Abstract
Objectives
To synthesize and characterize machinable, bioactive glass-ceramics (GCs) suitable for dental implant applications.
Methods
A glass in the SiO 2 –Al 2 O 3 –CaO–CaF 2 –K 2 O–B 2 O 3 –La 2 O 3 system was synthesized by wet chemical methods, followed by calcination, melting and quenching. Crystallization kinetics were determined by differential thermal analysis (DTA). GC discs were produced by cold pressing of the glass powder and sintered using schedules determined by DTA. The crystalline phases and microstructure of GC samples were characterized by X-ray diffraction (XRD) and scanning electron microscopy (SEM), respectively. Dynamic Young’s modulus ( E ), true hardness ( H o ), fracture toughness ( K IC ) and brittleness index (BI) were evaluated. Bioactivity was studied by examining the formation of hydroxyapatite (HA) on the GC surfaces after soaking in simulated body fluid (SBF). Attachment and proliferation of MC3T3-E1 osteoblastic cells were assessed in vitro .
Results
Miserite [KCa 5 (Si 2 O 7 )(Si 6 O 15 )(OH)F] was the main crystalline phase of the GC with additional secondary phases. Microstructural studies revealed interlocking lath-like crystalline morphology. E , H o , and K IC values for the GCs were 96 ± 3 GPa, 5.27 ± 0.26 GPa and 4.77 ± 0.27 MPa m 0.5 , respectively. The BI was found to be 1.11 ± 0.05 μm −0.5 , indicating outstanding machinability. An HA surface layer was formed on the GC surfaces when soaked in SBF, indicating potential bioactivity. MC3T3-E1 cells exhibited attachment, spreading and proliferation on GC surfaces, demonstrating excellent biocompatibility.
Significance
We present a novel approach for the synthesis of miserite GC with the physical and biological properties required for non-metallic dental implant applications.
1
Introduction
Dental implantology has become a predictable treatment for patients with missing teeth. Titanium (Ti) is the material most widely used for dental implants due to its superior mechanical properties, biocompatibility and resistance to corrosion. However, a long term clinical study revealed biological and technical complications with Ti dental implants . Hypersensitivity and allergic reactions to Ti dental implants occur, although these reactions are under-reported, and the etiological factors of implant failure are poorly understood . In addition, the esthetic outcomes of Ti dental implants can be compromised in cases of gingival tissue recession, especially when replacing anterior teeth .
Ceramics were introduced as potential materials for non-metallic dental implants. Among a variety of choices, the material currently used is yttria-stabilized tetragonal zirconia (Y-TZP) . Advantages of zirconia include tooth-like color, good mechanical properties, biocompatibility, low affinity for plaque, and osseointegration comparable with Ti dental implants . However, zirconia undergoes accelerated aging under stress in the presence of moisture, leading to surface deterioration, nucleation and propagation of microcracks that can result in spontaneous catastrophic failure .
Bioactivity is defined as the ability of biomaterials to promote the formation of a crystalline HA layer from physiological fluid . In implantology, there is considerable interest in bioactive materials to establish strong chemical bonding between the implant and bone, as well as to accelerate implant anchorage by inducing an HA layer on the implant surface . Ti and zirconia are considered bioinert; therefore, to improve their osseointegration, different bioactive coatings such as plasma-sprayed HA have been developed . However, such coatings do not degrade uniformly, giving rise to weak points at the bone-coating-implant interfaces .
GCs are produced by controlled nucleation and crystallization of glasses, and their properties can be tailored for specific applications . There is great interest in GCs that possess appropriate physical, mechanical and biological properties for biomedical applications. These properties can be improved and modified by varying the base glass composition or heat treatment conditions . Currently, several bioactive GCs are used for orthopedic applications, including vertebral prostheses, middle ear devices and bone defect fillers . Miserite is a triclinic potassium calcium silicate mineral [KCa 5 (Si 2 O 7 )(Si 6 O 15 )(OH)F], which has an anisotropic, lath or rod-like crystal shape . Miserite was introduced in 1999 as the predominant crystal phase in multi-phase GCs that showed high bending strength (175 MPa) and high fracture toughness (>3.5 MPa m 0.5 ) . It is anticipated that miserite GC is biocompatible and bioactive; however, it has not been evaluated previously as a material for dental implant applications .
Machinable biomaterials allow dentists to design and fabricate customized dental restorations with a high level of accuracy and precision through CAD-CAM technology . Introducing CAD-CAM technology in dental implantology offers dental clinicians a variety of treatment plans, with customized implants for each individual patient. The objective of this study was to synthesize and characterize bioactive and machinable miserite GCs, with physical, mechanical and biological properties appropriate for dental implant materials.
2
Materials and methods
2.1
Glass synthesis
Preliminary unpublished work conducted in our laboratory involved the synthesis of a range of multicomponent glass compositions in the system (SiO 2 –Al 2 O 3 –CaO–CaF 2 –K 2 O–B 2 O 3 –La 2 O 3 ) by wet chemical synthesis using sol–gel chemistry followed by spay-drying, calcination and melting. The glass composition (wt%) that was selected for the present study was 57.8 SiO 2 , 0.5 Al 2 O 3 , 18.4 CaO, 12.6 CaF 2 , 6.8 K 2 O, 1.0 B 2 O 3 and 2.9 La 2 O 3 . The rationale for using this composition was based on optimizing mechanical properties and machinability. The reagents used were of high purity and soluble in water to insure the homogeneity of the final product.
The glass was synthesized through the following four steps. (1) Hydrolysis and polycondensation of metal alkoxides by mixing appropriate amounts of Si(C 2 H 5 O) 4 , Al(NO 3 ) 3 9H 2 O, Ca(NO 3 ) 2 4H 2 O, CaF 2 , KNO 3 , H 3 BO 3 and La(NO 3 ) 3 6H 2 O in aqueous solution. (2) Spray-drying with feed flow rate of 10 ml/min, inlet air temperature of 160 °C and outlet air temperature of 80 °C using a 190 mini spray-dryer (BÜCHI, Switzerland), yielding a spray-dried powder. (3) Calcination of the powder by heating incrementally at 200 °C, 500 °C and 700 °C for 2 h each in a Ney 650 vacuum oven (Ney-Barkmeyer, USA). (4) Melting of the calcined powder in an uncovered platinum crucible at 1150 °C for 3 h and 1250 °C for 1 h in a high temperature furnace (Thermolyne Corporation, USA), followed by quenching in iced water to obtain transparent glass frits.
2.2
Crystallization kinetics
Transition and crystallization temperatures of experimental glasses were determined by DTA using a SDT Q600 V20.5 Build 15 system (TA Instruments, USA) under air atmosphere at five different heating rates (10–50 °C/min), starting from room temperature up to 1200 °C. The average sample weight was 20 mg and an equivalent weight of α-Al 2 O 3 was used as a reference. The activation energy (Δ E ) was calculated from the plot of ln (( T p ) 2 /) vs 1000/ T p using the Kissinger equation , where T p is the exothermic peak as determined from the DTA spectra and is the heating rate (°C/s). The Avrami exponent ( n A ) was determined from the DTA exothermic peak using the Augis-Bennett equation .
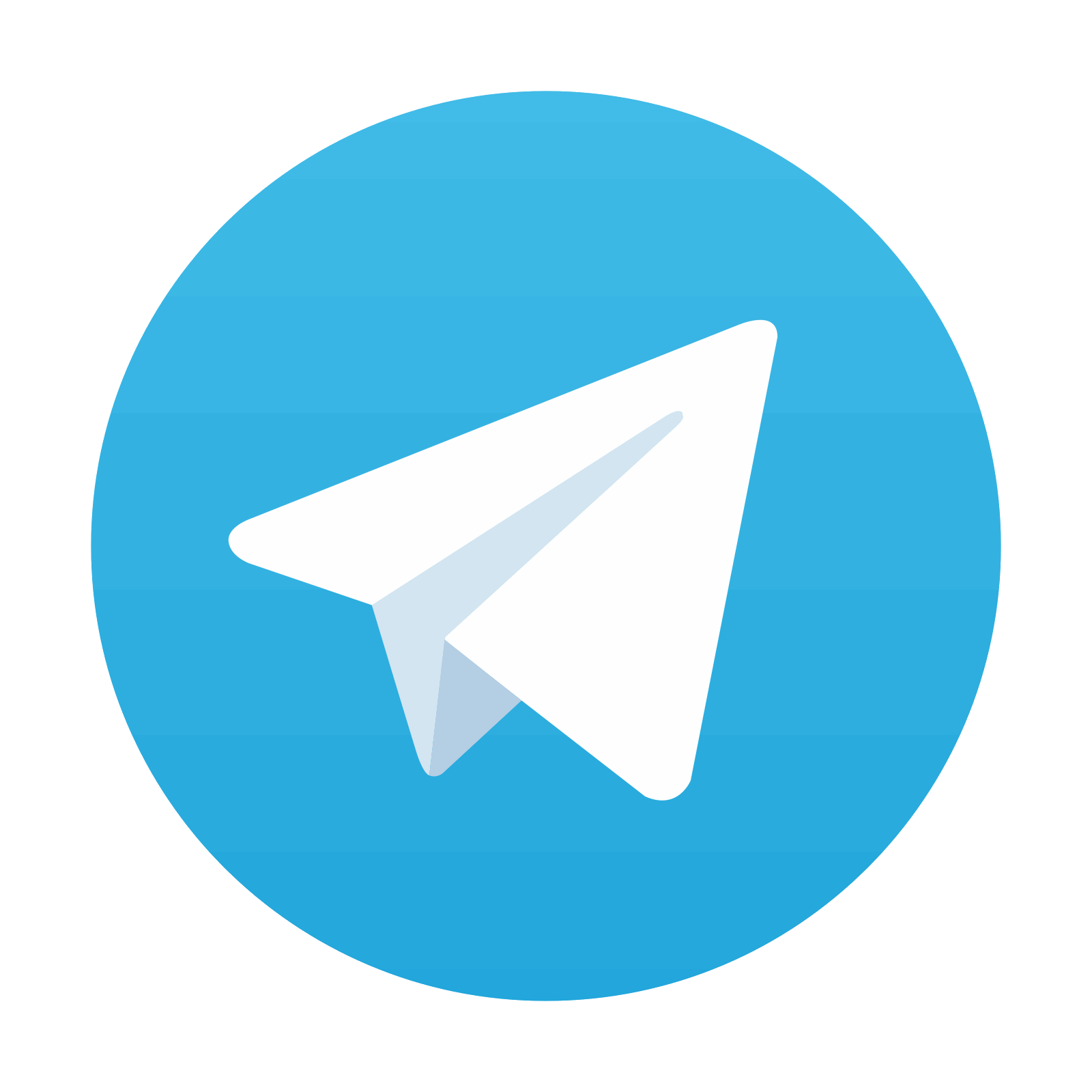
Stay updated, free dental videos. Join our Telegram channel

VIDEdental - Online dental courses
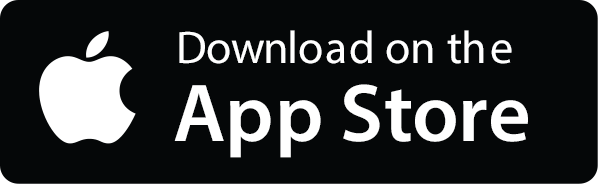
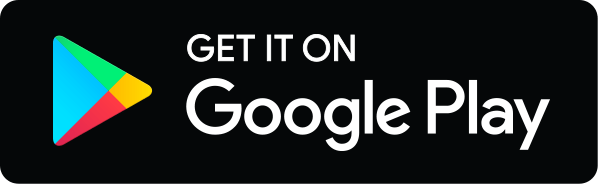