Graphical abstract

Abstract
Objective
The goal of the study was to characterize the efficiency of polymerization of Type 1 and Type 2 initiators for visible light cure of a BisGMA-TEGDMA monomer mixture.
Methods
Raman spectroscopy was used to follow conversion during polymerization of a BisGMA-TEGDMA mixture using a Type I photoinitiator diphenyl(2,4,6 dimethylbenzoyl)phosphine oxide (TPO) and a Type II photoinitiator camphorquinone (CQ) and an amine, both initiators at 0.5 wt.%. Different light exposure times and storage times after light curing were used as variables.
Results
There was a significant difference between the relative exposure times of TPO and CQ/amine (5 s for TPO vs. 20 s for CQ/Amine) for attaining maximum % conversion (78% in TPO vs. 65% in CQ/Amine). There was also a significant difference in the effect of storage time (no effect in TPO vs. increased % conversion with CQ/Amine). These effects are attributed to differences in the rate controlling steps of free radical generation in Type 1/Type 2 initiators, and the potential for radiative and non-radiative energy losses in CQ/Amine in its excited state.
Conclusions
The results confirm that photo-polymerization of BisGMA is much more efficient with TPO than with CQ/amine. Both exposure and storage times were important variables in CQ/amine, but not in TPO.
Significance
TPO photolysis generates significantly more free radicals with potentially very little radiative and non-radiative energy loss in comparison with CQ/amine. The resulting improved monomer conversion is of major importance in resisting chemical and mechanical degradation and preventing toxicological adverse effects.
1
Introduction
The extent of conversion in BisGMA monomer during photo-activated polymerization is known to be adversely affected due to association between BisGMA molecules . The structure of BisGMA is shown in Fig. 1 . Despite the presence of two hydroxyl groups in the structure that can promote intra-molecular hydrogen bond, rigidity constraints due to the bulky aromatic groups that are located in the vicinity of the hydroxyl groups rule out intra-molecular association. This limits hydrogen bonding to intermolecular association, leading to a highly viscous liquid that slows the polymerization rate and restricts the degree of polymerization. The traditional visible light photo-initiator used in dentistry to cure BisGMA is camphorquinone (CQ) together with an amine as a hydrogen donor and co-initiator. This combination is known to be relatively slow and not optimally efficient. The maximum conversion in BisGMA-TEGDMA mixture using CQ/amine is limited to ≈65% even after a delayed dark cure. For this reason, more efficient and reactive initiators such as acyl phosphine oxides have been introduced.

It is important to consider the factors that influence the ability of photoinitiator molecules to generate free radicals in assessing how efficiently each initiator can polymerize a selected monomer. A photoinitiator absorbs energy from photons at a certain wavelength range during light exposure, facilitating its dissociation into two or more free radicals. However, the mechanism of photolysis to generate free radicals may differ depending on the choice of the initiator. For example, CQ, a Type 2 photoinitiator, absorbs light in the wavelength range of 400–500 nm (with peak at 470 nm). The energy at this wavelength range (≈55–65 kcal/mol) helps to elevate the energy of the molecule to its excited singlet state CQ (S) and to its triplet state CQ (T) [by inter-system crossing from the singlet state], but the absorbed energy is inadequate to cause direct scission of the molecule to free radicals . For this reason, the amine is added to the initiator to facilitate the excited state of the initiator molecule to extract a hydrogen atom to form CQ ketyl radical (CQH ) and to convert the amine to amino-alkyl radical (A ), as illustrated in Fig. 2 (a). But this process involving hydrogen transfer as a rate determining step is a slower cure option and is used in dental applications to take advantage of photo-bleaching, better depth of cure and oxygen inhibition effects . Naturally, the introduction of hydrogen transfer step has potential implications for the kinetics and extent of polymerization.

A number of studies have also explored a Type 1 photoinitiator diphenyl(2,4,6 trimethyl-benzoate) phosphine oxide (TPO) for polymerization of dental monomers . The photolysis of TPO involves excitation of the initiator molecule to its excited states on light exposure at a lower wavelength region (range 230–430 nm, peak 385 nm). The amount of energy absorbed in this case is higher (≈68–75 kcal/mol), and it is sufficient to effect direct fragmentation of the TPO initiator molecule to free radicals. This is illustrated in Fig. 2 (b). This process does not require an additional hydrogen transfer step needed in CQ/amine system. This may be favorable for faster kinetics and a higher level of conversion in the TPO system.
Another factor that may adversely affect the generation of free radicals from the excited state of photoinitiator molecules is the potential for energy dissipation of the excited state without producing free radicals. There are competing energy dissipation processes through which the excited state is reverted to ground state without generating free radicals. This is better understood by using the energy changes associated with a Jablonski energy diagram for photo-activated processes . For simplicity, the illustrative diagram shown in Fig. 3 uses only one singlet (S 1 ), and one triplet state (T 1 ). The excited states [(S 1 ) and (T 1 )] may lose energy and revert to ground state (S 0 ) through radiative emissions and non-radiative processes without generating free radicals. For example, fluorescence (radiative emissions from the singlet state to ground state (S 1 → S 0 )) and phosphorescence (radiative emissions from triplet state to ground state (T 1 → S 0 )) can both dissipate energy of the excited state of the initiator without yielding any free radicals. Similarly, non-radiative energy losses (e.g., oxygen and monomer quenching by both excited states (S 1 ) and (T 1 )) can also dissipate energy without producing free radicals. Such energy dissipation events may strongly depend upon the relative life times of the respective excited states.

Previous studies have shown that photopolymerization in dimethacrylate monomers can be more rapid and efficient by using TPO as initiator . Some studies have also identified some limitations such as decreased depth of cure in filled composites when TPO alone is used . Mismatch in the refractive index between the resin matrix and filler particles can accentuate this problem in resin composites . However, optimized mixtures of CQ/amine and TPO initiator systems, proper choice of filler composition and newer Type 1 initiator systems (e.g., acyl- and diacyl-germane-based initiators) can overcome some of these problems .
The goal of this study was to carry out a systematic investigation of conversion in a BisGMA-TEGDMA blend using CQ/amine and TPO as initiators, respectively, using Raman spectroscopy. The null hypotheses tested are: (1) the level of conversion of BisGMA-TEGDMA mixture is not significantly different as a function of initiators (CQ/amine vs. TPO), (2) the level of conversion is not significantly different as a function of cure exposure time (ET) for initiators CQ/amine and TPO, (3) the dark cure dependence on storage time (ST) after initial light cure is not significantly different for CQ/amine and TPO as initiators.
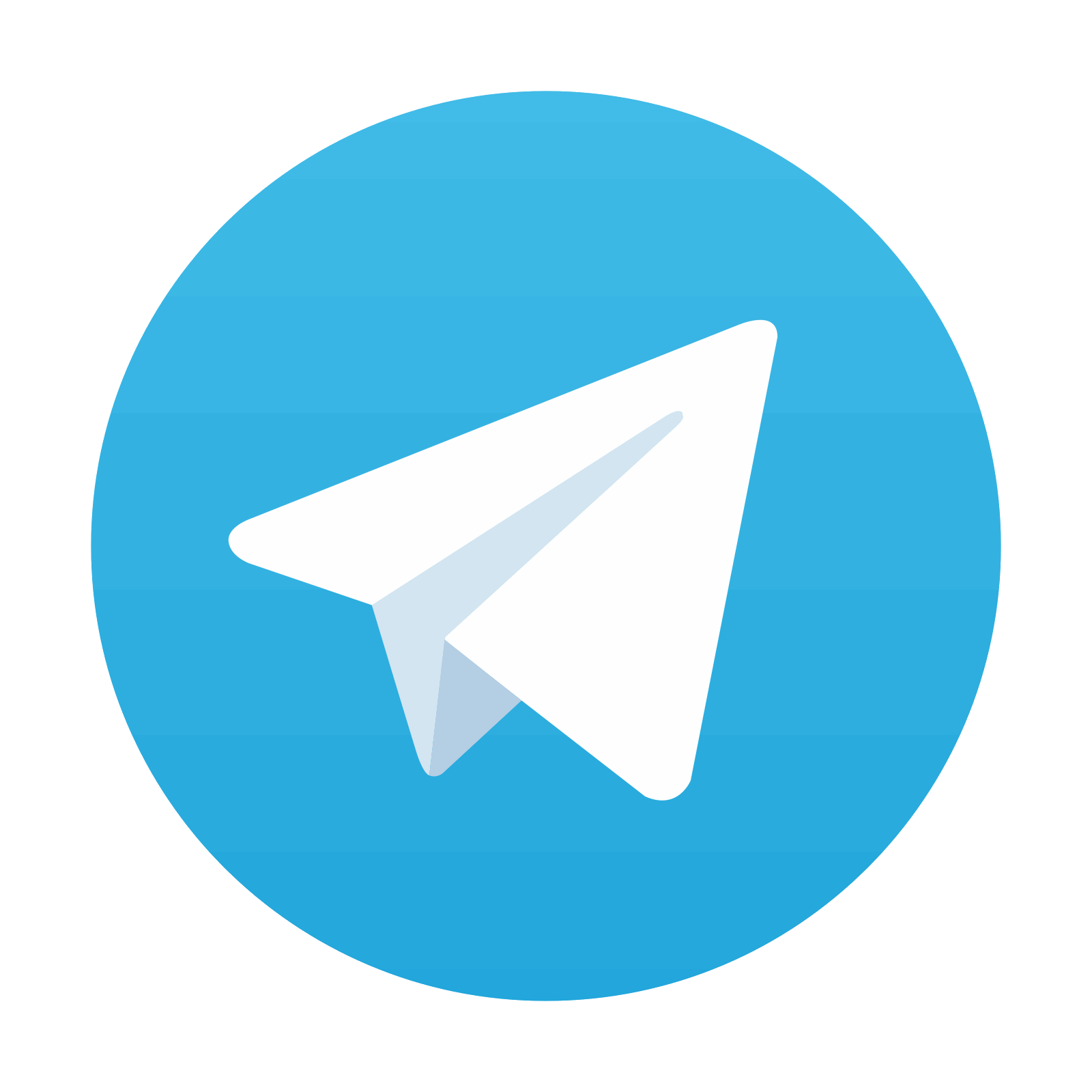
Stay updated, free dental videos. Join our Telegram channel

VIDEdental - Online dental courses
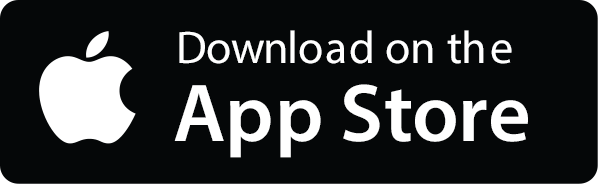
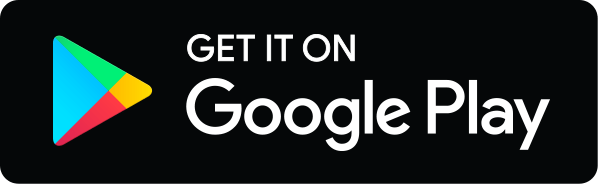