Abstract
The osteoinductive potential of periosteum and bone can be stimulated by elevating the periosteum in a distraction-like modus, but also by an ad-hoc elevation. This was tested and proved in an experiment in 9 Goettingen mini-pigs with elevation heights of 5, 10 and 15 mm. Specially designed and perforated titanium meshes were implanted subperiosteally to compare the dynamic elevation procedure with static shielding. The cumulative results for bone formation underneath the mesh were 66% in dynamic and 67% in non-dynamic elevation. The cumulative results for mineralization of the bone regenerates in comparison with the underlying basal bone of the skull showed independently from the technique applied no difference bigger than 5%. No major difference in bone formation could be observed in this pilot study. The main advantages of dynamic periosteal elevation and static shielding are minimal invasion and morbidity. Periosteal elevation could be applied in cranio-maxillofacial surgery, in preimplantological augmentation and in reconstructive surgery of the skull; applications in other specialties may be possible.
In a previous experimental study, the authors showed that dynamic and static elevation of the periosteum was a safe procedure for gaining bone . The elevation technique combines aspects of distraction treatment with guided bone regeneration and results in controlled formation of new bone . In contrast to most procedures for bone augmentation in oral and maxillofacial surgery the transplantation of autogenous bone or implantation of bone substitutes is not necessary. The three major advantages of the new technique in comparison to the clinically established procedures are: the local bone does not have to be cut as in vertical alveolar ridge distraction; there is no co-morbidity due to donor site inconvenience as in local or distant bone harvesting; and there is no risk of transplant-associated infections as in xenogenous bone substitutes.
Periosteal elevation can be used to gain bone in atrophic jaws as a preimplantological treatment and in cranio-maxillofacial surgery to reconstruct large skull bone defects . Despite good results in reconstructing these defects using customized titanium and polyacryl-ether-ether-ketone (PEEK) implants the manufacturing is costly and can only be performed in specialized medical centres or institutions .
The amount of bone formed by elevation of the periosteum seems to be unlimited, being dependent solely on the elevation height of the mesh . In a previous experiment on periosteal elevation the controlled and guided elevation stimulated the osteogenic potential of the periosteum, which resulted in a quick and massive growth of bone . The elevation height of 5 mm did not seem safe enough to exclude spontaneous bone forming as is known from traumatic bone regeneration. These results led to a second project in which maximum elevation heights three times as high were chosen.
This study addresses the periosteal bone-forming capacity in elevation heights above 5 mm, and studies whether the dynamic procedure has provable advantages compared with static shielding.
Material and methods
The trial was performed in the Franz-Penzolt-Center for Experimental Medicine of the University of Erlangen-Nuremberg, Germany (acknowledged governmental permission: 05.06.23.1/05). The experiment was carried out on 9 female pigs with a mean weight of 34 kg (±4.8 kg) . The average age of the animals was 23 months. Three animals each were randomly assigned to three groups. The three groups were defined by different consolidation periods of 14, 28 and 42 days after the end of dynamic elevation. Each animal received one of the following augmentation procedures in the forehead region ( Tables 1 and 2 ): dynamic elevation of the periosteum of 5, 10 and 15 mm; and static elevation (shielding) of the periosteum of 5, 10 and 15 mm.
A conventional augmentation with an autogenous bone block 5 mm high was only performed in the 5 mm mesh elevation group. All animals were sedated with intramuscular ketamin (10 mg/kg body weight) (Ketavet™, Pfizer, Karlsruhe, Germany) and midazolam (1 mg/kg body weight) (Dormicum™, Hoffmann-La Roche, Grenzach-Whylen, Germany).
General anaesthesia was performed after oral intubation with a Murphy entotracheal tube (5.5–7 mm internal diameter) (Isofluran Curamed, Curamed Pharma, Karlsruhe, Germany). Intermittent positive pressure ventilation adjusted to normal end-tidal carbon dioxide level (etCO2) was established. Cardiopulmonary function was monitored during the intervention (Siemens Sirecust 630, Siemens, Erlangen, Germany). Systemic antibiotic protection was given as a single shot during the operation.
For the dynamic and static elevation of the periosteum a special elevator was designed and manufactured in cooperation with KLS Martin, Tuttlingen, Germany. A 0.25 mm thick laser-perforated titanium mesh of 30 mm ×30 mm length was fitted with threads to carry three distraction screws 22 mm long. One complete turn rendered 0.5 mm of mesh elevation. The lower ends of the threads (2 mm long) were rounded and polished for passive fixation in the underlying bone ( Fig. 1 ).

After a U-shaped incision in the forehead the frontal bone of the animal was exposed. Two elevators were placed in each animal. The rotating pins were anchored in the external bicortical layer of the frontal bone. The conventional augmentation was performed only in the animals that were allocated to the 5 mm elevation procedure. A 10 mm trephine bur was used to harvest a cortico-cancellous bone block from the external table of the skull bone.
This bone fragment was fixed with a single 2.0 mm titanium screw of variable length next to the harvesting site. The immediate, static elevation of the mesh was performed by activating the threads by 5, 10 and 15 mm, respectively. For the dynamic elevation the mesh remained in contact with the underlying bone ( Fig. 2 ). After wound closure the threads perforated the skin of the forehead to allow activation of the mesh elevators.

Periosteal elevation started after a latency period of 3 days. The mesh was elevated by 0.5 mm twice per day for 5, 10 and 15 days, respectively. No postoperative analgesia was administered.
After completion of the active elevation period the animals were killed according to the schedule in Table 1 . The procedure for killing the animals, preparing the frontal bone and the histological and micro-radiological analysis is described in previous studies . A quantitative, micro-radiological and histological analysis was performed. The microradiographs were used to determine the ratio of ‘bone volume/total area’ (BV/TA). For that purpose bone slices were gained from the regions of interest (ROI), where a maximum of bone volume had been created during the different augmentation procedures. For the micro-radiographic evaluation bone slices of 90–120 μm thickness were X-rayed with the Faxitron R (Faxitron Cabinet X-ray Systems, Faxitron X-ray Corporation, IL, USA). The X-rays were scanned and evaluated using Adobe Photoshop 6.0 graphic software. For the histological evaluation of the bone-forming processes bone specimens 30 μm thick were stained with toluidin blue and trichrome-Goldner. These stains were chosen to reveal mineralized bone underneath the meshes. Quantitative analysis was performed with the Bioquant Osteo™ program (BIOQUANT Image Analysis Corporation, Nashville, TN, USA). This program allows an exact, standardized volumetric evaluation of newly formed bone in a defined area. In this experiment, the area underneath the meshes was defined as ROI.
Statistical analysis
For statistical analysis the calculation program excel 2003 was used (Microsoft Corporation, Seattle, USA). Owing to the small numbers of animals no statistically significant differences could be measured. Absolute and relative values, as well as mean values, were evaluated.
Results
Activation of the elevation threads was possible without sedation. No perforation of the meshes occurred either in the dynamic or after static elevation ( Fig. 3 ). No wound dehiscence was seen even though the animals rubbed their heads against the steel fence. Protective head bandages could not avoid deviation of the elevation rods in some animals. Rod deviations are well known from distraction therapy and were seen in the bone specimen of animals with elevation heights of 10, respectively 15 mm (see Fig. 5 c). The angulations of the rods measured from the perpendicular axis were no bigger than 30°. So the planned elevation height could be reached.

In all specimens, new bone formation could be observed. The process of new bone formation in elevation and shielding showed a characteristic vertical growth pattern, beginning at the outer surface of the external table. Depending on the consolidation time, progressive bone formation could be observed from the underlying bone towards the meshes. In some specimens in both elevation groups, bone formation was even seen above the mesh.
The mineralization of the newly formed bone was compared with the local bone surrounding the region of interest. In dynamic periosteal elevation typical longitudinally stretched bone trabeculae can be seen and they are well known from distraction studies . The vertically oriented trabeculae showed the beginning of mineralization along their sides ( Figs. 4 and 5 , Table 3 ). The histomorphometric architecture of the newly formed bone in static elevation resembled that of woven bone. The subperiosteal space underneath the mesh showed a successive, time dependent osteogenetic formation of woven bone ( Figs. 4 and 5 ). The conventionally augmented bone blocks displayed resorption with rounding of the cranial edges, resulting in a reduction of the total volume ( Fig. 5 a). The inner structure of the bone blocks resembled the structure of the local tabular bone ( Figs. 4 and 5 ). The central pins were embedded in a thin layer of connective tissues indicating a certain, but controlled mobility. The mesh was stabilized under the tension of the rigid overlying skin and the pins inserted in the external table.

The amount of newly formed bone depends on the technique applied, the elevation height and the consolidation period ( Table 3 ). Dynamic periosteal elevation up to 5 mm elevation height rendered a BV/TA ratio of 71% after 14 days, 95% after 28 days and 69% after 42 days of consolidation. The data for immediate elevation of the mesh were 72%, 91% and 79%, respectively. Elevation up to 10 mm resulted in the dynamic group in a BV/TA ratio of 52%, 33% and 80%. The comparable data in non-dynamic mesh elevation were 45%, 55% and 75%. After a maximum elevation of the mesh of 15 mm the BV/TA ratios were 30%, 85% and 82% for the dynamic procedure. The immediate elevation of the titanium mesh up to 15 mm rendered 40%, 67% and 78% of new bone after a consolidation period of 14, 28 and 42 days, respectively. The results indicate the dependency of the amount of newly formed bone on the technique applied, the elevation height and the time of consolidation.
Mineralization of the transplanted and newly formed bone is evaluated in Tables 4 and 5 . The autogenous bone transplants had an average degree of mineralization related to the time of survival of about 60%. Dynamic and static elevation of 5 mm rendered mineralization of 59% and 64% after 14 days, 52% and 56% after 28 days and 62% and 52% after 42 days of consolidation. Elevation of 10 and 15 mm rendered comparable results for dynamic and static elevation: 55% versus 42%, 52% versus 44% after 14 days, 65% versus 55%, 49% versus 58% after 28 days and 59% versus 58%, 47% versus 60% after 42 days, respectively. The cumulative results for mineralization in all kinds of regeneration in comparison with the underlying basal bone of the skull showed, independently of the technique applied, no difference bigger than 5%.
Elevation height | |||||||||
---|---|---|---|---|---|---|---|---|---|
5 mm | 10 mm | 15 mm | |||||||
Method | Method | Method | |||||||
auto | dyn | stat | auto | dyn | stat | auto | dyn | stat | |
BV/TV | 60% | 58% | 57% | 60% | 52% | 49% | 54% | ||
BV/TV skull bone | 55% | 57% | 56% | 59% | 57% | 50% | 52% |
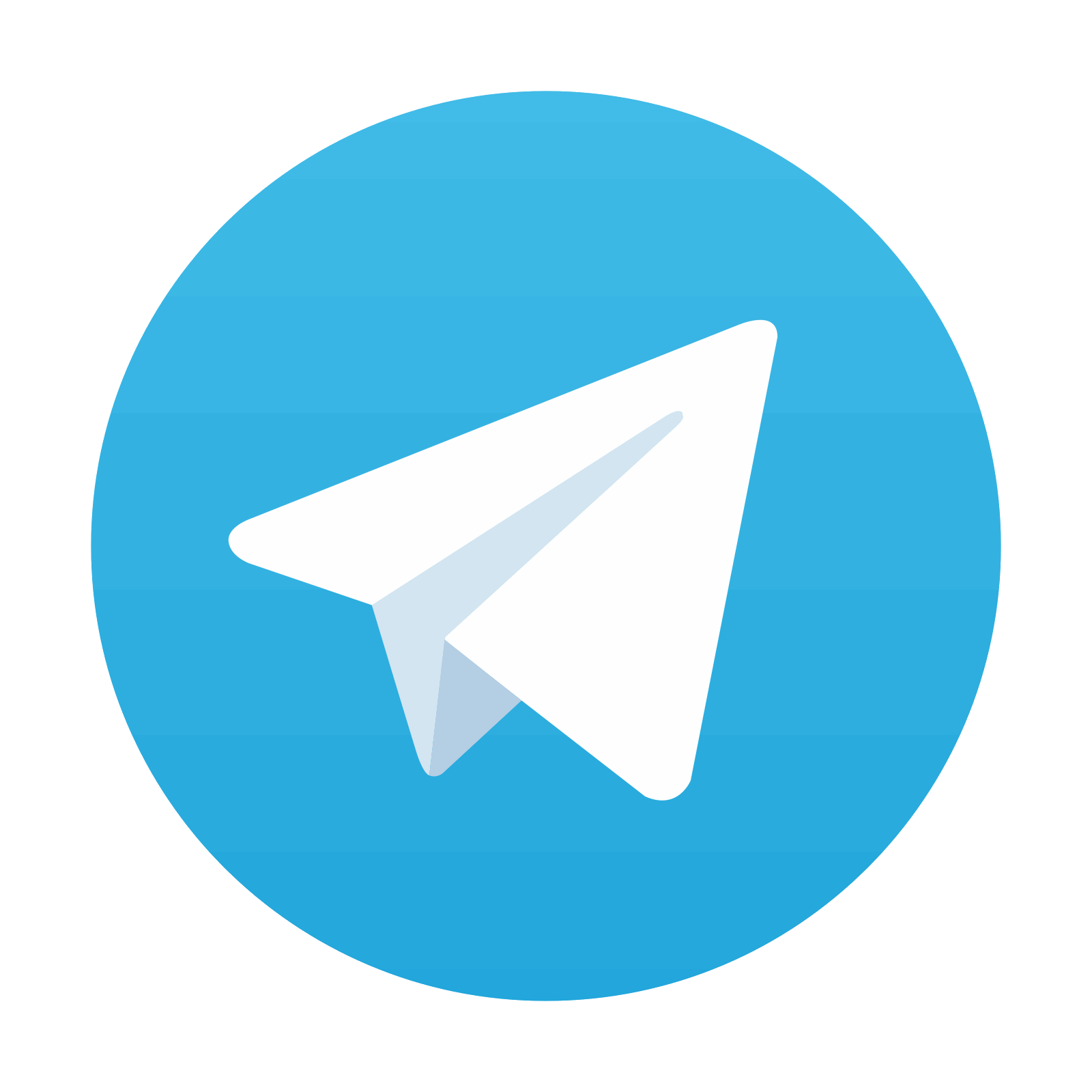
Stay updated, free dental videos. Join our Telegram channel

VIDEdental - Online dental courses
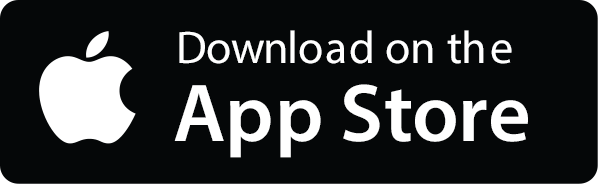
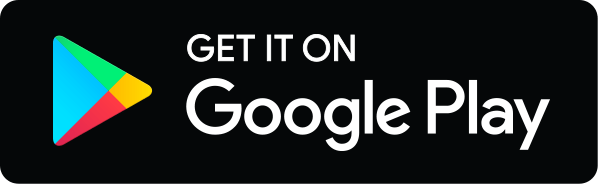
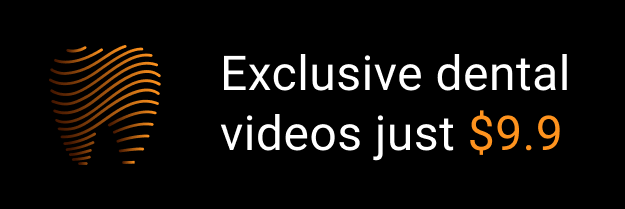