Abstract
This paper reflects on the state of the art of self-etch adhesives anno 2010. After presenting the general characteristics of self-etch adhesives, the major shortcomings of the most simple-to-use one-step (self-etch) adhesives are addressed. Special attention is devoted to the AD-concept and the benefit of chemical interfacial interaction with regard to bond durability. Finally, issues like the potential interference of surface smear and the more challenging bond to enamel for ‘mild’ self-etch adhesives are discussed.
1
Introduction
Adhesive technology has evolved rapidly since it was introduced more than fifty years ago. The main challenge for dental adhesives is to provide an equally effective bond to two hard tissues of different nature. Bonding to enamel has been proven to be durable. Bonding to dentin is far more intricate and can apparently only be achieved when more complicated and time-consuming application procedures are followed. Consequently, today’s adhesives are often regarded as technique-sensitive with the smallest error in the clinical application procedure being penalized either by rapid de-bonding or early marginal degradation. As a consequence, the demand for simpler, more user-friendly and less technique-sensitive adhesives remains high, urging manufacturers into developing new adhesives at a rapid pace.
Today’s adhesives either follow an ‘etch-and-rinse’ or a ‘self-etch’ (or ‘etch-and-dry’) approach, which differ significantly in the manner they deal with tooth tissue. Nevertheless, it should be stated that both approaches have performed successfully in laboratory as well as clinical research, while obviously there also exists a high product-dependency. Following the previous presentation (and paper) by David Pashley on ‘ The state of the art of etch-and-rinse adhesives ’, the main objective of this presentation (and paper) is to present the latest developments with regard to the self-etch approach.
2
General characteristics of self-etch adhesives
Different from etch-and-rinse adhesives, self-etch adhesives do not require a separate etching step, as they contain acidic monomers that simultaneously ‘condition’ and ‘prime’ the dental substrate. Consequently, this approach has been claimed to be user-friendlier (shorter application time, less steps) and less technique-sensitive (no wet-bonding, simple drying), thereby resulting in a reliable clinical performance , though this appeared very product-dependent. Another important clinical benefit of self-etch adhesives is the absence of, or at least lower incidence of post-operative sensitivity experienced by patients (as compared to that associated with etch-and-rinse adhesives) . This should to a great extent be attributed to their less aggressive (with respect to dentin and as compared to phosphoric-acid etching) and thus more superficial interaction with dentin, leaving tubules largely obstructed with smear (see below). All these favorable key-features have lead to the steadily growing popularity of self-etch adhesives in today’s dental practices.
Self-etch adhesives can come as ‘two-step’ and ‘one-step’ adhesives, depending on whether a self-etching primer and (mostly solvent-free) adhesive resin are separately provided or are combined into one single solution. One-step adhesives can be further subdivided into ‘two-component’ and ‘single-component’ one-step self-etch adhesives. By separating ‘active’ ingredients (like the functional monomer from water), two-component self-etch adhesives theoretically posses a longer shelf life, but additional and adequate mixing of both components is needed. The single-component one-step adhesives can be considered as the only true ‘one-bottle’ or ‘all-in-one’ adhesives, as they combine ‘conditioning’, ‘priming’ and ‘application of the adhesive resin’, and do not require mixing.
In order to enable (self-)etching, all self-etch adhesives contain water as an ionizing medium. The only exceptions are some commercially available water-free self-etch adhesives. They however require a more technique-sensitive ‘wet-bonding’ application technique (like required for acetone-based etch-and-rinse adhesives) . Sufficient surface-water should then indeed be present to enable self-etching, again leading to the question ‘how wet should dentin be?’ . In general, self-etch adhesives have the advantage to demineralise and infiltrate the tooth surface simultaneously to the same depth, theoretically ensuring complete penetration of the adhesive . With increasing depth, the acidic monomers are gradually buffered by the mineral content of the substrate, loosing their ability to further etch dentin . The morphological features of the adhesive-tooth interface produced by self-etch adhesives depend to a great extent on the manner their functional monomers interact with the dental substrate ( Fig. 1 ) . In part depending on the pH of the self-etch solutions, the actual interaction depth of self-etch adhesives at dentin differs from a few hundreds of nanometers following an ‘ultra-mild’ self-etch approach (pH > 2.5) ( Fig. 1 a), which sometimes is being referred to as ‘nano-interaction’ , (b) an interaction depth of around 1 μm for a ‘mild’ self-etch approach (pH ≈ 2) ( Fig. 1 b), (c) an interaction depth between 1 and 2 μm for an ‘intermediately strong’ self-etch approach (pH between 1 and 2; not shown), and (d) to an interaction of several micrometers deep for a ‘strong’ self-etch approach (pH ≤ 1) ( Fig. 1 c). Only with the strong self-etch adhesives typical resin tags are formed at dentin, while they are hardly formed with mild and ultra-mild self-etch adhesives or at maximum the smear plugs get slightly demineralised and subsequently resin-infiltrated.
The actual bonding performance attained by self-etch adhesives varies a great deal, depending not only on the actual class of self-etch adhesives, but certainly also on the actual composition and more specific on the actual functional monomer included in the adhesive formulation (see below).
2
General characteristics of self-etch adhesives
Different from etch-and-rinse adhesives, self-etch adhesives do not require a separate etching step, as they contain acidic monomers that simultaneously ‘condition’ and ‘prime’ the dental substrate. Consequently, this approach has been claimed to be user-friendlier (shorter application time, less steps) and less technique-sensitive (no wet-bonding, simple drying), thereby resulting in a reliable clinical performance , though this appeared very product-dependent. Another important clinical benefit of self-etch adhesives is the absence of, or at least lower incidence of post-operative sensitivity experienced by patients (as compared to that associated with etch-and-rinse adhesives) . This should to a great extent be attributed to their less aggressive (with respect to dentin and as compared to phosphoric-acid etching) and thus more superficial interaction with dentin, leaving tubules largely obstructed with smear (see below). All these favorable key-features have lead to the steadily growing popularity of self-etch adhesives in today’s dental practices.
Self-etch adhesives can come as ‘two-step’ and ‘one-step’ adhesives, depending on whether a self-etching primer and (mostly solvent-free) adhesive resin are separately provided or are combined into one single solution. One-step adhesives can be further subdivided into ‘two-component’ and ‘single-component’ one-step self-etch adhesives. By separating ‘active’ ingredients (like the functional monomer from water), two-component self-etch adhesives theoretically posses a longer shelf life, but additional and adequate mixing of both components is needed. The single-component one-step adhesives can be considered as the only true ‘one-bottle’ or ‘all-in-one’ adhesives, as they combine ‘conditioning’, ‘priming’ and ‘application of the adhesive resin’, and do not require mixing.
In order to enable (self-)etching, all self-etch adhesives contain water as an ionizing medium. The only exceptions are some commercially available water-free self-etch adhesives. They however require a more technique-sensitive ‘wet-bonding’ application technique (like required for acetone-based etch-and-rinse adhesives) . Sufficient surface-water should then indeed be present to enable self-etching, again leading to the question ‘how wet should dentin be?’ . In general, self-etch adhesives have the advantage to demineralise and infiltrate the tooth surface simultaneously to the same depth, theoretically ensuring complete penetration of the adhesive . With increasing depth, the acidic monomers are gradually buffered by the mineral content of the substrate, loosing their ability to further etch dentin . The morphological features of the adhesive-tooth interface produced by self-etch adhesives depend to a great extent on the manner their functional monomers interact with the dental substrate ( Fig. 1 ) . In part depending on the pH of the self-etch solutions, the actual interaction depth of self-etch adhesives at dentin differs from a few hundreds of nanometers following an ‘ultra-mild’ self-etch approach (pH > 2.5) ( Fig. 1 a), which sometimes is being referred to as ‘nano-interaction’ , (b) an interaction depth of around 1 μm for a ‘mild’ self-etch approach (pH ≈ 2) ( Fig. 1 b), (c) an interaction depth between 1 and 2 μm for an ‘intermediately strong’ self-etch approach (pH between 1 and 2; not shown), and (d) to an interaction of several micrometers deep for a ‘strong’ self-etch approach (pH ≤ 1) ( Fig. 1 c). Only with the strong self-etch adhesives typical resin tags are formed at dentin, while they are hardly formed with mild and ultra-mild self-etch adhesives or at maximum the smear plugs get slightly demineralised and subsequently resin-infiltrated.
The actual bonding performance attained by self-etch adhesives varies a great deal, depending not only on the actual class of self-etch adhesives, but certainly also on the actual composition and more specific on the actual functional monomer included in the adhesive formulation (see below).
3
Major shortcomings of one-step adhesives
The latest generation of most simple-to-use one-step adhesives are intricate mixes of hydrophilic and hydrophobic components. These ‘difficult’ mixtures should so far be considered as ‘compromise’ materials that have consequently been documented with several shortcomings ( Fig. 2 ) . Generally, a reduced ‘immediate’ bond strength is recorded in comparison to that measured for multi-step adhesives . In addition, any kind of ‘aging’ demonstrates a lower long-term bonding effectiveness. Moreover, numerous studies report on increased interfacial nano-leakage . One-step adhesives that are rich in HEMA, showed enhanced water sorption from the host dentin, in particular when the lining composite is not immediately cured to block these osmosis effects . On the other hand, typical is also the phase-separation described for HEMA-free/poor adhesives . An adapted ‘strong’ air-drying procedure provides a means to remove water (that is separated from the more hydrophobic components) from the interfacial area, theoretically enabling better polymerization. While this technique works on relatively flat surfaces, the water ‘bubbles’ (formed once the solvent starts to evaporate, by which the more hydrophobic monomer components no longer remain in solution) are more difficult to blow away in more complex cavity configurations. In addition, mixing all ingredients into one bottle has caused shelf-life problems , though recently some manufacturers solved this issue by using more hydrolytically resistant acrylamide monomers . Finally, inferior clinical performance of one-step adhesives confirmed the less favorable laboratory findings, while it must also be said that the latest generation of one-step adhesives definitely perform better .
4
The ‘AD-concept’ revisited as basis of durable bonding
The fundamental mechanism of bonding to enamel and dentin is essentially based on an exchange process, in which minerals removed from the dental hard tissues are replaced by resin monomers that upon polymerization become micro-mechanically interlocked in the created porosities . This process, which is called ‘ hybridization ’ on dentin, involves infiltration and subsequent in situ polymerization of resin within the created surface porosities, and thus is a process primarily based upon diffusion. While the resultant micro-mechanical interlocking is a prerequisite to achieve good bonding (certainly within clinical circumstances), the potential benefit of additional chemical interaction between functional monomers and tooth substrate components has recently regained attention . Additional ‘primary’ chemical interaction is thought to particularly improve bond durability.
The p K a value of an acid was generally considered as the major parameter that determines how molecules interact with mineralised tissues . However, it does not fully explain the mechanisms why certain molecules adhere to tooth tissue, while others do not, but rather severely decalcify it . For instance, 1 M oxalic acid (p K 1 = 1.27, p K 2 = 4.28) with a pH of 0.6 is more acidic than 10% maleic acid (p K 1 = 1.94, p K 2 = 6.23), which has a pH of 0.9. Nevertheless, oxalic acid chemically bonds to hydroxyapatite (HAp), while maleic acid decalcifies it. In other words, it is not necessarily true that the lower the pH (the more acidic), the more the solution will demineralise enamel and dentin.
The way molecules interact with HAp-based tissues has been described in the so-called ‘AD-concept’ or ‘Adhesion-Decalcification concept’ ( Fig. 3 ) . This model shows that initially all acids chemically (ionically) bond to calcium of HAp (PHASE 1). This first bonding phase goes together with release of phosphate (PO 4 3− ) and hydroxide (OH − ) ions from HAp into the own solution, such that the surface remains electro-neutral. Whether the molecule will remain bonded (PHASE 2, OPTION 1 or 2.1) or will de-bond (PHASE 2, OPTION 2 or 2.2), depends on the stability of the formed bond to Ca, or in other words on the stability of the respective calcium salt.
More specifically, molecules like 10-methacryloyloxydecyl dihydrogen phosphate or 10-MDP (as functional monomer in self-etch adhesives), but also polyalkenoic acids (as functional polymer in glass-ionomers), will chemically bond to Ca of HAp (thus according to AD-concept (PHASE 2, OPTION 1 or 2.1), forming stable calcium-phosphate and calcium-carboxylate salts, respectively, along with only a limited surface-decalcification effect. ‘Mild’ self-etch adhesives and glass-ionomers indeed only superficially interact with enamel and dentin, and hardly dissolve HAp crystals, but rather keep them in place (within a thin submicron hybrid layer; see below and Fig. 1 b).
On the contrary, molecules like phosphoric and maleic acid, but also functional monomers of self-etch adhesives like 2-(methacryloyloxyethyl)phenyl hydrogenphosphate (phenyl-P) and HEMA-phosphates, will initially bond to Ca of HAp (PHASE 1), but will readily de-bond (thus according to ‘AD-concept PHASE 2, OPTION 2 or 2.2’). The negatively loaded phosphate ions (or carboxyl groups for carboxyl-based monomers/acids) will remove the positively loaded (and thus electro-statically attracted) Ca ions from the surface, up to a certain depth depending on the application time. This results in a severe decalcification or ‘ etching ’ effect, as it is best known for phosphoric acid that is used as ‘ etchant ’ as part of the ‘ etch-and-rinse ’ approach. Because the calcium-phosphate/carboxylate bond originally formed (during PHASE 1) at the enamel/dentin surface is not stable, the bond will dissociate, leading to a typical etch pattern at enamel and a relatively deep (3–5 μm) hybrid layer at dentin that does no longer contain any HAp crystals ( Fig. 1 c).
5
Avoid ‘strong’ self-etch adhesives
‘Strong’ self-etch adhesives present rather deep demineralisation effects at both enamel and dentin ( Fig. 1 c). The interfacial ultra-structure produced by these adhesives resembles that of etch-and-rinse systems, but differs for the fact that the dissolved calcium phosphates are not rinsed away. These embedded calcium phosphates are expected to be very unstable in an aqueous environment, thereby seriously weakening the interfacial integrity. Laboratory as well as clinical data have undeniably shown that despite their rather reasonable bonding potential to enamel, strong self-etch adhesives generally underperform at dentin, in particular with regard to bond durability and restoration longevity . These data corroborate the AD-concept , and should be attributed to the low hydrolytic stability of the embedded calcium phosphates, along with the lack of stable chemical interaction with the exposed collagen.
Although manufacturers have introduced strong self-etch adhesives some years ago, especially with regard to their better etching performance at enamel, their severely compromised bonding to dentin has apparently pushed them today more toward the more promising ‘mild’ self-etch approach.
6
Importance of keeping hydroxyapatite at the interface to protect collagen and generate chemical interaction receptiveness
Indeed, ‘mild’ self-etch adhesives demineralise dentin only partially, leaving a substantial amount of HAp-crystals around the collagen fibrils ( Fig. 1 b). Dentinal collagen exposed by an etch-and-rinse procedure has been documented to be highly vulnerable to hydrolytic and enzymatic degradation processes . Smart biomimetic remineralisation procedures have been developed by Tay and Pashley to ‘repair’ the natural HAp-protection of collagen. However, the clinical applicability of such a time-consuming process is today still unclear. As a potential remedy to remineralise caries-affected dentin lesions, this procedure appears technologically more rapidly attainable to work intra-orally. Actually, the fact that an etch-and-rinse hybrid layer can be demineralised, confirms the relatively permeable nature of the resin-impregnated collagen layer and perhaps its consequent instability on the long term. On the other hand, it also underlines the great advantage of mild self-etch adhesives as they keep collagen not only encapsulated and thus protected by HAp, but also provide the potential to chemically interact with HAp .
The resultant two-fold micro-mechanical and chemical bonding mechanism of mild self-etch adhesives closely resembles that of glass-ionomers . The latter also typically present with a submicron hybrid layer that still contains substantial HAp that was not dissolved by the polyalkenoic acid. In this respect, glass-ionomers could even be regarded as a kind of mild self-etch adhesives. According to the AD-concept , polyalkenoic acid is a polymer with a multitude of carboxyl functional groups that as chemical ‘hands’ grab individual Ca-ions along the mineral substrate. This chemical bonding, combined with micro-mechanical interlocking through shallow hybridization, establishes the unique self-adhesiveness of glass-ionomers (even without any form of beforehand treatment). Glass-ionomers have indeed been recorded with the lowest annual failure rate with regard to Class-V adhesive restorations . The basic difference with ‘true’ resin-based self-etch adhesives is that the latter possess functional monomers with usually only one or two functional chemical groups with affinity to HAp. They provide individual monomers that become upon polymerization a polymer that is linked to HAp, versus glass-ionomers that make use of an already existing (polyalkenoic-acid) polymer with multiple functional groups that are attached to the polymer backbone and can ‘grab’ Ca at different and remote sites. The additional chemical bonding provided by glass-ionomers and mild self-etch adhesives is believed to be advantageous in terms of bond durability .
In case of self-etch adhesives, chemical interaction is achieved through specific functional monomers, such as 10-MDP, 4-MET (or 4-methacryloxyethyl trimellitic acid) and phenyl-P. The ionic bond formation of the carboxylic/phosphate groups of these functional monomers to Ca of HAp was first proven by Yoshida et al. in 2004 using XPS (or X-ray photo-electron spectroscopy) . However, chemical bonding potential on its own is insufficient; the formed ionic bonds should also be stable in an aqueous environment. In this sense, the chemical bonding promoted by 10-MDP is not only more effective, but also more stable in water than that provided by 4-MET and phenyl-P, in this order . The dissolution rate of the respective calcium salts of these three monomers, as measured by AAS (or atomic absorption spectroscopy), was inversely related to their chemical bonding potential, as revealed by XPS: the more intense the chemical bonding potential, the less the resultant calcium salt could be dissolved.
Confirming these experimental chemical data (and hence the AD-concept ), the bond strength to dentin of the 10-MDP-based ‘mild’ two-step self-etch adhesive Clearfil SE (Kuraray) remained high after long-term thermo-cycling, while that of Unifil Bond (GC) that contains 4-MET, significantly dropped (but only after 100,000 cycles) and that of Clearfil Liner Bond II (Kuraray) that contains phenyl-P, gradually decreased the longer the bond was exposed to thermo-cycling . Clearfil SE Bond (Kuraray) has been proven to yield reliable results in terms of bonding effectiveness and durability when compared to other commercially available self-etch adhesives, this in laboratory as well as clinical research .
The functional monomer 10-MDP bonds through its phosphate groups to HAp and peculiarly forms a regularly layered structure at the HAp surface ( Fig. 4 ) . Both XRD (or X-ray diffraction) of HAp powder allowed to interact with 10-MDP, and high-resolution TEM (or transmission electron microscopy) of 10-MDP-treated HAp powder revealed the formation of a 4-nm layered structure ( Fig. 4 ). Such ‘nano-layering’ could not be detected for the functional monomers phenyl-P and 4-MET. Each layer of this self-assembled nano-layered structure consists of two 10-MDP molecules with their methacrylate groups directed toward each other and their functional hydrogen phosphate groups directed away from each other . In between the layers, calcium salts are deposited. This high chemical affinity of 10-MDP to HAp along with nano-layering was first demonstrated on pure synthetic HAp using XRD and later confirmed by NMR (or nuclear magnetic resonance). Apatite in natural dentin is carbonated and also contains trace amounts of Na, Mg, Sr, and Al among others . Direct evidence of the formation of a nano-layered structure on natural dentin was later provided by TEM ( Fig. 5 ), and structurally by XRD of 10-MDP-treated dentin samples ( Fig. 6 ) . More recent experiments proved that nano-layering was formed at dentin when an experimental 10-MDP-based adhesive (as well as the commercially available Clearfil SE Bond of Kuraray) was applied to dentin following a clinically relevant application procedure (i.e. 20-s application followed by gently air-drying). Furthermore, rubbing the primer solution on the dentin surface intensified the nano-layering, which may explain why this ‘active’ application technique increases the bond strength as observed in previous studies. However, nano-layering was clearly less detectable by XRD at enamel, which may be due to the significantly higher crystallinity of enamel, reducing the interaction potential of 10-MDP. Dentinal HAp has not only a lower crystallinity, but also the crystal rods are smaller in size and oriented crisscross (versus parallel oriented at enamel).
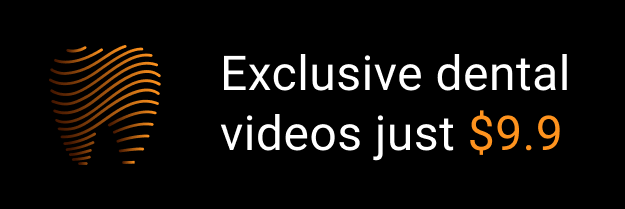