to determine the maximum strain at which the resin still behaves like a structured fluid (solid-like). A strain value lower than this maximum strain was chosen individually for each composite. Therefore, the complex viscosity ( η *) of the different composites achieved from frequency sweeps (0.1 rad/s, 1 rad/s, 10 rad/s and 100 rad/s) are comparable.
Additionally, stress relaxation tests were used to investigate the flow behavior of the resin composites after instantaneous shear strain. To mimic the conditions during the preparation of the samples a pre-shear rate of 10 s −1 was used for 5 s before applying a strain of 10%. The resulting shear modulus was measured as a function of time.
2.4
Contact angle measurements on the dental composites
It is well known from the literature that the bacterial adhesion to a surface is affected by its wettability. Therefore, water contact angle measurements were carried out to determine the wettability of the microstructured composites and the unstructured control samples. The static contact angle was determined with the sessile drop method using a Drop Shape Analysis System DSA 10 (Krüss GmbH, Hamburg, Germany). For statistical analysis, ten measurements with deionized water were carried out on respectively 3 samples and averaged.
2.5
Microbial adhesion and biofilm formation test
Microbial adhesion and biofilm formation as functions of the dental composites surface microstructures was investigated using oral microorganisms originating from a test person (age 25) with a DMF-T-index (decayed-missed-filled-teeth) of 0. Plaque was sampled from the test person from each quadrant using sterile paper tips and incubated anaerobically in Schaedler nutrient solution (OXOID Deutschland GmbH, Wesel, Germany) for 24 h to induce microbial growth. Afterwards, the microbial suspension was adjusted with fresh nutrient solution to an optical density (OD570 nm) of 0.5 using a photospectrometer (Eppendorf PCP 6121, Eppendorf AG, Hamburg, Germany). Simultaneously, the composite specimens were, first, incubated in deionized water for 7d at 37 °C to remove free radicals left from the polymerization reaction. Afterwards, for pre-conditioning of the microstructured composite surfaces, saliva was collected from the test person after tooth brushing in the morning, centrifuged (Eppendorf 5415D, Eppendorf AG) for 5 min at 13,000 rpm (16,110 × g ) and the composite specimens were incubated for 1 h at 37 °C each in 500 μL of the supernatant. The pre-conditioned composite samples were rinsed twice in phosphate buffered saline (PBS) and incubated for 4 h with the microbial suspension while gently mixing using a plate shaker (Titramax 100 m, Heidolph North America, USA) at 150 rpm to reduce the effect of sedimentation of the microbial cells on adhesion.
To investigate the specific influence of the composite surface microstructures on bacterial adhesion and biofilm formation and to exclude interference from specific chemical surface properties of the different dental composite materials, adhesion and biofilm tests were performed only on the specimens prepared from the GF composite.
2.6
Biofilm analysis
The established biofilms were quantitatively characterized by estimation of the colony forming units (CFU) and scanning electron microscopy. Before analysis, all samples were carefully rinsed with PBS to remove non-adherent microorganisms.
For estimating the CFU numbers, composite specimens were placed each in 1 mL of PBS and microorganisms adherent on the samples’ surfaces were removed using a vortexer. 20 μL of each microbial suspension was, as the first step, diluted 1:10 in physiological saline solution. Afterwards, a dilution series was prepared with the highest dilution of 10 −6 . Each dilution step was incubated under anaerobic conditions for 48 h at 37 °C on Schaedler agar plates. The CFU were estimated by counting.
For SEM, composite samples with adherent microorganisms were incubated in glutaraldehyde solution (2%) for 30 min at room temperature followed by an incubation step with cacodylate buffer (0.1 M) for 10 min. After fixation, samples were washed for 10 min in PBS buffer solution and twice in deionized water. Samples were, then, dehydrated using an ascending ethanol series from 30% to 96%. Dehydrated samples were air dried for 24 h and sputter coated with gold (S150B, Edwards Ltd, Crawley, UK). For imaging, the AURIGA 60 SEM was operated at 3.5 kV and a working distance of about 3 mm. In addition to CFU counting, the numbers of adherent microbial cells were additionally estimated based on the SEM images by direct counting, as well.
2.7
Statistical analysis
The data were analyzed for statistically significant differences among groups using a one-way ANOVA (analysis of variance) based on a Tukey post hoc comparison (Sigmaplot 12.0, Systat Software Inc., Chicago, USA). The level of significance was p ≤ 0.05. All data are given as means ± standard deviations.
2
Materials and methods
2.1
Dental composites
Six different composites were first tested for their structurability by polydimethylsiloxane (PDMS) templates: the nanohybride composites CLEARFIL MAJESTY Posterior (CMP; Kuraray Europe GmbH, Frankfurt, Germany), Grandio Flow (GF; Voco GmbH, Cuxhaven, Germany), Premise (P; Kerr Corporation, Orange, USA), Tetric EvoFlow (TEF; Ivoclar Vivadent AG, Schaan, Liechtenstein), Venus Diamond Flow (VDF; Heraeus Kulzer GmbH, Hanau, Germany) and the microhybrid composite XFlow (XF; Dentsply International, York, USA). All composites used in the current study were of the shade A2. The properties of the composites, as obtained from manufacturers data sheets, are listed in Table 1 .
Composite | Company | Filler content | Filler size | Filler material |
---|---|---|---|---|
CLEARFIL MAJESTY Posterior (CMP) | Kuraray Europe GmbH | 92% (w/w) 82% (v/v) |
20 nm–1.5 μm | Aluminum oxide, glass |
Grandio Flow (GF) | Voco GmbH | 80% (w/w) 65.6% (v/v) |
40 nm–1 μm | Glass, SiO 2 |
Premise (P) | Kerr Corporation | 84% (w/w) 70% (v/v) |
20 nm/0.4 μm/30 μm | Prepolymerized fillers, glass, SiO 2 |
Tetric EvoFlow (TEF) | Ivoclar Vivadent AG | 57.5% (w/w) 30.7% (v/v) |
550 nm | Glass, ytterbium trifluoride, prepolymerized fillers, mixed oxides |
Venus Diamond Flow (VDF) | Heraeus Kulzer GmbH | 65% (w/w) 41% (v/v) |
20 nm–5 μm | Glass, SiO 2 , YbF 3 |
XFlow (XF) | Dentsply International | 60% (w/w) 38% (v/v) |
1.6 μm | Glass, SiO 2 |
2.2
PDMS template assisted microstructuring
Cut pieces of silicon (Si) wafers, with a size of 10 mm × 10 mm, and a thickness of 0.5 mm with three different surface structures created by photolithography (cubes, linear trapezoid structures, flat pyramids) and flat native Si as control were used as initial master pattern samples. The exact dimensions of the structures can be found in the supplementary part. Initially only the cube structures were used for testing the structurability of the different composites.
The structures were transferred from the Si masters to polydimethylsiloxane (PDMS) templates made of PDMS Sylgard 184 (Dow Corning Corporation, Midland, USA). The ratio between pre-polymer and curing agent was 10:1 wt./wt. This liquid was poured on the Si masters and subsequently cured for 24 h at 75 °C resulting in a flexible PDMS template. Flat PDMS templates cured on flat, unstructured Si were used for the creation of flat control samples.
Approximately 0.25 ml of the composite material was deposited on a glass slide. Subsequently, the composite was covered with the structured PDMS template (size 10 mm × 10 mm), and a slight pressure was applied to the template by hand. Afterwards, the composite was polymerized for 30 s by an Elipar FreeLight 2 LED light curing unit (LCU; 3M ESPE AG, Seefeld, Germany) by subsequently curing overlapping areas covered by the light guide of the LCU. This procedure was applied to different composites. The thickness of the so structured and cured composite samples was approximately 0.5 mm. The different steps of the PDMS template assisted microstructuring of the dental composites are illustrated schematically in Fig. 1 .
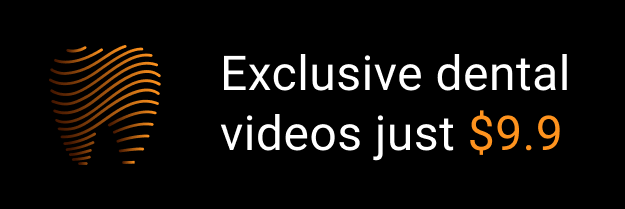