Abstract
Light curing in dentistry has truly revolutionized the practice of this art and science. With the exception bonding to tooth structure, there is perhaps no single advancement that has promoted the ease, efficiency, productivity, and success of performing dentistry. Like most every major advancements in this profession, the technology underlying the successful application of light curing in dentistry did not arise from within the profession, but instead was the result of innovative adaptations in applying new advances to clinical treatment. One cannot appreciate the current status of dental photocuring without first appreciating the history and innovations of the science and industry underlying the advances from which it developed. This review will place the current status of the art within the context of its historical progression, enabling a better appreciation for the benefits and remaining issues that photocuring has brought us. Lastly, the manuscript will present thoughts for future considerations in the field, offering suggestions as to how current advances in light-generating science might yet be adapted for dental use.
1
Introduction to the review
There is hardly a clinical procedure performed in contemporary dentistry where photocuring of some material is not required. The process seems so simple, perhaps even trivial: just point and shoot. What could be so complicated? However, there is much more to this story than that. In order to appreciate the convenience and benefits today’s state-of-the-art offers, it is first necessary to understand and value how this technology developed over time. This manuscript will provide a short review of the history underlying development of photocuring in dentistry, focusing more on issues related to construction and operation of the light sources themselves. The perspective for appreciating current technology will be established, the issues that we currently face will be addressed, and those we have yet to overcome. A summary of recent key findings is provided, and thoughts on important factors affecting the future of development in dental photopolymerization are developed.
2
Literature
2.1
Abbreviated history of light curing in dentistry
From the late 1940, with power/liquid, self-curing direct, acrylic-based restorative materials, to the late 1960s when the dual-paste, self-cure composite systems were popular , use of direct polymer-based restorative materials has changed the way dentistry is performed and perceived. The early paste–paste systems, although rivaling their predecessors with respect to appearance and durability, still left many clinicians wanting for a faster process of fabricating tooth-mimicking restorations, where the working time was almost infinite , the setting time was only seconds and not minutes, and where colors were stable and long-term wear allowed successful use in the posterior segment .
2.2
UV-curing
Like many advances in dentistry, the technology for using light to polymerize resin-based materials did not originate within the profession, but instead was an existing technology that was adapted for dental use . The first photocuring units were designed to emit ultraviolet light (about 365 nm) through a quartz rod from a high pressure mercury source and were introduced in the early 1970s . This development was seen as a revolutionary step in dentistry, for it allowed a “cure on demand” feature, which was previously unattainable using the self-curing products. Typical exposure durations were 20 s, but 60 s provided enhanced results . Filled, photocurable composites as well as sealant materials were available , and were used in very innovative ways to not only restore carious processes, but to also repair tooth fractures and provide easily performed esthetic results . The phoinitiating system relied on benzoine ether-type compounds , which broke down into multiple radicals, without need of an intermediary component . The spectral distribution of light sources of the time with the absorption spectrum of the initiator help to correlate these two parameters . Although some of the restorations placed using this early technology have proven to be remarkably successful , in general the procedure was fraught with many issues. First, because of the limited ability of light to penetrate deep within the material, incremental buildups were required instead of bulk placement, and were limited in depth . In addition, concerns were voiced about the potential for harmful effects of the short wavelength energy being exposed to human eyes (corneal burns and cataract formation) as well as possible changes in the oral microflora .
2.3
Visible light curing
Amazingly, only a few years following the introduction of UV radiation for curing dental restoratives, the ability of using visible radiation was introduced: February 24, 1976. On that day, Dr. Mohammed Bassoiuny of the Turner School of Dentistry, Manchester, placed the first visible light-cured composite restoration on Dr. John Yearn, the then head of development of this effort by Imperial Chemical Industries (ICI) of England . Again, development of the chemistry for doing so did not arise from the dental field, but instead adapted from use in the field of polymerizing thin resin films for printing, metal and plastic coatings, and paints . The optimization of a visible light-curing photoinitiation system composition using camphorquinone and a tertiary amine co-initiator was key to the success of this effort, and remains the most popular basic formulation in use today . The unit consisted of a quartz–tungsten–halogen (QTH) source having heat absorbing glass and a bandpass filter allowing only light between 400 and 550 nm to pass: the wavelengths required to activate the photoinitiator, camphorquinone. ICI went on to partner with Johnson and Johnson to introduce the first light-cured resin composite and light: the FotoFil system . The advantages of using visible light were that 2-mm thick increments could typically be placed using from 40 s to 60 s exposure from a QTH source, and that the potential formation of cataracts and oral microflora alteration were minimized . However, direct retinal burning and advancement of macular degeneration were now a potential for ocular damage, as the wavelength needed to initiate the visible-light initiated systems fell directly within the frequencies known to cause immediate, and permanent damage resulting from retinal burning . Thus, practitioners were advised to place a filtering film between their eyes and the curing light to preclude ocular damage, while also allowing high levels of longer wavelengths to pass in order to adequately visualize the treatment field while curing: the so-called “blue-blockers” .
2.4
Quartz–tungsten–halogen lights
The QTH light source was not developed for this purpose, but was, instead, advanced by engineers at General Electric for use in aircraft lights, where small but very bright and durable sources were needed . However, the QTH light became the mainstay of dental light curing for many years, into the late 1990s. During that time advances included a wide range of adaptations for convenience and efficacy. Bulb wattage increased from 35 W up to 100 W for hand-held units , and up to 340 W for table-top models . Output values ranged from an average of 400 to 500 mW/cm 2 up to an extreme of 3000 mW/cm 2 from one unit: The Swiss Master Light, Electro Medical Systems, Nyon, Switzerland. The filtered spectral emission matched the absorption profile of camphorquinone very well . Units were either hand-held (gun style) using a light source within the gun, from which light was emitted to the target through a bundled glass fiber light guide. The gun was connected to a base power unit using a flexible cord containing electrical wires. The adaptation of a curing gun to accept a wide variety of sizes and styles of different light guides fitting with similar focal points lead to the customization of specific types of light delivery to oral locations . The other basic model was a table top unit, which contained a high-Wattage source directing filtered radiation to a flexible glass bundle cord , or a liquid light guide. The QTH sources in the hand-held units lasted from 30 to 50 h , and utilized the halogen cycle to remain clear from tungsten contamination on the inner wall of the quartz envelope . Sources were readily available, easy to install, and relatively inexpensive. Typical exposure duration times to provide uniform properties in a 2-mm thick composite increment ranged from 40 s to 60 s , and controls allowed selected exposure intervals . Units had to be fan-cooled in order to extend the working time of the source and allow the halogen cycle to operate correctly.
2.5
Vital tooth bleaching and introduction of the “alternative initiators”
Vital tooth bleaching was becoming an overnight success in the early 1990s. However, following this type treatment, manufacturers were not able to provide direct esthetic restorative materials that were of high enough value to match the newly bleached teeth. This situation arose because the photoinitiating system of that time only used camphorquinone, which is a bright canary yellow, and photobleaches only slightly upon exposures within clinically relevant times . Thus, after curing, the restoration tended to have a yellow, residual tinge. In order to provide restorative materials of high value, manufacturers resorted to utilizing other photoinitiators that were used for the UV coating and printing industries, which had a small portion of their absorption range within the short, visible light spectrum . These compounds were a class of initiator that directly broke into multiple radicals without need for any co-initiator, and, although they were pale yellow in color, photobleached to clear once utilized . Examples of such compounds are bis(2,3,6-trimethylbenzoyl)-phenylphosphineoxide (Ciba Specialty Chemicals, Inc., Basel, Switzerland), also known as Irgacure 819, and 2,4,6-trimethylbenzoyl-diphenylphosphine oxide (BASF Corporation, Charlotte, NC), commonly referred to as Lucerin ® TPO. Later, an yellowish oily initiator (1-phenylpropane-1,2-dione, PPD), was introduced to help broaden the overall absorption of initiators between 400 nm and 500 nm . When combined with CQ, a synergistic effect was found, allowing the concentration of CQ to be reduced, thus decreasing the yellow residual color following photocuring , however, others found no reduction in yellow . Also, to reduce the time needed to obtain maximal bleaching effect, manufacturers of bleaching agents were advocating exposure of the solutions to bright light in order to heat the components and hasten their breakdown into peroxy radicals. The wavelengths required for this process were short, near the violet and near ultraviolet, and as well as within the blue region. Thus, light curing units now serve a dual-fold function: to photoactivate resin-based restorative materials, and provide an energy source to hasten vital tooth bleaching.
2.6
The argon-ion laser
As is typically the case, the use of the argon laser to photopolymerize acrylics did not arise within the dental community, but was instead a product of industrial development in the coating industry. The argon-ion laser was marketed first to enhance the effects of vital tooth bleaching in Europe, and is still used for that purpose today . However, when first introduced to the United States , only the clinician could operate the unit, and not auxiliary personnel , so the light became more profitable to use as a dental polymerization source. The initial delivery of power from the laser to the tooth was directly through the end of a fiber optic cable. However, due to the divergent nature of that radiation, other methods were developed in an attempt to make a fairly collimated beam of coherent energy, whose target power was not related to the tip-to-tooth distance , as was the case with conventional QTH light guides. The devices proved very effective in providing high physical properties in dental restorative materials , and because of the unit’s tremendous radiant output, some noted that shorter exposure times were needed to provide similar properties as a QTH source , while some did not . The size and footprint of the unit were very large at first , but a single source could be adapted so that one laser could feed multiple operatories using a network of fiber optic cabling . Over time, the unit became much smaller, and could easily fit into an operatory, but because of weight, needed to be on a cart, if moved from room-to-room. However, owing to the high expense (near $5000) of a typical unit, the inability to replace the source by office personnel, and the elevated room temperatures resulting from the device use, this curing system became outdated in a short time. The emission profile of argon-ion lasers marketed in the Unites States eliminated the strongest output at 514 nm for some reason. This wavelength was typically used to provide hemostatis , and was present as a selectable feature on units marketed in Europe. Excluding the 514 nm peak, the major output from dental argon lasers in the US occurred at 488 nm, just on the sharply declining, long wavelength leg of camphorquinone absorption. Other minor emission peaks at 457.9 nm, 468 nm, and 476.5 nm do occur within high CQ absorption levels, and are thus effective in curing, but others emissions are not: 496 nm . High speed pulsing of the light was found to provide enhanced surface and depth conversion over continuous exposure , and some researchers advocated that each brand and shade of composite required an individualized energy delivery for optimal performance . Designs for a portable diode laser for dental light curing have been developed, but to date, no commercial product has arisen .
2.7
Plasma arc lights
Because dental auxiliaries were prohibited from using the argon laser in the US, technology was imported from Europe (French patent #2,305,092, 1976), that had already met with a great amount of success: the plasma-arc curing light. Developed in the mid 1960s , this type source was not initially developed for dental purposes, but instead was used to provide broad-banded radiation for visualization of operating fields (endoscopy and colonoscopy, for examples) as well as for minimally invasive medical procedures. The source consists of two tungsten electrodes separated by a small distance, encased in a high pressure gas-filled chamber, having a synthetic sapphire window through which the light emission was directed from a parabolic reflective surface . A high electrical potential is developed between the two electrodes, which then forms a spark, ionizing the gas, and providing a conductive path (a plasma) between the electrodes. Once the initial spark is established, electronics then adjust the operating current to maintain light generation through a variety of sophisticated feedback system . The gas originally used in dental PAC lights in Europe contained argon, and was extremely high in output, allowing claims of “sub-second” exposures in advertisements to be used to replace conventional 40–60 s exposures using the QTH light. These units had to be highly filtered, as they generated tremendous amounts of infrared light (resulting in heat generation at the target) as well as ultraviolet (having dangerous ozone formation potential). In dental applications, these types of light sources were pulsed and initially developed for curing UV-polymerizable resins, although no commercial unit could be found . They were later adapted for use in visible-light cured products . Thus, the table top unit contained the conventional heat-absorbing glass and visible bandpass filters, but the light emitted after this entered a three-foot-long liquid light guide , which acted as a very long optical filter, eliminating UV and IR radiation, and passing only visible light . The typical output from these types of lights was near 2000 mW/cm 2 , and was broad banded: from 380 to 500 nm, with a naturally occurring peak near 460 nm, where CQ has its optimal absorption. Originally designed in Europe as the Argo HP, claiming the ability to cure composite using “sub-second exposures”, the unit was attempted to be marketed in the US by DMDS, Inc, of Salt Lake City, UT, as the Apollo 9500. It is conjectured that the source in this unit contained argon, which allowed it to emit higher than conventional xenon, but did not last as long. However, issues with licensing led to the US version of the light introduced in August, 1998 as the Apollo 95e . This unit was quite small, and touted provision of adequate exposure times of 3 s as being equivalent to that of a 40 or 60 s exposure from a QTH light. However, the reason why this unit provided no longer continuous exposure than 3 s did not arise from its adequacy of providing sufficient energy to optimally cure a composite within that time, but instead was attributed to the power supply driving the source not containing sophisticated enough feedback to allow continuous output for any longer time. To perform adequately clinically, multiple 3-s exposures were typically required . The unit offered a variety of removable curing tips at the end of the light guide: curing tips: 430 nm or 460 nm for resin curing and 400–500 nm for bleaching. It was from the confusion concerning tip selection for curing specific restorative materials that the confusion started related to the issue of what light will work with which composite or bonding resin. Subsequent PAC units overcame many of the initial shortfalls of the Apollo 95e: broad banded output (not requiring a special bandpass tip end), longer, continuous exposure times, and a general tendency to provide adequate composite curing using a single 10-s exposure .
2.8
High-intensity QTH lights
The QTH light was then competing with PAC units for market share. In order to respond to the new, high output device, manufacturers of QTH lights used different mechanisms to increase the overall output of their units, and claimed they were “equivalent” to a PAC unit with respect to performance. First, source settings were available to “boost” light output over normal values. This mechanism really just drove the filament at a higher voltage, exceeding accepted tolerance values than the bulb manufacturer suggested. Settings in such output modes were no longer than 10 s, as longer exposures would severely degrade the operating lifetime of the source. Another mechanism of increasing output was the development of the “turbo-tip”. This device was a rigid glass fiber bundle, but had the individual fibers drawn while hot, so that the bundle diameter was smaller at the distal, emitting end than at the proximal, receiving end of the guide. By doing so, the same amount of power was present at both ends, but was distributed over a much smaller area at the emitting end, resulting in about a 1.6 times increase in irradiance . This type tip is still widely used in contemporary LED units to help increase overall output values. However, even with both of these features in operation, the output of the QTH did not match that of a typical PAC light of the day . The future for the QTH light is not “bright”, as many governmental agencies are banning this tremendously inefficient incandescent light sources from general usage soon. Thus US government has stipulated deadlines by which incandescent light sources will stopped being marketed: elimination of the 100-W bulb in 2012 and ending with removal of the 40-W source by 2014 .
2.9
Issues resulting from application of high light levels
During this time of application of ever increasing irradiance levels to teeth and gingiva, issues arose related to the application of excessive heat to the target. Clinicians were admonished to direct light output on their thumb nail to sense the level of “heat” evolved from a light. In addition, with such high intensity levels, the PAC lights were now noted to cause a high degree of polymerization stress, as the resin was polymerized so fast that relaxation was not allowed to occur in the polymer network before it became vitrified. To overcome this problem, researchers found that if the light intensity was delivered in such a manner as to control the rate of curing, stress relief would occur by allowing the resin to flow prior to vitrification. It was hoped that in so doing the potential for debonding between the restoration and the tooth would be much lower . In addition, less heat would generate in the target during the restorative process . However, little difference was noted in microleakage studies . Thus arose the concept of “soft-start” polymerization.
When first introduced, this exposure method was added as a selectable feature on QTH lights, and resulted in an initial short (10-s) low-level output near 100 mW/cm 2 , which was followed by an immediate jump to full output power for the remainder of the exposure: step curing . Later changes resulted in a time-based increase of the light initially applied, with the remainder of exposure providing full output: ramp curing . Still another modification was provided as the “pulse-delay” technique, where, for the last composite increment to be cured, a very low level, short duration exposure was given (3 s at 200 mW/cm 2 ), The clinician was advised to treat another patient (for 5–10 min) while the composite was allowed to flow and relieve stress, after which a bolus of higher output power was provided to complete the cure (30 s at 500 mW/cm 2 ) (BISCO VIP). However, the results did not produce any remarkable differences to what was being used conventionally .
An issue with use of this type exposure delivery is the adequacy of energy delivery at the bottom of an increment. Thus, the possible correlation between enhanced marginal adaptation, reduced shrinkage, and lowered curing stress using this curing protocol might have been related to an overall lower extent of cure at the bottom surface . As long as an adequate exposure is provided during the high-output phase of this technique, concerns about under polymerization are not an issue .
Variations of a soft-start feature are still offered on many contemporary light curing units, even LEDs. Comparison of material conversion between conventional 40 s exposure and that when using a ramped soft-start procedure did not indicate any significant different difference , however, contraction strain and the polymerization exotherm were decreased . To remain competitive, manufacturers of PAC lights also attempted to utilize a soft-start mode to reduce the tremendously high stresses and temperature rises developed from their use. However, the operational characteristics of the PAC light do not provide for low irradiant output, as the spark arising from even the minimal voltage needed to maintain its presence produces more irradiance than does a conventional QTH light using regular output. Thus, benefits of soft-start curing with these types of units could not be realized . However, one manufacturer incorporated a modulated level of output during exposure in order to maintain an overall high irradiant output, but also to decrease target temperature .
2.10
Light emitting diodes
The 50th anniversary of the invention of the light emitting diode (LED) is fast approaching . Use of this technology has truly changed our lives, and will continue to do so, as it proves to be an efficient, cost-effective lighting source. These solid state devices rely on the forward-biased energy difference (band gap) between two dissimilar semiconductor substrates (n-type conduction band, and p-type valence band), to determine the wavelength of emitted light . They are much more efficient than previous types of dental light sources, are light weight, and can be easily battery powered for portability. During the middle-to-late 1990s, tremendous strides were made in the video display, optical communications and solid-state lighting industries with the introduction of light emitting diodes (LEDS). At that time, the red laser was used for providing the source of reading optically encoded video discs. Because the wavelength of red is longer than that of blue, the physical size of the hole through which the light had to pass in order to create a digital signal was also large. With development of shorter wavelength, blue LEDs, smaller sized holes were required than with red, and literally many more openings could be placed into the same physical area occupied by fewer and larger holes that transmitted red light. Thus, more digital information could be stored on smaller sized media, and development in LEDs within this wavelength range became a focus of interest .
Blue emissions were developed using indium–gallium–nitride (InGaN) substrates in the early 1990s . This was also a color needed to activate phosphors to emit yellow, enabling the first white-appearing LED in history . It was thus coincidental that the output range of the newly developed blue LEDs fell within the maximal absorption of CQ, enabling the possibility of using blue LEDs as a dental light-curing source. As has been mentioned previously, it did not take long before the blue LED made its way into a dental curing light .
2.11
First generation leD
These lights were first introduced to the commercial market at the end of 2000 , with the introduction of the LUX o MAX light (Akeda Dental A/S, Lystrup, Denmark), however the concept was developed much earlier: 1995 . A typical design of first generation LED curing lights used an assemblage of multiple, individual LED, single-emitting “5 mm lamp” LED elements (each chip providing 30–60 mW) into a focused, axial or planar array , arranged such that the combined output of the luminaire was sufficient enough to provide sufficient energy to activate CQ. Arrays of from seven (Freelight, 3 M/ESPE, St. Paul, MN) to 64 (GC-e-light, GC America, Alsip, IL) were available, but even with those numbers, the extent of radiation was not sufficient to warrant competition with respect to providing decreased exposure time over that of the standard QTH light. In addition, battery technology relied upon use of NiCad cells, which were plagued with poor performance and memory effects. However, because some photo-curable restorative products used only the short wavelength, alternative initiators, the new blue LED lights would not polymerize them, nor would the argon-ion laser. This situation started the great confusion over what light will adequately polymerize which restorative material, a phenomenon that lasts even to this day. Needless to say, the effectiveness of LED lights over that of conventional QTH units took a while to understand, but it had to do with the amount of radiation emitted within the maximal absorption needs of CQ: with blue LEDs providing much more output within this area (450–470 nm) than from a typical QTH light . As a result, it was possible to provide equivalent resin curing in shorter time with an LED light that provided less measurable output than from a longer exposure from a QTH light emitting more total light. In general, however, the overall curing potential of this first-generation LED light was much less than those of conventional, competitive source types of the time .
2.12
Second generation LED
Advances in the LED chip industry in the early 2000s led to the ability of placing multiple emitting die pads on a single chip, greatly increasing overall light output . Again, dental manufacturers incorporated the new 1-W chips into curing lights, developing what is referred to as the “second generation” LED lights. Chip manufacturers were now fabricating chips specifically within the wavelength requirements for dentistry, and labeling them as “dental LED blue”. Luxeon is Philips Lumileds Lighting Company’s trade name for their high-power LEDs which dissipate 1 W or more. At first, there were two types of Luxeon chips used in second generation lights, according to the development of the technology: the 1 W chip (Luxeon LXHL-BRD1 or –MRD1 generating 140 mW output), and the 5 W chip (Luxeon LXHL-PRD5 or –MRD5, generating 600 mW output). Characteristic of these units is the great increase in output power over that of the first generation , with one 5-W chip providing similar luminance as 10–20 of the individual 5-mm types of the first generation. However, a similar wavelength range output persisted as with the first generation, resulting in a continued inability to photocure restorative materials using only short wavelength initiators. Battery technology also improved, and NiMH energy packs became the typical power source. However, because of the generation of tremendously high power in such a small area, the temperature within the chip was now a concern, as too high a temperature would result in permanent chip damage, if not thermostatically cut off prior to that point . Thus, units had heavy metal heat sinks or large metal surfaces to dissipate chip heat . In addition, the return of fans to curing lights was seen – something everyone thought was gone forever. Recently, a 10 W blue LED has become available especially for dental applications (LZ4-00DB10, LedEngin, Inc., Santa Clara, CA, as well as a 15 W unit (LZ4-00CB15, LedEngin, Inc.), and can provide up to 4.2 W and 5.6 W of radiant flux, respectively. With the dramatically increased chip output, second generation LED lights were now reliably able to outperform their commercial competitive curing light types using shorter exposure times .
2.13
Third generation LEDS
In order to enable polymerization of restorative materials utilizing more than only CQ as initiator, curing light manufacturers resorted to providing LED chip sets that emitted more than one wavelength . The first of these units utilized a separate 5 W blue LED central chip surrounded by four low power violet (around 400 nm) LEDs: the Ultralume 5, Ultradent Products, South Jordan, UT. Thus, by combining the output from these two wavelengths, light was provided at wavelengths that were effective for not only CQ, but for the alternative set of initiators as well, creating the equivalent of a “broad banded LED” curing light. Other manufacturers incorporated the violet chips located next to other blue chips within a single LED element: LZ4-00D110, High Efficiency Dental Blue + UV LED Emitter, Led Engin, Inc. with 1 UV die emitting 0.76 W and 3 blue dies each emitting 3 W. This ability to generate multiple wavelengths from a single LED light led a “third generation” of LED dental curing lights. Typically, these units use either NiMH or Li-ion battery technology. One manufacturer currently incorporates three different wavelength chips: VALO, Ultradent Products. Units of this generation are quite effective in providing sufficient irradiance at appropriate wavelengths to be able to polymerize any type of dental restorative material.
2.14
LED construction styles
Contemporary LED lights are either those styled after the old QTH guns, with a turbo-tip fiber optic bundle, or are a pencil-type unit, having the emitting chip set at the distal end of the unit, or a similar fiber optic guide. The lights are either totally driven by mains connection, are battery operated, or both. Those with the chips at the distal end of the unit either have no coverage over the elements, a sealed or removable clear plate covering it, or utilize a fixed lens. Advantages of the pencil style body include the ability to gain access to many more locations in the mouth than possible with the hard, long glass bundled guide, as well as less possibility of breakage, should the unit drop.
2.15
Summary of history
Even with all the advantages that solid state light curing has brought to dentistry, many of the issues correlated with other types of light sources (polymerization shrinkage and stress, intrapulpal temperature rise, and confusion over method of light intensity application) persist at present. Misunderstanding and frustration still exist over compatibilities between lights and restorative resins, as well as the advantages and disadvantages of using curing units with such tremendously high output levels . Even with all the improvements made in photocuring over the last 40 years, after 10 years of service, only 43% of almost 100,000 resin restorations were still in service, with performance described as “comparable only to the worst performing amalgam restoration ”. Only with this background, can the most contemporary findings and issues can be better placed in context of the entire field.
2
Literature
2.1
Abbreviated history of light curing in dentistry
From the late 1940, with power/liquid, self-curing direct, acrylic-based restorative materials, to the late 1960s when the dual-paste, self-cure composite systems were popular , use of direct polymer-based restorative materials has changed the way dentistry is performed and perceived. The early paste–paste systems, although rivaling their predecessors with respect to appearance and durability, still left many clinicians wanting for a faster process of fabricating tooth-mimicking restorations, where the working time was almost infinite , the setting time was only seconds and not minutes, and where colors were stable and long-term wear allowed successful use in the posterior segment .
2.2
UV-curing
Like many advances in dentistry, the technology for using light to polymerize resin-based materials did not originate within the profession, but instead was an existing technology that was adapted for dental use . The first photocuring units were designed to emit ultraviolet light (about 365 nm) through a quartz rod from a high pressure mercury source and were introduced in the early 1970s . This development was seen as a revolutionary step in dentistry, for it allowed a “cure on demand” feature, which was previously unattainable using the self-curing products. Typical exposure durations were 20 s, but 60 s provided enhanced results . Filled, photocurable composites as well as sealant materials were available , and were used in very innovative ways to not only restore carious processes, but to also repair tooth fractures and provide easily performed esthetic results . The phoinitiating system relied on benzoine ether-type compounds , which broke down into multiple radicals, without need of an intermediary component . The spectral distribution of light sources of the time with the absorption spectrum of the initiator help to correlate these two parameters . Although some of the restorations placed using this early technology have proven to be remarkably successful , in general the procedure was fraught with many issues. First, because of the limited ability of light to penetrate deep within the material, incremental buildups were required instead of bulk placement, and were limited in depth . In addition, concerns were voiced about the potential for harmful effects of the short wavelength energy being exposed to human eyes (corneal burns and cataract formation) as well as possible changes in the oral microflora .
2.3
Visible light curing
Amazingly, only a few years following the introduction of UV radiation for curing dental restoratives, the ability of using visible radiation was introduced: February 24, 1976. On that day, Dr. Mohammed Bassoiuny of the Turner School of Dentistry, Manchester, placed the first visible light-cured composite restoration on Dr. John Yearn, the then head of development of this effort by Imperial Chemical Industries (ICI) of England . Again, development of the chemistry for doing so did not arise from the dental field, but instead adapted from use in the field of polymerizing thin resin films for printing, metal and plastic coatings, and paints . The optimization of a visible light-curing photoinitiation system composition using camphorquinone and a tertiary amine co-initiator was key to the success of this effort, and remains the most popular basic formulation in use today . The unit consisted of a quartz–tungsten–halogen (QTH) source having heat absorbing glass and a bandpass filter allowing only light between 400 and 550 nm to pass: the wavelengths required to activate the photoinitiator, camphorquinone. ICI went on to partner with Johnson and Johnson to introduce the first light-cured resin composite and light: the FotoFil system . The advantages of using visible light were that 2-mm thick increments could typically be placed using from 40 s to 60 s exposure from a QTH source, and that the potential formation of cataracts and oral microflora alteration were minimized . However, direct retinal burning and advancement of macular degeneration were now a potential for ocular damage, as the wavelength needed to initiate the visible-light initiated systems fell directly within the frequencies known to cause immediate, and permanent damage resulting from retinal burning . Thus, practitioners were advised to place a filtering film between their eyes and the curing light to preclude ocular damage, while also allowing high levels of longer wavelengths to pass in order to adequately visualize the treatment field while curing: the so-called “blue-blockers” .
2.4
Quartz–tungsten–halogen lights
The QTH light source was not developed for this purpose, but was, instead, advanced by engineers at General Electric for use in aircraft lights, where small but very bright and durable sources were needed . However, the QTH light became the mainstay of dental light curing for many years, into the late 1990s. During that time advances included a wide range of adaptations for convenience and efficacy. Bulb wattage increased from 35 W up to 100 W for hand-held units , and up to 340 W for table-top models . Output values ranged from an average of 400 to 500 mW/cm 2 up to an extreme of 3000 mW/cm 2 from one unit: The Swiss Master Light, Electro Medical Systems, Nyon, Switzerland. The filtered spectral emission matched the absorption profile of camphorquinone very well . Units were either hand-held (gun style) using a light source within the gun, from which light was emitted to the target through a bundled glass fiber light guide. The gun was connected to a base power unit using a flexible cord containing electrical wires. The adaptation of a curing gun to accept a wide variety of sizes and styles of different light guides fitting with similar focal points lead to the customization of specific types of light delivery to oral locations . The other basic model was a table top unit, which contained a high-Wattage source directing filtered radiation to a flexible glass bundle cord , or a liquid light guide. The QTH sources in the hand-held units lasted from 30 to 50 h , and utilized the halogen cycle to remain clear from tungsten contamination on the inner wall of the quartz envelope . Sources were readily available, easy to install, and relatively inexpensive. Typical exposure duration times to provide uniform properties in a 2-mm thick composite increment ranged from 40 s to 60 s , and controls allowed selected exposure intervals . Units had to be fan-cooled in order to extend the working time of the source and allow the halogen cycle to operate correctly.
2.5
Vital tooth bleaching and introduction of the “alternative initiators”
Vital tooth bleaching was becoming an overnight success in the early 1990s. However, following this type treatment, manufacturers were not able to provide direct esthetic restorative materials that were of high enough value to match the newly bleached teeth. This situation arose because the photoinitiating system of that time only used camphorquinone, which is a bright canary yellow, and photobleaches only slightly upon exposures within clinically relevant times . Thus, after curing, the restoration tended to have a yellow, residual tinge. In order to provide restorative materials of high value, manufacturers resorted to utilizing other photoinitiators that were used for the UV coating and printing industries, which had a small portion of their absorption range within the short, visible light spectrum . These compounds were a class of initiator that directly broke into multiple radicals without need for any co-initiator, and, although they were pale yellow in color, photobleached to clear once utilized . Examples of such compounds are bis(2,3,6-trimethylbenzoyl)-phenylphosphineoxide (Ciba Specialty Chemicals, Inc., Basel, Switzerland), also known as Irgacure 819, and 2,4,6-trimethylbenzoyl-diphenylphosphine oxide (BASF Corporation, Charlotte, NC), commonly referred to as Lucerin ® TPO. Later, an yellowish oily initiator (1-phenylpropane-1,2-dione, PPD), was introduced to help broaden the overall absorption of initiators between 400 nm and 500 nm . When combined with CQ, a synergistic effect was found, allowing the concentration of CQ to be reduced, thus decreasing the yellow residual color following photocuring , however, others found no reduction in yellow . Also, to reduce the time needed to obtain maximal bleaching effect, manufacturers of bleaching agents were advocating exposure of the solutions to bright light in order to heat the components and hasten their breakdown into peroxy radicals. The wavelengths required for this process were short, near the violet and near ultraviolet, and as well as within the blue region. Thus, light curing units now serve a dual-fold function: to photoactivate resin-based restorative materials, and provide an energy source to hasten vital tooth bleaching.
2.6
The argon-ion laser
As is typically the case, the use of the argon laser to photopolymerize acrylics did not arise within the dental community, but was instead a product of industrial development in the coating industry. The argon-ion laser was marketed first to enhance the effects of vital tooth bleaching in Europe, and is still used for that purpose today . However, when first introduced to the United States , only the clinician could operate the unit, and not auxiliary personnel , so the light became more profitable to use as a dental polymerization source. The initial delivery of power from the laser to the tooth was directly through the end of a fiber optic cable. However, due to the divergent nature of that radiation, other methods were developed in an attempt to make a fairly collimated beam of coherent energy, whose target power was not related to the tip-to-tooth distance , as was the case with conventional QTH light guides. The devices proved very effective in providing high physical properties in dental restorative materials , and because of the unit’s tremendous radiant output, some noted that shorter exposure times were needed to provide similar properties as a QTH source , while some did not . The size and footprint of the unit were very large at first , but a single source could be adapted so that one laser could feed multiple operatories using a network of fiber optic cabling . Over time, the unit became much smaller, and could easily fit into an operatory, but because of weight, needed to be on a cart, if moved from room-to-room. However, owing to the high expense (near $5000) of a typical unit, the inability to replace the source by office personnel, and the elevated room temperatures resulting from the device use, this curing system became outdated in a short time. The emission profile of argon-ion lasers marketed in the Unites States eliminated the strongest output at 514 nm for some reason. This wavelength was typically used to provide hemostatis , and was present as a selectable feature on units marketed in Europe. Excluding the 514 nm peak, the major output from dental argon lasers in the US occurred at 488 nm, just on the sharply declining, long wavelength leg of camphorquinone absorption. Other minor emission peaks at 457.9 nm, 468 nm, and 476.5 nm do occur within high CQ absorption levels, and are thus effective in curing, but others emissions are not: 496 nm . High speed pulsing of the light was found to provide enhanced surface and depth conversion over continuous exposure , and some researchers advocated that each brand and shade of composite required an individualized energy delivery for optimal performance . Designs for a portable diode laser for dental light curing have been developed, but to date, no commercial product has arisen .
2.7
Plasma arc lights
Because dental auxiliaries were prohibited from using the argon laser in the US, technology was imported from Europe (French patent #2,305,092, 1976), that had already met with a great amount of success: the plasma-arc curing light. Developed in the mid 1960s , this type source was not initially developed for dental purposes, but instead was used to provide broad-banded radiation for visualization of operating fields (endoscopy and colonoscopy, for examples) as well as for minimally invasive medical procedures. The source consists of two tungsten electrodes separated by a small distance, encased in a high pressure gas-filled chamber, having a synthetic sapphire window through which the light emission was directed from a parabolic reflective surface . A high electrical potential is developed between the two electrodes, which then forms a spark, ionizing the gas, and providing a conductive path (a plasma) between the electrodes. Once the initial spark is established, electronics then adjust the operating current to maintain light generation through a variety of sophisticated feedback system . The gas originally used in dental PAC lights in Europe contained argon, and was extremely high in output, allowing claims of “sub-second” exposures in advertisements to be used to replace conventional 40–60 s exposures using the QTH light. These units had to be highly filtered, as they generated tremendous amounts of infrared light (resulting in heat generation at the target) as well as ultraviolet (having dangerous ozone formation potential). In dental applications, these types of light sources were pulsed and initially developed for curing UV-polymerizable resins, although no commercial unit could be found . They were later adapted for use in visible-light cured products . Thus, the table top unit contained the conventional heat-absorbing glass and visible bandpass filters, but the light emitted after this entered a three-foot-long liquid light guide , which acted as a very long optical filter, eliminating UV and IR radiation, and passing only visible light . The typical output from these types of lights was near 2000 mW/cm 2 , and was broad banded: from 380 to 500 nm, with a naturally occurring peak near 460 nm, where CQ has its optimal absorption. Originally designed in Europe as the Argo HP, claiming the ability to cure composite using “sub-second exposures”, the unit was attempted to be marketed in the US by DMDS, Inc, of Salt Lake City, UT, as the Apollo 9500. It is conjectured that the source in this unit contained argon, which allowed it to emit higher than conventional xenon, but did not last as long. However, issues with licensing led to the US version of the light introduced in August, 1998 as the Apollo 95e . This unit was quite small, and touted provision of adequate exposure times of 3 s as being equivalent to that of a 40 or 60 s exposure from a QTH light. However, the reason why this unit provided no longer continuous exposure than 3 s did not arise from its adequacy of providing sufficient energy to optimally cure a composite within that time, but instead was attributed to the power supply driving the source not containing sophisticated enough feedback to allow continuous output for any longer time. To perform adequately clinically, multiple 3-s exposures were typically required . The unit offered a variety of removable curing tips at the end of the light guide: curing tips: 430 nm or 460 nm for resin curing and 400–500 nm for bleaching. It was from the confusion concerning tip selection for curing specific restorative materials that the confusion started related to the issue of what light will work with which composite or bonding resin. Subsequent PAC units overcame many of the initial shortfalls of the Apollo 95e: broad banded output (not requiring a special bandpass tip end), longer, continuous exposure times, and a general tendency to provide adequate composite curing using a single 10-s exposure .
2.8
High-intensity QTH lights
The QTH light was then competing with PAC units for market share. In order to respond to the new, high output device, manufacturers of QTH lights used different mechanisms to increase the overall output of their units, and claimed they were “equivalent” to a PAC unit with respect to performance. First, source settings were available to “boost” light output over normal values. This mechanism really just drove the filament at a higher voltage, exceeding accepted tolerance values than the bulb manufacturer suggested. Settings in such output modes were no longer than 10 s, as longer exposures would severely degrade the operating lifetime of the source. Another mechanism of increasing output was the development of the “turbo-tip”. This device was a rigid glass fiber bundle, but had the individual fibers drawn while hot, so that the bundle diameter was smaller at the distal, emitting end than at the proximal, receiving end of the guide. By doing so, the same amount of power was present at both ends, but was distributed over a much smaller area at the emitting end, resulting in about a 1.6 times increase in irradiance . This type tip is still widely used in contemporary LED units to help increase overall output values. However, even with both of these features in operation, the output of the QTH did not match that of a typical PAC light of the day . The future for the QTH light is not “bright”, as many governmental agencies are banning this tremendously inefficient incandescent light sources from general usage soon. Thus US government has stipulated deadlines by which incandescent light sources will stopped being marketed: elimination of the 100-W bulb in 2012 and ending with removal of the 40-W source by 2014 .
2.9
Issues resulting from application of high light levels
During this time of application of ever increasing irradiance levels to teeth and gingiva, issues arose related to the application of excessive heat to the target. Clinicians were admonished to direct light output on their thumb nail to sense the level of “heat” evolved from a light. In addition, with such high intensity levels, the PAC lights were now noted to cause a high degree of polymerization stress, as the resin was polymerized so fast that relaxation was not allowed to occur in the polymer network before it became vitrified. To overcome this problem, researchers found that if the light intensity was delivered in such a manner as to control the rate of curing, stress relief would occur by allowing the resin to flow prior to vitrification. It was hoped that in so doing the potential for debonding between the restoration and the tooth would be much lower . In addition, less heat would generate in the target during the restorative process . However, little difference was noted in microleakage studies . Thus arose the concept of “soft-start” polymerization.
When first introduced, this exposure method was added as a selectable feature on QTH lights, and resulted in an initial short (10-s) low-level output near 100 mW/cm 2 , which was followed by an immediate jump to full output power for the remainder of the exposure: step curing . Later changes resulted in a time-based increase of the light initially applied, with the remainder of exposure providing full output: ramp curing . Still another modification was provided as the “pulse-delay” technique, where, for the last composite increment to be cured, a very low level, short duration exposure was given (3 s at 200 mW/cm 2 ), The clinician was advised to treat another patient (for 5–10 min) while the composite was allowed to flow and relieve stress, after which a bolus of higher output power was provided to complete the cure (30 s at 500 mW/cm 2 ) (BISCO VIP). However, the results did not produce any remarkable differences to what was being used conventionally .
An issue with use of this type exposure delivery is the adequacy of energy delivery at the bottom of an increment. Thus, the possible correlation between enhanced marginal adaptation, reduced shrinkage, and lowered curing stress using this curing protocol might have been related to an overall lower extent of cure at the bottom surface . As long as an adequate exposure is provided during the high-output phase of this technique, concerns about under polymerization are not an issue .
Variations of a soft-start feature are still offered on many contemporary light curing units, even LEDs. Comparison of material conversion between conventional 40 s exposure and that when using a ramped soft-start procedure did not indicate any significant different difference , however, contraction strain and the polymerization exotherm were decreased . To remain competitive, manufacturers of PAC lights also attempted to utilize a soft-start mode to reduce the tremendously high stresses and temperature rises developed from their use. However, the operational characteristics of the PAC light do not provide for low irradiant output, as the spark arising from even the minimal voltage needed to maintain its presence produces more irradiance than does a conventional QTH light using regular output. Thus, benefits of soft-start curing with these types of units could not be realized . However, one manufacturer incorporated a modulated level of output during exposure in order to maintain an overall high irradiant output, but also to decrease target temperature .
2.10
Light emitting diodes
The 50th anniversary of the invention of the light emitting diode (LED) is fast approaching . Use of this technology has truly changed our lives, and will continue to do so, as it proves to be an efficient, cost-effective lighting source. These solid state devices rely on the forward-biased energy difference (band gap) between two dissimilar semiconductor substrates (n-type conduction band, and p-type valence band), to determine the wavelength of emitted light . They are much more efficient than previous types of dental light sources, are light weight, and can be easily battery powered for portability. During the middle-to-late 1990s, tremendous strides were made in the video display, optical communications and solid-state lighting industries with the introduction of light emitting diodes (LEDS). At that time, the red laser was used for providing the source of reading optically encoded video discs. Because the wavelength of red is longer than that of blue, the physical size of the hole through which the light had to pass in order to create a digital signal was also large. With development of shorter wavelength, blue LEDs, smaller sized holes were required than with red, and literally many more openings could be placed into the same physical area occupied by fewer and larger holes that transmitted red light. Thus, more digital information could be stored on smaller sized media, and development in LEDs within this wavelength range became a focus of interest .
Blue emissions were developed using indium–gallium–nitride (InGaN) substrates in the early 1990s . This was also a color needed to activate phosphors to emit yellow, enabling the first white-appearing LED in history . It was thus coincidental that the output range of the newly developed blue LEDs fell within the maximal absorption of CQ, enabling the possibility of using blue LEDs as a dental light-curing source. As has been mentioned previously, it did not take long before the blue LED made its way into a dental curing light .
2.11
First generation leD
These lights were first introduced to the commercial market at the end of 2000 , with the introduction of the LUX o MAX light (Akeda Dental A/S, Lystrup, Denmark), however the concept was developed much earlier: 1995 . A typical design of first generation LED curing lights used an assemblage of multiple, individual LED, single-emitting “5 mm lamp” LED elements (each chip providing 30–60 mW) into a focused, axial or planar array , arranged such that the combined output of the luminaire was sufficient enough to provide sufficient energy to activate CQ. Arrays of from seven (Freelight, 3 M/ESPE, St. Paul, MN) to 64 (GC-e-light, GC America, Alsip, IL) were available, but even with those numbers, the extent of radiation was not sufficient to warrant competition with respect to providing decreased exposure time over that of the standard QTH light. In addition, battery technology relied upon use of NiCad cells, which were plagued with poor performance and memory effects. However, because some photo-curable restorative products used only the short wavelength, alternative initiators, the new blue LED lights would not polymerize them, nor would the argon-ion laser. This situation started the great confusion over what light will adequately polymerize which restorative material, a phenomenon that lasts even to this day. Needless to say, the effectiveness of LED lights over that of conventional QTH units took a while to understand, but it had to do with the amount of radiation emitted within the maximal absorption needs of CQ: with blue LEDs providing much more output within this area (450–470 nm) than from a typical QTH light . As a result, it was possible to provide equivalent resin curing in shorter time with an LED light that provided less measurable output than from a longer exposure from a QTH light emitting more total light. In general, however, the overall curing potential of this first-generation LED light was much less than those of conventional, competitive source types of the time .
2.12
Second generation LED
Advances in the LED chip industry in the early 2000s led to the ability of placing multiple emitting die pads on a single chip, greatly increasing overall light output . Again, dental manufacturers incorporated the new 1-W chips into curing lights, developing what is referred to as the “second generation” LED lights. Chip manufacturers were now fabricating chips specifically within the wavelength requirements for dentistry, and labeling them as “dental LED blue”. Luxeon is Philips Lumileds Lighting Company’s trade name for their high-power LEDs which dissipate 1 W or more. At first, there were two types of Luxeon chips used in second generation lights, according to the development of the technology: the 1 W chip (Luxeon LXHL-BRD1 or –MRD1 generating 140 mW output), and the 5 W chip (Luxeon LXHL-PRD5 or –MRD5, generating 600 mW output). Characteristic of these units is the great increase in output power over that of the first generation , with one 5-W chip providing similar luminance as 10–20 of the individual 5-mm types of the first generation. However, a similar wavelength range output persisted as with the first generation, resulting in a continued inability to photocure restorative materials using only short wavelength initiators. Battery technology also improved, and NiMH energy packs became the typical power source. However, because of the generation of tremendously high power in such a small area, the temperature within the chip was now a concern, as too high a temperature would result in permanent chip damage, if not thermostatically cut off prior to that point . Thus, units had heavy metal heat sinks or large metal surfaces to dissipate chip heat . In addition, the return of fans to curing lights was seen – something everyone thought was gone forever. Recently, a 10 W blue LED has become available especially for dental applications (LZ4-00DB10, LedEngin, Inc., Santa Clara, CA, as well as a 15 W unit (LZ4-00CB15, LedEngin, Inc.), and can provide up to 4.2 W and 5.6 W of radiant flux, respectively. With the dramatically increased chip output, second generation LED lights were now reliably able to outperform their commercial competitive curing light types using shorter exposure times .
2.13
Third generation LEDS
In order to enable polymerization of restorative materials utilizing more than only CQ as initiator, curing light manufacturers resorted to providing LED chip sets that emitted more than one wavelength . The first of these units utilized a separate 5 W blue LED central chip surrounded by four low power violet (around 400 nm) LEDs: the Ultralume 5, Ultradent Products, South Jordan, UT. Thus, by combining the output from these two wavelengths, light was provided at wavelengths that were effective for not only CQ, but for the alternative set of initiators as well, creating the equivalent of a “broad banded LED” curing light. Other manufacturers incorporated the violet chips located next to other blue chips within a single LED element: LZ4-00D110, High Efficiency Dental Blue + UV LED Emitter, Led Engin, Inc. with 1 UV die emitting 0.76 W and 3 blue dies each emitting 3 W. This ability to generate multiple wavelengths from a single LED light led a “third generation” of LED dental curing lights. Typically, these units use either NiMH or Li-ion battery technology. One manufacturer currently incorporates three different wavelength chips: VALO, Ultradent Products. Units of this generation are quite effective in providing sufficient irradiance at appropriate wavelengths to be able to polymerize any type of dental restorative material.
2.14
LED construction styles
Contemporary LED lights are either those styled after the old QTH guns, with a turbo-tip fiber optic bundle, or are a pencil-type unit, having the emitting chip set at the distal end of the unit, or a similar fiber optic guide. The lights are either totally driven by mains connection, are battery operated, or both. Those with the chips at the distal end of the unit either have no coverage over the elements, a sealed or removable clear plate covering it, or utilize a fixed lens. Advantages of the pencil style body include the ability to gain access to many more locations in the mouth than possible with the hard, long glass bundled guide, as well as less possibility of breakage, should the unit drop.
2.15
Summary of history
Even with all the advantages that solid state light curing has brought to dentistry, many of the issues correlated with other types of light sources (polymerization shrinkage and stress, intrapulpal temperature rise, and confusion over method of light intensity application) persist at present. Misunderstanding and frustration still exist over compatibilities between lights and restorative resins, as well as the advantages and disadvantages of using curing units with such tremendously high output levels . Even with all the improvements made in photocuring over the last 40 years, after 10 years of service, only 43% of almost 100,000 resin restorations were still in service, with performance described as “comparable only to the worst performing amalgam restoration ”. Only with this background, can the most contemporary findings and issues can be better placed in context of the entire field.
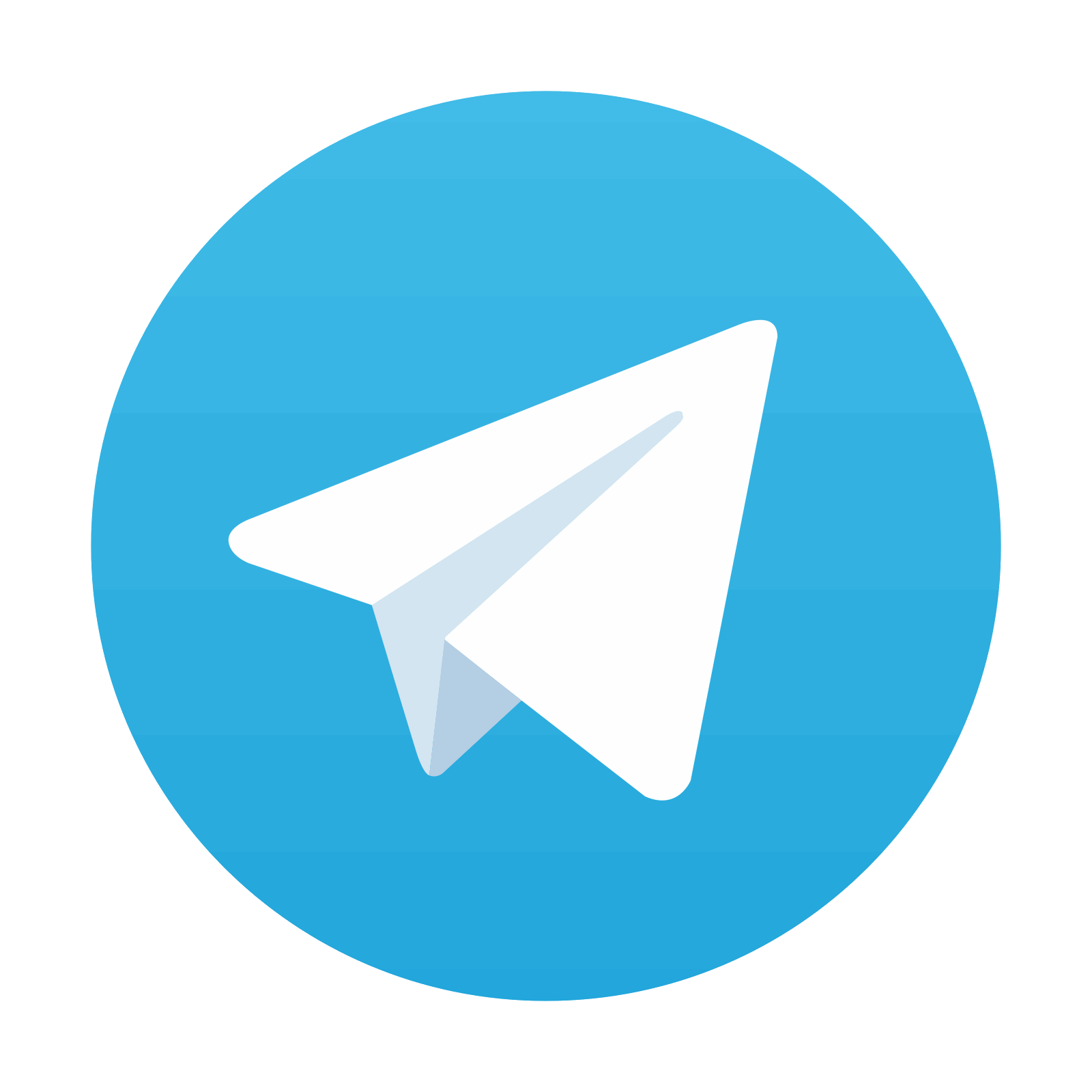
Stay updated, free dental videos. Join our Telegram channel

VIDEdental - Online dental courses
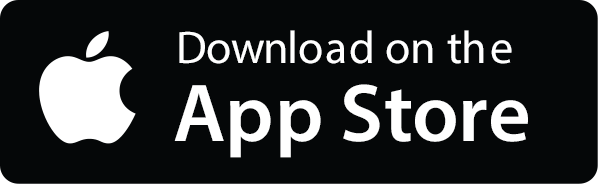
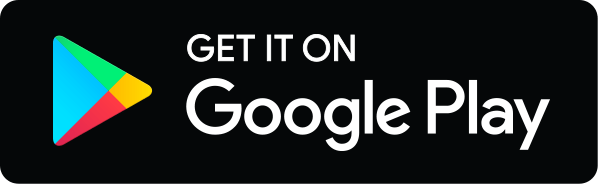