Abstract
Objectives
To synthesize a glass–ceramic (GC) that is suitable for non-metallic one-piece dental implant application.
Methods
Three glasses in a SiO 2 –Al 2 O 3 –CaO–CaF 2 –K 2 O–B 2 O 3 –P 2 O 5 –CeO 2 –Y 2 O 3 system were produced by wet chemistry. Differential thermal analysis (DTA) was carried out to determine the glass crystallization kinetic parameters and the heating schedules that were used for sintering of GCs. Crystalline phases and crystal morphologies were studied by X-ray diffraction (XRD) and scanning electron microscopy (SEM), respectively. Mechanical properties of the GCs were determined by ultrasonic and indentation tests and its machinability were evaluated. Chemical durability was carried out according to ISO 6872, whereas testing chemical degradation in tris buffered solution was executed according to ISO 10993-14.
Results
XRD of the GC specimens showed that wollastonite was the main crystalline with other secondary phases; GC2 had cristobalite as an additional phase. SEM of the GCs revealed dense acicular interlocking crystals. Young’s modulus of elasticity ( E ), true hardness ( H o ) and fracture toughness ( K IC ) of the GCs were 89–100 GPa, 4.85–5.17 GPa and 4.62–5.58 MPa m 0.5 , respectively. All GCs were demonstrated excellent machinability. The GCs exhibited various chemical durability and degradation rates. K IC values of the GCs following chemical durability testing were not significantly different from those of the original materials ( p > 0.05). GC2 exhibited significantly higher K IC value compared with GC1 and GC3 ( p < 0.05) and its chemical durability satisfied ISO 6872 specification for dental ceramics.
Significance
Wollastonite–cristobalite GC can be considered as a promising material for one-piece dental implant applications due to its strength, machinability and chemical durability.
1
Introduction
Glass–ceramics are polycrystalline materials with an inorganic–inorganic microstructure that were prepared from base glass by controlled crystallization. This was achieved by subjecting glasses to regulated heat treatment, which resulted in the nucleation and growth of one or more crystal phases within the glass. GCs have diverse physical, chemical, mechanical, optical and biological properties that can be modified with glass composition and heat treatment conditions . As a consequence of the continuous need of the general public as well as the dental professionals to eliminate dental metal-based products, there is a trend toward GCs and ceramic-based materials in the biomedical field . Yttrium-stabilized tetragonal zirconia polycrystalline has been used extensively as a material of choice for ball heads in total hip replacement since the 1980s, and it became commercially available as non-metallic dental implants in the 2000s, owing to its strength, fracture resistance and appropriate optical properties . However, zirconia undergoes aging through low-temperature degradation phenomenon that is moisture/water related and unfavorably affects its physical properties. In 2000, several hundreds of hip prosthesis failed over a short period of time. These failures were ascribed to an accelerated aging of the zirconia femoral head in particular batches . Additionally, literatures showed that zirconia is correlated with two types of radiation impurities, alpha and gamma. Alpha radiation has been observed in a significant amounts and it could harm soft and hard tissues’ cell due to their high ionization .
The wollastonites (CaSiO 3 ) are white glassy silicate minerals that occur as masses or tabular crystals of metamorphosed limestone. A silica chain GC that contains crystalline apatite and β-wollastonite (AW) was introduced in an MgO–CaO–SiO 2 –P 2 O 5 glassy matrix and it showed an excellent bioactivity, biocompatibility, machinability and adequate mechanical properties such as Young’s modulus (117 GPa), compressive strength (1080 MPa), and bending strength (215 MPa) . AW has been used for orthopedic applications—artificial vertebrae, intervertebral discs and iliac crest prostheses . Kokubo proved that the mechanical strength of the AW was significantly unaffected in simulated body fluid at 36.5 °C and presumed that it can withstand a bending stress of 65 MPa in the human body environment for over 10 years . Yet, the fracture toughness of the AW had relatively low values (2–2.5 MPa m 0.5 ); therefore it’s limited to non-load bearing applications; and we note that, a custom AW prostheses production by conventional lost wax casting can be difficult as a result of the surface crystallization . However, because of its excellent bioactivity, AW was used as a coating on different substrates such as titanium alloys or as a composite scaffold by incorporation of AW with other materials .
Fracture toughness ( K IC ) measures the resistance of a material to cracks propagation and the ability to prevent the initiation of catastrophic fracture. Indentation test has been considered as an accurate procedure to measure the fracture toughness for brittle materials, like ceramics and GCs. The indentation method has the advantages of simplicity and economy; where only small specimen area is needed, hence the technique is suited for comparative evaluation .
Chemical durability affects the clinical performance of dental materials. With a wide range of pH and temperature, dental materials should resist chemical degradation and dissolution. Chemical degradation of ceramic dental materials could lead to structure’s weakening and surface roughness as a result of surface–ion exchange. This phenomenon leads to increase plaque attachment onto the biomaterials and intensify abrasion potential against opposing natural teeth and other restorative materials .
The brittle behavior of the GCs makes them sensitive to milling and machining; therefore, the development of machinable GCs for dental implant applications is considered a significant progress. A machinable dental implant material can be introduced to CAD–CAM technology and customized implants for different clinical cases can be fabricated, in addition to the possibility of the modification and adjusting of the implant at the time of surgical insertion. Machinable GCs can be used as one-piece dental implants where the upper part can be prepared to produce the abutment unit and get rid of multiple component implants i.e. fixture, screw and abutment.
The objectives of this study were to synthesize machinable wollastonite GCs with mechanical properties suitable for dental implant applications, to assess their chemical durability using acetic acid and tris buffered solution at different time points and evaluate their fracture toughness following chemical degradation testing.
2
Material and methods
2.1
Glass synthesis
Transparent glass frits were synthesized by wet chemical methods through four steps. In the beginning, the desired glass compositions in wt% ( Table 1 ) were prepared by mixing batch ingredients {Si(C 2 H 5 O) 4 , Al(NO 3 ) 3 ·9H 2 O, Ca(NO 3 ) 2 ·4H 2 O, CaF 2 , KNO 3 , H 3 BO 3 , Ce(NO 3 ) 3 ·6H 2 O and Y(NO 3 )·6H 2 O} in aqueous solution, and kept stirred overnight to commence hydrolysis and polycondensation of metal alkoxides. This was followed by spray-drying of the solutions at a feed flow rate of 10 ml/min, inlet air temperature of 160 °C and outlet air temperature of 80 °C using a 190 mini spray-dryer (BÜCHI, Switzerland). The spray-dried powders were calcined by means of a Ney 650 vacuum oven (Ney-Barkmeyer, USA) through sequential heating schedules at 200, 500 and 700 °C for 2 h. Finally, the calcined powders were melted in an uncovered platinum crucible at 1350 °C for 3 h in a high temperature furnace (Thermolyne Corporation, USA), followed by quenching in iced-water to obtain the glass frits.
Glass component | Composition (wt%) | ||
---|---|---|---|
G1 | G2 | G3 | |
SiO 2 | 55 | 59 | 50 |
Al 2 O 3 | 2 | 1 | 2 |
CaO | 17 | 15 | 20 |
CaF 2 | 12 | 12 | 12 |
K 2 O | 5 | 5 | 7 |
B 2 O 3 | 3 | 3 | 3 |
P 2 O 5 | 1.5 | 2 | 3 |
CeO 2 | 1.5 | – | – |
Y 2 O 3 | 3 | 3 | 3 |
2.2
Differential thermal analysis
Crystallization kinetic parameters of the glasses (G) were determined using SDT Q600 V20.5 Build 15 (TA Instruments, USA) at different five heating rates (10–50 °C/min), starting from room temperature up to 1200 °C under air atmosphere; for each heating rate, three-independent runs were carried out ( n = 3). The average sample weight was 20 mg and an equivalent weight of α-Al 2 O 3 was used as a reference. The activation energy ( ΔE ) was calculated from the plot of ln ( T p ) 2 / Ø vs. 1000/ T p using the Kissinger equation where T p is the exothermic peak (°C) as determined from the DTA spectra and Ø is the heating rate (°C/s). The Avrami exponent ( n A ) was calculated using the Augis–Bennett equation from the full width at the half maximum of the exothermic peak at 20 °C/min. n A is related to the directionality of crystal growth, lower values (≈1) of n A reflected surface crystallization, whereas n A values above 1.5 indicated bulk crystallization .
2.3
Glass–ceramics preparation
Glass powders were mixed with 4% polyethylene glycol as a binder and ball-milled using a planetary ball mill, Pulverisette 7 (Laval Lab, USA). Cylindrical ingots (6 mm × 10 mm) and disk specimens (11 mm × 1 mm) of the glass powders were produced by cold pressing using a Carver laboratory cold press (Carver, USA) in stainless steel dies at 40 MPa. Pressed glass specimens were heated for 1 h at the glass transition temperature that was obtained from DTA spectra for the nuclei formation. Afterward the temperature was subsequently raised to the crystallization temperatures determined from the DTA exothermic peaks and held for 4 h, Table 2 .
Samples | Heating rate (°C/min) | Heat treatment | |
---|---|---|---|
Temperature (°C) | Holding time (h) | ||
GC1 | 20 | 700 | 1 |
950 | 4 | ||
GC2 | 10 | 750 | 1 |
1000 | 4 | ||
GC3 | 10 | 700 | 1 |
950 | 4 |
2.4
X-ray diffraction
Crystalline phases of the GCs were analyzed by XRD using a Rotaflex RTP 300 RC (Rigaku Co, Japan) operating on Co K α radiation at 45 kV and 160 mA. Spectra were collected in the 2 θ range between 2° and 82°, with 0.05° step and 10°/min scan speed. Bragg’s law was used to calculate 2 θ for equivalent Cu K α radiation .
2.5
Microstructure
Prior to SEM analysis, samples were mounted on aluminum stubs with silver paint and then coated with 3 nm osmium metal (Filgen OPC 80T). The fracture surfaces of the glasses and GCs were examined using LEO 1540XB FIB/SEM (Zeiss, Germany) equipped with an energy dispersive X-ray spectroscopy (EDX) system.
2.6
Dynamic elastic constants of the GCs
The dynamic elastic properties of the synthesized GCs and yttria-stabilized zirconia (YZ) ceramic control (Vita Zahnfabrik, Germany) were evaluated by ultrasonic method . The density of each specimen ( ρ ) was determined using the Archimedes method in deionized water at room temperature. The dynamic elastic moduli and Poisson’s ratios ( n = 3) were calculated from the velocities of the longitudinal ( V L ) and shear ( V S ) waves which were determined using lithium niobate crystals that were used for transmitting and receiving waves at 10 MHz resonant frequencies The equations used for the calculation are reported elsewhere .
2.7
True hardness and fracture toughness evaluation
The true hardness ( H o ) and the fracture toughness ( K IC ) of the GCs and YZ specimens were determined using a Buehler Micromet 5114 Knoop and Vickers micro hardness indenter, (Buehler, USA), respectively. The specimens were embedded in resin and polished ( n = 3). To calculate the H o , a series of Knoop indentations were performed on each specimen at six consecutive loads (0.49–9.9 N). The average diagonal lengths of the Knoop indentations were measured using Buehler OmniMet MH T7.2 Rev.2 optical microscope equipped with a digital camera and computer software (Buehler, USA). Indentation lengths were plotted vs. the square root of the different load values, and H o was calculated from the slope of the linear regression line . K IC of the GCs and YZ were determined by measuring the diagonals of the Vickers indentation and the crack lengths using the Lankford equation . The average K IC value was calculated from 45 indentations performed on three specimens at a load of 9.8 N.
2.8
Chemical durability
The ISO standard 6782 was used for assessing the chemical durability of the GCs and YZ. Triplicate samples were washed in distilled water in a digital ultrasonic bath (Eumax, China) and dried in a Temp Master “A” furnace (JELRUS, USA) at 150 ± 5 °C for 4 h. The weight of the samples and the total surface area were determined to the nearest 0.1 mg and 0.1 cm 2 , respectively. Specimens were soaked in 20 ml of pre-heated (80 °C) 4% acetic acid (AC) solution in glass bottles. The bottles were placed in a vacuum oven (VWR, USA) at 80 °C for 16 h. Then the specimens were washed with distilled water in the ultrasonic bath, dried at 150 ± 5 °C until a constant weight was reached. The chemical durability was analyzed by measuring of the specimens weight loss/unit area (wt/A; μg/cm 2 ). Later, to assess the effect of the chemical dissolution on the mechanical properties of the materials, the K IC were re-measured for chemically tested specimens.
2.9
Degradation testing
The degradation testing was performed according to ISO 10993-14 . The test is based on a buffered solution that simulates the body’s normal pH level. Tris–HCl buffered solution (TBS) was prepared by dissolving tris (hydroxymethyl) aminomethane in water; the pH of the solution was adjusted to 7.4 ± 0.1 with an appropriate amount of 1 mol/l HCl acid at 37 °C. Triplicate specimens were washed in a digital ultrasonic bath (Eumax, China) and dried at 150 ± 5 °C in a Temp Master “A” furnace (JELRUS, USA). Samples’ weights were measured, and then the specimens were soaked in freshly prepared TBS in polypropylene containers. The bottles were placed in a MaxQ 5000 circular agitated controlled-temperature chamber (Thermo Scientific, USA) at 37 °C for different timelines (1, 3, 7, 30, 60, 90, and 120 days). At the predetermined time point the specimens were washed with distilled water in the ultrasonic bath, dried to constant mass and weighed. The degradation of each sample was determined by calculating the percentage weight loss. In a similar manner as described above, the K IC for the specimens those were soaked for 120 days in TBS were re-measured.
2.10
Machinability
Qualitative evaluation of the machinability of the GCs was conducted through drilling holes, grooves, bevels as well as preparing shoulder finish lines on GCs’ ingots and disks of different thicknesses. Additionally, quantitative assessment was done by calculating brittleness index (BI), which is the ratio of the hardness to the fracture toughness. The lower the brittleness index, the higher the machinability .
2.11
Statistical analyses
One-way analysis of variance (ANOVA) followed by Tukey’s multiple comparisons test at a significance value of p < 0.05 was used to analyze differences among groups. Nonlinear regression analysis was used to evaluate the degradation rates at different timeline. The K IC values for the chemically tested specimens at 120 days were analyzed with simple main effect analysis and Tukey’s multiple comparisons test, p < 0.05.
2
Material and methods
2.1
Glass synthesis
Transparent glass frits were synthesized by wet chemical methods through four steps. In the beginning, the desired glass compositions in wt% ( Table 1 ) were prepared by mixing batch ingredients {Si(C 2 H 5 O) 4 , Al(NO 3 ) 3 ·9H 2 O, Ca(NO 3 ) 2 ·4H 2 O, CaF 2 , KNO 3 , H 3 BO 3 , Ce(NO 3 ) 3 ·6H 2 O and Y(NO 3 )·6H 2 O} in aqueous solution, and kept stirred overnight to commence hydrolysis and polycondensation of metal alkoxides. This was followed by spray-drying of the solutions at a feed flow rate of 10 ml/min, inlet air temperature of 160 °C and outlet air temperature of 80 °C using a 190 mini spray-dryer (BÜCHI, Switzerland). The spray-dried powders were calcined by means of a Ney 650 vacuum oven (Ney-Barkmeyer, USA) through sequential heating schedules at 200, 500 and 700 °C for 2 h. Finally, the calcined powders were melted in an uncovered platinum crucible at 1350 °C for 3 h in a high temperature furnace (Thermolyne Corporation, USA), followed by quenching in iced-water to obtain the glass frits.
Glass component | Composition (wt%) | ||
---|---|---|---|
G1 | G2 | G3 | |
SiO 2 | 55 | 59 | 50 |
Al 2 O 3 | 2 | 1 | 2 |
CaO | 17 | 15 | 20 |
CaF 2 | 12 | 12 | 12 |
K 2 O | 5 | 5 | 7 |
B 2 O 3 | 3 | 3 | 3 |
P 2 O 5 | 1.5 | 2 | 3 |
CeO 2 | 1.5 | – | – |
Y 2 O 3 | 3 | 3 | 3 |
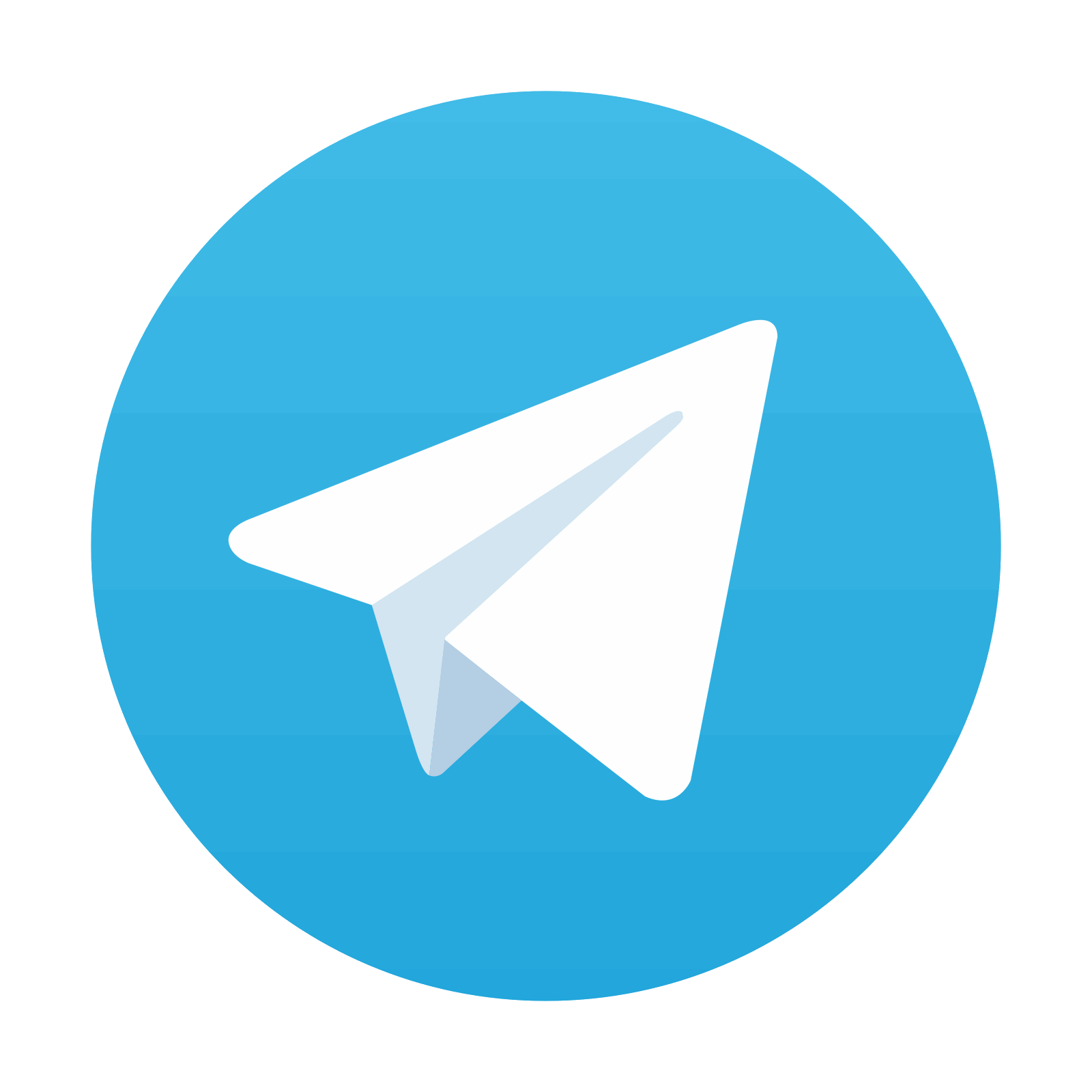
Stay updated, free dental videos. Join our Telegram channel

VIDEdental - Online dental courses
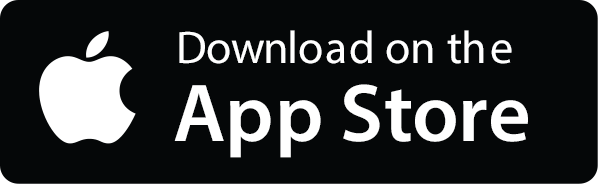
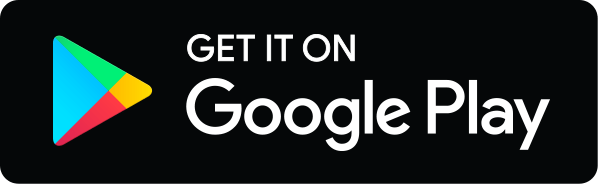
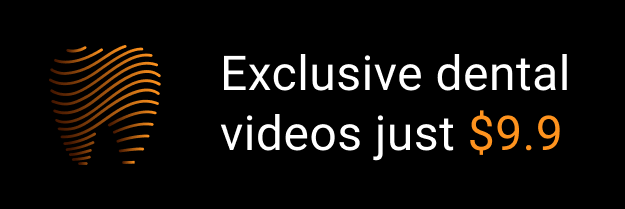