Introduction
The purpose of this study was to apply a previously reported homologous model for quantifying and visualizing the three-dimensional (3D) cranio-mandibular morphology.
Methods
Twenty-eight 3D cone-beam computed tomography (CBCT) images of Japanese patients (7 to 13 years of age) showing skeletal Class 1 malocclusions were used in this study. Wire mesh fitting was conducted for each patient based on the assignment of landmarks to each 3D surface (homologous modeling). Cranial and mandibular images were generated using surface rendering of the CBCT images. The mean and standard deviation of each point on the wire mesh were calculated as the normative mean. Two operators conducted the process twice for seven patients, with a one-week interval between fittings. The intra- and inter-examiner reliability were determined using the 95% confidence interval minimal detectable change (MDC 95 ) for random error and intra-class correlation coefficients (ICCs).
Results
The intra- and inter-examiner reliability of the mesh fitting method were almost perfect. Normative ranges of the cranial and mandibular surfaces of Japanese were determined.
Conclusions
A clinical method that enables practitioners to quantify and visualize the hard tissues of a patient’s face in three dimensions with almost perfect reliability is presented. This method allows practitioners to evaluate how patients’ skeletal characteristics differ from normative means in three dimensions.
Graphical abstract

Highlights
- •
A wire mesh fitting was conducted on each cranio-mandibular 3D model.
- •
The normative range of each point on the wire mesh was calculated.
- •
We proposed a process that effectively evaluates the 3D deformation of a new patient.
- •
The intra- and inter-examiner reliability were found to be “almost perfect.”
Lateral cephalograms have been used for orthodontic diagnoses and treatment planning since the early 20 th century. , Cephalograms enable the quantification of craniofacial morphology using linear and angular measurements, followed by statistical comparisons to age- and sex- matched controls. It can be said that craniometry and cephalograms enabled Edward H. Angle to develop systematic orthodontic treatments.
Although cephalograms are still a commonly used tool in contemporary orthodontics, certain drawbacks remain, such as the identification of landmarks being influenced by the reduction from three-dimensional (3D) to two-dimensional (2D) space. For example, structures on the left and right are projected onto radiographs with different magnification rates. Landmarks on structures that are ill-defined due to the superimposition of bilateral structures with many craniofacial structures overlying each other were difficult to identify in 2D images. The sutures of the cranium are biologically meaningful, but it is difficult to identify such landmarks on cephalograms. Posterior-anterior cephalograms are also easily affected by head posture, thereby reducing the significance of measurements.
To overcome these disadvantages, computed tomography (CT) was developed in 1972. Today, CT is useful for the diagnosis, analysis, and treatment planning for patients with orthognathic and orthodontic anomalies. However, to quantify the CT data, the information included in the 3D structures is simplified as angles and distances in the same manner as in 2D cephalograms. Although several studies have conducted surface analyses to depict differences before and after treatment, , additional information, such as surface topography, has been largely ignored. Why 3D analyses have not been developed further may be due to the technical challenges associated with the analysis of 3D structures. Theoretically, as 2D data have a size of x 2 , the size of 3D data should be x 3 , leading to significantly greater computation costs, algorithm complexity, and visualization difficulties.
In contrast, in biological studies, shape analyses had been traditionally developed and used for 2D and 3D analyses. A variety of biological processes produce differences in shapes between individuals in whole or in part, including disease, mutations, ontogenetic development, and adaptation to local geographic factors. Shape analyses aid in understanding those diverse causes of morphological variation and transformation. Recently, the concept of geometric morphometrics, a kind of shape analysis, was developed and made a major impact on the field of shape analyses; geometric morphometrics involves studying shapes using landmark and semi-landmark coordinates that are capable of capturing all of the geometric information that remains when the location, scale, and rotational effects are filtered out from a 2D or 3D object. Geometric morphometrics allows us to visualize differences among complex shapes by maximizing anatomical distinct shape variations among individuals through statistical approaches. For example, several previous studies have applied geometric morphometrics to 3D human faces, helping us to understand the facial morphology in a statistical way.
Several methods for assessing geometric morphometries are known. Bookstein , developed a sliding semi-landmarks method wherein templates and sliding landmarks are superimposed onto a surface of interest. This method minimizes the Procrustes distance or bending energy associated with thin-plate deformation. , Landmark-free superimposition for a 3D shape analysis using the Generalized Procrustes Surface Analysis has also been reported. More recently, a homologous model was developed for the 3D analysis of the body shape. , This model is characterized by a wire mesh fitting followed by landmark identification while minimizing the external and internal energy functions. The external energy function was based on the Euclidean distance between corresponding points of the template models and those of the target models, while the internal energy function was based on the local deformation of the template models. With this homologous modeling, a novel method for detecting the 3D mandibular changes related to soft-diet feeding in mice using micro-CT was reported. In that study, the authors first used the homologous modelling on the mandibular surface of mice to assess the quantitative topographic variation. The results indicated that homologous modeling is a reliable and versatile method for the visualization of the 3D distribution of significant -differences on the surface. This study suggested the possibility of a new method of CT assessment known as “surface-based 3D cephalometry” in human subjects. Surface-based 3D cephalometry enables the objective immediate and long-term postoperative assessment of virtual planned and assisted craniofacial surgical procedures. It is expected to be a powerful tool for the planning, monitoring, and evaluation of craniofacial morphology and growth.
Thus, in the present study, we proposed and tested the application of homologous modeling for surface-based 3D cephalometry in humans by performing CBCT measurements and statistical comparisons of age- and sex- matched controls. To this end, we determined the normative range for a Japanese population and also demonstrated the reproducibility of this method.
Materials and methods
Samples
Ethical approval for this study was obtained from the Institutional Review Board of Osaka University (No. H30-E5-1). This retrospective study included 28 patients (mean age = 10.1 ± 1.7 years old; boys n = 13, girls xn = 15) who underwent cone beam computed tomography (CBCT) in private orthodontic clinics. The cephalometric data of the patients are described in Table I . Prior to the study, the calculation of the required sample size was conducted based on the sampling size of the population mean. A sample size of 28 achieved a 0.5 margin of error with a 99% confidence interval, assuming that the population standard deviation of ANB is 1.0 degree. The inclusion criteria were as follows: age, 7 to 13 years; skeletal 1 malocclusion, a history of diagnostic CBCT for nonroutine orthodontic treatment (to minimize radiation exposure, we performed the scans only when the diagnostic benefits outweighed the risks of radiation exposure). The exclusion criteria were as follows: a history of orthodontic treatment, craniofacial or growth abnormalities, and systemic disease or temporomandibular joint disorders. The CBCT examinations were performed by a CBCT scanner with low-dose modes (Alphard-3030; Asahi Roentgen Ind. Co., Ltd., Kyoto, Japan). CBCT images were taken at 80 kV and 2 mA. Axial images were obtained with the X-ray beam parallel to the Frankfort plane. The field of view was 20 × 20 cm. The voxel size was 0.39 × 0.39 × 0.39 mm. Low-dose modes allow for 3D images to be obtained with less radiation exposure to patients in comparison to panoramic and cephalometric radiographs, while providing equivalent or better resolution with more information (i.e., details of fractured teeth, anatomical structures for the placement of implants, the location of impacted teeth, resorption of adjacent teeth, and airway morphology). Additionally, CBCT data can also be used to generate 2D cephalograms and a panoramic radiograph in order to avoid taking conventional X-rays, which would be associated with further radiation exposure. In our cases, we primarily used the CBCT data for the examination of the location of impacted teeth and resorption of adjacent teeth as recommended in a previous study published by Palomo and colleagues. As a secondary purpose, for these patients, we used the CBCT data to generate 2D frontal and lateral cephalograms and a panoramic radiograph and avoided taking conventional X-rays, which require a field of view that measures 20 × 20 cm.
Measurement | Present study | Normative range | ||
---|---|---|---|---|
Mean | SD | Mean | SD | |
SNA (°) | 80.0 | 2.6 | 82 | 3 |
SNB (°) | 77.2 | 2.9 | 79 | 3 |
ANB (°) | 2.8 | 1.2 | 3 | 1 |
SN to maxillary plane (°) | 2.4 | 2.8 | 8 | 3 |
Wits Appraisal (mm) | −2.6 | 2.3 | 0 | N/A |
Upper incisor to maxillary plane angle (°) | 116.7 | 6.8 | 108 | 5 |
Lower incisor to mandibular plane (°) | 93.0 | 6.8 | 92 | 5 |
Interincisal Angle (°) | 121.4 | 9.5 | 133 | N/A |
Maxillary mandibular planes angle (°) | 28.9 | 3.3 | 27 | 5 |
UAFH (N-MxP) (mm) | 53.1 | 3.5 | 55 | 3 |
LAFH (Me-MxP) (mm) | 61.2 | 4.6 | 66 | 4 |
LAFH/TAFH (%) | 53.5 | 1.4 | 55 | 3 |
Lower incisor to APo line (mm) | 4.6 | 2.5 | 2 | N/A |
Lower lip to Ricketts E-Plane (mm) | 3.4 | 2.2 | −2 | N/A |
Segmentation
Three-dimensional reconstructions of the cranium and mandible were obtained using the ITK-SNAP open-source software program (ITK-SNAP, National Library of Medicine and National Institutes of Health, Bethesda, MD, USA; Fig 1 ). The program stores slices captured by CBCT scanners as digital imaging and communications in medicine (DICOM) images and generates virtual 3D models corresponding to anatomical parts of the human body by allowing the conversion of a stack of DICOM files to a surface STL file. After importing the DICOM files into the ITK-SNAP software program, the cranium and mandible surfaces were segmented using combined semi-automatic and manual segmentations. First, one of the authors (YT) manually determined the threshold values (lower to higher) for each patient so that the mandibular and cranial shapes could be segmented according to a previous report. Next, by combining the neighboring voxels showing grayscale values ranging between the determined thresholds, 2D areas inside the cranial and mandibular structures were determined for each slice image in the DICOM files. If necessary, a “paintbrush” tool for manual segmentation was used to remove areas not relevant to the structure of interest. The series of the determined areas for each slice image was combined as a 3D surface model, and these models of the cranium and mandible were exported as .stl files.

Landmark identification and coordinate system
Each 3D surface model, scaled to 75% of its actual size, was displayed on a 17-inch LCD monitor (1701FP; Dell Inc., Round Rock, TX, USA). The positions of 23 and 45 landmarks on the cranial and mandibular surfaces, respectively ( Supplementary Fig 1 and Supplementary Text 1 ), were identified by a visual inspection of the image and digitized using a computer mouse cursor and commercial software program (HBM Rugle, Medic Engineering Co., Kyoto, Japan). Landmark selection is described in the Supplementary Text 2 and Supplementary Figures 2 and 3 . The system of coordinates of each 3D image was established based on the landmarks for each cranial and mandibular surface ( Fig 2 ). Namely, the nasion was defined as the origin of the cranial and mandibular surfaces. The sagittal plane was defined as a plane passing through the origin and perpendicular to the line through the right and left zygomaticofrontal sutures. The axial plane was defined as a plane passing through the origin and parallel to the line connecting the midpoints of the bilateral porions and the bilateral orbitales, representing the Frankfort horizontal plane. The coronal plane was defined as the plane passing through the origin and perpendicular to both the axial and sagittal planes.

Homologous model
To enable statistical analyses based on geometrics morphometrics, the data for each individual were transformed to a homologous model using the Homologous Body Modeling (HBM) software program (National Institute of Advanced Industrial Science and Technology, Tsukuba, Japan). Homologous modeling is the reconstruction of data points in such a way that the data for each subject consist of the same number of data points of the same topology, and each data point has the same anatomical position across the subjects. Figure 1 shows a schematic illustration of the homologous modeling. As described in a previous research, this technique permits the extraction of the relevant surface anatomy from CBCT data while removing and/or smoothing non-relevant data. The result is high-resolution, 3D surface data that provide enough detail to facilitate a quantitative assessment while maintaining small file sizes that are easily manipulated and portable to a range of visualization technologies.
In the present study, for homologous modeling for each patient, we employed a set of pre-determined cranial and mandibular template models consisting of 54,426 and 16,438 data points, respectively (template models as .stl files are available as Supplementary Data ). One of the authors (YT) preformed the landmark identification on the template models utilizing the aforementioned method. With a set of cranial and mandibular template models and the corresponding landmarks, wire mesh fitting of the template models into each patient’s 3D surface model was performed based on the assignment of landmarks to each 3D image using the HBM software program. The program fit template models onto each patient’s model using the landmark positions as anchors, based on a non-rigid shape registration wherein the calculations are initialized by the rigid iterative closest point. To preserve the local geometric features, the non-rigid deformation of a whole mesh is approximated by the combination of piecewise similarity deformation (for detailed calculations, please see the previous study , ). Finally, for each patient, this method generated a point cloud from the nodes of the wire mesh: a set of 54,426 and 16,438 data points in a 3D coordinate system for the cranial and mandibular surfaces, respectively.
Reproducibility of homologous modeling
Seven randomly selected CBCT data points were used to examine the inter- and intra-observer reliability of a series of the processes mentioned above, consisting of landmark identification and homologous modeling. This identification and modeling process was repeated on two separate occasions by examiners A and B, orthodontists with more than seven years of clinical experience each; there was an interval of one week between sessions. The aforementioned coordinate system and the pre-determined cranial and mandibular template models, which were established by an author YT, were employed for these reproducibility examinations. The reproducibility of each model between sessions and between examiners was calculated as the absolute difference between X-, Y-, and Z-values ( <SPAN role=presentation tabIndex=0 id=MathJax-Element-1-Frame class=MathJax style="POSITION: relative" data-mathml='d¯’>?⎯⎯d¯
d ¯
) of each corresponding node of wire mesh of the homologous model and statistically tested using the intra-class correlation coefficient (ICC). “ Moderate ” and “ substantial ” agreements were defined as 0.40 < ICC ≤ 0.60 and 0.60 < ICC ≤ 0.80, respectively; an ICC ≥ 0.81 indicated “ almost perfect ” agreement.
Based on the Bland-Altman analysis, the 95% confidence interval minimal detectable change (MDC 95 ) was calculated for each point using the following equation:
MDC95=SDd×1.96
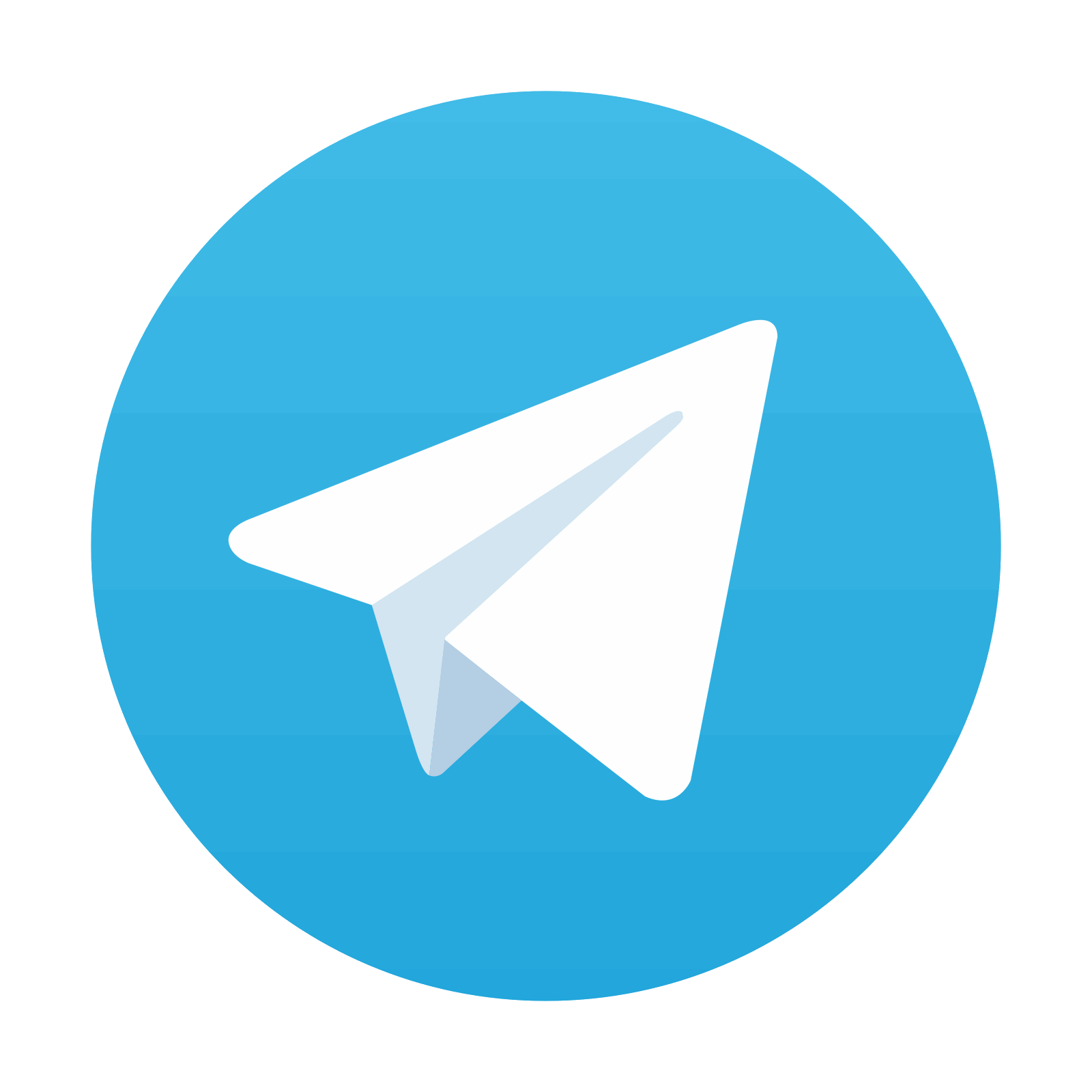
Stay updated, free dental videos. Join our Telegram channel

VIDEdental - Online dental courses
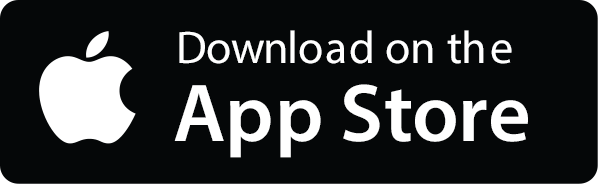
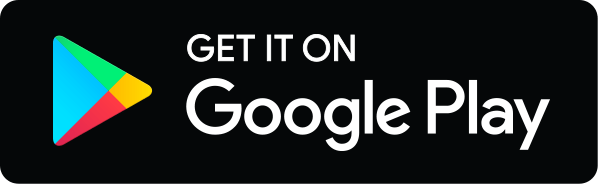
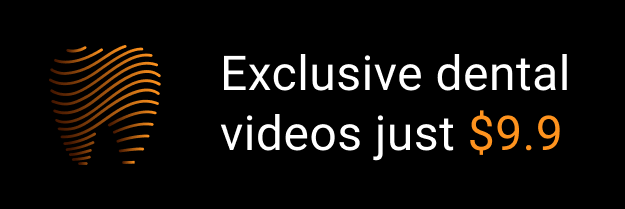