Abstract
Objective
The purpose of this study was to reveal the effectiveness of non-thermal atmospheric plasma brush in surface wettability and modification of four dental substrates.
Methods
Specimens of dental substrates including dentin, enamel, and two composites Filtek Z250, Filtek LS Silorane were prepared (∼2 mm thick, ∼10 mm diameter). The prepared surfaces were treated for 5–45 s with a non-thermal atmospheric plasma brush working at temperatures from 36 to 38 °C. The plasma-treatment effects on these surfaces were studied with contact-angle measurement, X-ray photoemission spectroscopy (XPS) and scanning electron microscopy (SEM).
Results
The non-thermal atmospheric argon plasma brush was very efficient in improving the surface hydrophilicity of four substrates studied. The results indicated that water contact angle values decreased considerably after only 5 s plasma treatment of all these substrates. After 30 s treatment, the values were further reduced to <5°, which was close to a value for super hydrophilic surfaces. XPS analysis indicated that the percent of elements associated with mineral in dentin/enamel or fillers in the composites increased. In addition, the percent of carbon (%C) decreased while %O increased for all four substrates. As a result, the O/C ratio increased dramatically, suggesting that new oxygen-containing polar moieties were formed on the surfaces after plasma treatment. SEM surface images indicated that no significant morphology change was induced on these dental substrates after exposure to plasmas.
Significance
Without affecting the bulk properties, a super-hydrophilic surface could be easily achieved by the plasma brush treatment regardless of original hydrophilicity/hydrophobicity of dental substrates tested.
1
Introduction
In dentistry, most joints consist of two adherends (the term adherend refers to either an initial substrate or substrate being bonded to). For most of dental joints, one set of adherends could be any dental substrates such as enamel, dentin, cement, residual amalgam or composite from a previous restoration, or an implant; the other set of adherends could be restorative ones such as composite, amalgam, or ceramics. Generally, good adhesion is critical in effectively creating these dental joints between the substrates.
Good dental adhesion entails a supreme contact between the adhesive and the adherend surface . To establish the adhesion, the adhesive material must spread impulsively across the entire adherend surface, or optimal wettability of the substrate is necessitated with reference to that adhesive . However, the adhesive is not always well-matched to the wettability of the certain adherend surface. For example, a hydrophobic material generally does not provide intimate contact with a hydrophilic substrate. Enamel or wet dentin surfaces are commonly hydrophilic while most composites are comparatively hydrophobic. Offering satisfactory wettability for both of the distinct substrates certainly poses a big dilemma for dental adhesives. Surface modification or engineering for wettability enhancement is needed to improve adhesion between these substrates. For example, in an etch-and-rinse adhesive system, phosphoric acid is usually employed to improve the wettability, elevate the surface free energy, and increase the roughness of the dentin or enamel surface . The acidic primers play a similar role in a self-etching system . Other efforts have also been made to improve the dental surface wettability, such as application of the HEMA primer , irradiation of the laser , and treatment of sodium hypochlorite .
In the field of surface modification/engineering, many methods have been employed to alter the substrate to improve the surfaces properties such as adhesive ability, wettability, biocompatibility, and permeability . These conventional methods include mechanical abrasion, wet chemical treatment, exposure to flame and heating in oxidative environment. Although they have been widely used for years, there are always some adverse factors. For example, the abrasion method may greatly alter the surface morphology, or the wet chemical treatment may require a complicated procedure and leave a large amount of harmful wastes. Plasma surface treatment/modification has turned out to be a well-accepted method since it offers superior surface property enhancement than other chemical methods. Compared to these methods, the newly developed plasma surface engineering is “clean” and “effective”, while the bulk properties of the materials can be un-altered or well-maintained after the plasma treatment.
Plasmas are considered as “the 4th state” of matter and occur naturally in the sun, star, auroras and lightning bolts, containing a large amount of highly reactive species including ions, electrons, free radicals and electronically excited neutrals. Once being exposed to plasmas, the surface properties of the substrates would be modified as a result of the “bombing” of these excited species. The temperature of the plasmas can reach as high as 10,000 K (so called “thermal plasmas”), while that of “non-thermal plasmas” can be as low as 300 K . Non-thermal gas plasmas are partially ionized gases that contain the above reactive particles, while the gas phase remains near room temperature. Depending on the plasma chemistry or gas composition, these highly reactive plasma species react with, clean, and etch surface materials, or otherwise modify the surface of plastic, metal, or ceramic materials to improve their bonding capabilities or achieve totally new surface properties . This non-thermal feature has led to enormous successes of plasma techniques in surface engineering and modification of solid state materials, especially in plasma cleaning, plasma etching, surface engineering for adhesion enhancement, and biomaterial development .
Non-thermal atmospheric plasma technologies developed and improved over the past decade make it possible to investigate their applications for in vivo medical treatment. Because the gas phase can be controlled at or close to room temperature, plasma treatment of a biological system is not due to heat but the controllable plasma reactive species. The patented non-thermal atmospheric plasma brush, developed in our plasma research center, can be ignited and sustained in an ambient atmosphere, at very low power consumption (several watts), with temperatures close to that of the human body. Previous studies indicate that this brush was highly effective in oral bacterial disinfection , interfacial bonding improvement of composite restorations , and inducing dental adhesive polymerization . In this study, the surfaces of human dentin, enamel, as well as two commercial dental composites were modified to alter wettability by the non-thermal argon plasma brush with different parameters. Good wettability of a dental surface is very important in adhesion improvement of dental restorations. With optimal wettability, the adhesives can easily spread on the dental substrates, enhance adhesion. Contact angle measurements, X-ray photoelectron spectroscopy (XPS or ESCA), and scanning electron microscopy (SEM) were employed to characterize the plasma-modified surfaces. The null hypothesis tested was that the wettability (reflected in contact angles) and surface chemistry of all four substrates would not be changed after short period (<1 min) plasma treatment.
2
Materials and methods
2.1
Specimen preparation
Extracted non-carious human third molars were collected after the patients’ informed consent was obtained under a protocol approved by the University of Missouri-Kansas City adult health sciences institutional review board. The teeth were stored in 0.9% (w/v) phosphate buffered saline (PBS) containing 0.002% sodium azide at 4 °C, and the storage period was less than 1 month before use. The roots of molars were removed to 2–3 mm below the cemento-enamel junction (CEJ) using a water-cooled low-speed diamond saw (Isomet, Buehler Ltd., Lake Bluff, IL, USA). The teeth were then adhered to aluminum stubs using a cyanoacrylate adhesive (Zapit, Dental Ventures of America, Corona, CA). To make a dentin disk, the occlusal one-third to one-half was removed using the same water-cooled diamond saw until all enamel was removed from the resulting dentin surface (as determined by reflective light microscopy). The second cut was made perpendicular to the long axis to produce a slice with a thickness of ∼2.0 mm and a diameter of ∼10 mm. To make an enamel disk, two similar cuts were also performed; but for the first cut, the occlusal one-fourth to one-fifth was removed to make sure that only enamel was shown on the resulting surface. The dentin and enamel discs (∼10 mm in diameter) were polished with 600-grit SiC sandpaper (Buehler Ltd.) under water for 30 s, and then washed using ultrasonic cleaning for 5 min in deionized water to remove any possible debris. These discs were stored in ambient atmosphere (temperature ≈20 °C, relative humidity ≈15%) for 24 h to be naturally dried.
Two different commercial dental composites were employed in this study. The Filtek™ Z250 (Z250) was BIS-GMA based, and the Filtek™ LS (LS) was silorane-based (both were from 3M ESPE Dental Products, St. Paul, MN, USA). The chemical composition of these two composites is shown in Table 1 . Each composite was injected into a polytetrafluoroethylene mold of 10 mm in diameter and 2 mm in thickness, covered with a Mylar film (Fisher Scientific, Pittsburg, PA, USA) and light cured by a conventional dental light polymerization unit (Spectrum Light, DENTSPLY, Milford, DE, USA) at an output power of 550 mW/cm 2 for a period of time according to its instructions (Z250: 20 s; LS: 40 s). After curing, the mold and the film were removed. The specimens were polished with 600-grit SiC sandpaper (Buehler Ltd.) under water for 30 s, and then cleaned in deionized water using ultrasonic cleaning for 5 min to remove any possible debris. The composite discs were stored in ambient atmosphere accompanied with the dentin/enamel discs for 24 h to be naturally dried.
Material | Organic/resin matrix | Content (wt) | Inorganic/filler | Content (wt) |
---|---|---|---|---|
Dentin | Collagenous proteins | ∼20–23 | Mineral apatite | ∼70–77 |
Enamel | Enamel proteins | ∼1 | Mineral apatite | ∼96 |
Filtek Z250 | BisGMA, BisEMA, UDMA, TEGDMA | ∼20 | ZrO/SiO 2 | ∼80–82 |
Filtek LS | Bis-3,4-epoxycyclohexylethyl-phenyl-methylsilane, 3,4-epoxycyclohexylcyclopolymethylsiloxane | ∼24 | Quartz, yttrium fluoride | ∼76 |
a Manufactures data. BisGMA: bisphenol A diglycidyl ether dimethacrylate; BisEMA: bisphenol A polyethylene glycol diether dimethacrylate; UDMA: urethane dimethacrylate; TEGDMA: triethylene glycol dimethacrylate.
2.2
Non-thermal atmospheric plasma brush
The non-thermal atmospheric plasma brush employed in this study was designed by the Plasma Research Center at University of Missouri and Los Alamos National Laboratory. The detailed information about this device can be found in previous publications . Compressed argon gas (ultra high purity) was used as the plasma gas supply. A MKS mass flow controller (MKS Instruments Inc., Andover, MA, USA) was introduced to adjust the argon gas flow rate. A glow discharge by the direct current power source (Model 1556C, Power designs Inc., Westbury, NY, USA) was ignited between the two electrodes in a walled, Teflon chamber ( Fig. 1 A ). One of the electrodes was attached to a ballasted resistor which controls the discharge current. The other electrode was grounded for electrical safety. The formed plasma discharge could be blown out of the chamber to create a brush-shaped non-thermal plasma jet, which was generated in about 2 s after turning on the power. Fig. 1 B shows a photograph of the plasma brush on a tooth in the atmospheric environment. Plasma temperatures were characterized by a type K thermocouple and infrared imaging.
The above dried dentin, enamel and composite discs were divided into different treatment groups, respectively, with 6 discs in each group. Every specimen was examined carefully under an optical microscope to avoid any micro fissure or defect on the surface before use. Each of the naturally dried discs was placed on a stage at a distance of 2 mm between plasma brush and specimen surface. The argon flow rate was fixed at 2000 sccm, at which the length of the brush was about 6–8 mm with plasma temperature at less than 38 °C. The input power (5 and 10 W) and treatment time (5, 15, 30, 45 s) were varied.
2.3
Surface contact angle measurement
The wettability of the surfaces to water was determined with static contact angle measurements. Right after plasma treatment, the contact angles of the surfaces in each group ( n = 6) were measured by using a contact angle goniometer (A-100, Ramé-hart Inc., NJ, USA). Right above the specimen stage was the tip of a syringe that could be set to dispense a predetermined amount of water. Each time 0.5 μL deionized water droplet was dispensed on each surface. After the water was applied on the surface for 5 s, the droplet arc and the angle of contact ( θ ) at the interface were traced and recorded ( Fig. 2 ). The contact angles of the discs were also tested and recorded before exposed to plasmas. The results were analyzed by one-way ANOVA and t -test ( α = 0.05) by SPSS Version 11.0.
2.4
X-ray photoemission spectroscopy (XPS) measurement
The direct and instant chemical surface modification was measured using quantitative X-ray photoemission spectroscopy. The specimens were placed on graphite tape and XPS data were acquired using a Kratos AXIS HS electron spectrometer (Kratos Analytical Ltd., Manchester, UK) with an Al Kα X-ray source. All compositional measurements were acquired at surface normal with charge neutralization (binding energy shifts for charge compensation were unnecessary). For each specimen, a compositional survey scan was acquired using a pass energy of 80 eV and core level spectra with a pass energy of 20 eV. Two or more replicates of each specimen were analyzed and averaged to obtain the reported atomic percent (at.%) values. Data analysis was performed with Vision Processing data reduction software (Kratos Analytical Ltd.) and CasaXPS (Casa Software Ltd., Teignmouth, UK). The concentration analysis was performed by integrating the cross-section-normalized Gaussian peaks of the relevant core levels both manually and by CasaXPS (Casa Software Ltd.), and the results were found to be within a maximum of 2% of each other; the CasaXPS values are reported.
2.5
Surface assessment using scanning electron microscope (SEM)
The un-treated and plasma-treated surfaces with different treatment times were observed in a Philips XL30 ESEM-FEG (Philips, Eindhoven, Netherlands). Specimens were sputter-coated (SCD050, Leica Microsystems GmbH, Wetzlar, Germany) with Au–Pd alloy and observed at a 5 or 15 kV accelerating voltage.
2
Materials and methods
2.1
Specimen preparation
Extracted non-carious human third molars were collected after the patients’ informed consent was obtained under a protocol approved by the University of Missouri-Kansas City adult health sciences institutional review board. The teeth were stored in 0.9% (w/v) phosphate buffered saline (PBS) containing 0.002% sodium azide at 4 °C, and the storage period was less than 1 month before use. The roots of molars were removed to 2–3 mm below the cemento-enamel junction (CEJ) using a water-cooled low-speed diamond saw (Isomet, Buehler Ltd., Lake Bluff, IL, USA). The teeth were then adhered to aluminum stubs using a cyanoacrylate adhesive (Zapit, Dental Ventures of America, Corona, CA). To make a dentin disk, the occlusal one-third to one-half was removed using the same water-cooled diamond saw until all enamel was removed from the resulting dentin surface (as determined by reflective light microscopy). The second cut was made perpendicular to the long axis to produce a slice with a thickness of ∼2.0 mm and a diameter of ∼10 mm. To make an enamel disk, two similar cuts were also performed; but for the first cut, the occlusal one-fourth to one-fifth was removed to make sure that only enamel was shown on the resulting surface. The dentin and enamel discs (∼10 mm in diameter) were polished with 600-grit SiC sandpaper (Buehler Ltd.) under water for 30 s, and then washed using ultrasonic cleaning for 5 min in deionized water to remove any possible debris. These discs were stored in ambient atmosphere (temperature ≈20 °C, relative humidity ≈15%) for 24 h to be naturally dried.
Two different commercial dental composites were employed in this study. The Filtek™ Z250 (Z250) was BIS-GMA based, and the Filtek™ LS (LS) was silorane-based (both were from 3M ESPE Dental Products, St. Paul, MN, USA). The chemical composition of these two composites is shown in Table 1 . Each composite was injected into a polytetrafluoroethylene mold of 10 mm in diameter and 2 mm in thickness, covered with a Mylar film (Fisher Scientific, Pittsburg, PA, USA) and light cured by a conventional dental light polymerization unit (Spectrum Light, DENTSPLY, Milford, DE, USA) at an output power of 550 mW/cm 2 for a period of time according to its instructions (Z250: 20 s; LS: 40 s). After curing, the mold and the film were removed. The specimens were polished with 600-grit SiC sandpaper (Buehler Ltd.) under water for 30 s, and then cleaned in deionized water using ultrasonic cleaning for 5 min to remove any possible debris. The composite discs were stored in ambient atmosphere accompanied with the dentin/enamel discs for 24 h to be naturally dried.
Material | Organic/resin matrix | Content (wt) | Inorganic/filler | Content (wt) |
---|---|---|---|---|
Dentin | Collagenous proteins | ∼20–23 | Mineral apatite | ∼70–77 |
Enamel | Enamel proteins | ∼1 | Mineral apatite | ∼96 |
Filtek Z250 | BisGMA, BisEMA, UDMA, TEGDMA | ∼20 | ZrO/SiO 2 | ∼80–82 |
Filtek LS | Bis-3,4-epoxycyclohexylethyl-phenyl-methylsilane, 3,4-epoxycyclohexylcyclopolymethylsiloxane | ∼24 | Quartz, yttrium fluoride | ∼76 |
a Manufactures data. BisGMA: bisphenol A diglycidyl ether dimethacrylate; BisEMA: bisphenol A polyethylene glycol diether dimethacrylate; UDMA: urethane dimethacrylate; TEGDMA: triethylene glycol dimethacrylate.
2.2
Non-thermal atmospheric plasma brush
The non-thermal atmospheric plasma brush employed in this study was designed by the Plasma Research Center at University of Missouri and Los Alamos National Laboratory. The detailed information about this device can be found in previous publications . Compressed argon gas (ultra high purity) was used as the plasma gas supply. A MKS mass flow controller (MKS Instruments Inc., Andover, MA, USA) was introduced to adjust the argon gas flow rate. A glow discharge by the direct current power source (Model 1556C, Power designs Inc., Westbury, NY, USA) was ignited between the two electrodes in a walled, Teflon chamber ( Fig. 1 A ). One of the electrodes was attached to a ballasted resistor which controls the discharge current. The other electrode was grounded for electrical safety. The formed plasma discharge could be blown out of the chamber to create a brush-shaped non-thermal plasma jet, which was generated in about 2 s after turning on the power. Fig. 1 B shows a photograph of the plasma brush on a tooth in the atmospheric environment. Plasma temperatures were characterized by a type K thermocouple and infrared imaging.
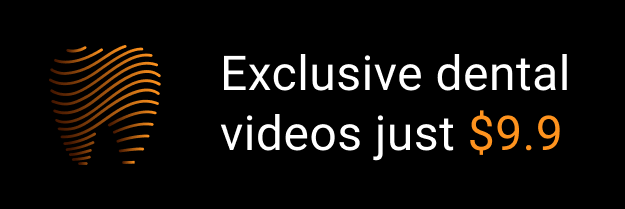