Abstract
The combined resection of skull-infiltrating tumours and immediate cranioplastic reconstruction predominantly relies on freehand-moulded solutions. Techniques that enable this procedure to be performed easily in routine clinical practice would be useful. A cadaveric study was developed in which a new software tool was used to perform single-stage reconstructions with prefabricated implants after the resection of skull-infiltrating pathologies. A novel 3D visualization and interaction framework was developed to create 10 virtual craniotomies in five cadaveric specimens. Polyether ether ketone (PEEK) implants were manufactured according to the bone defects. The image-guided craniotomy was reconstructed with PEEK and compared to polymethyl methacrylate (PMMA). Navigational accuracy and surgical precision were assessed. The PEEK workflow resulted in up to 10-fold shorter reconstruction times than the standard technique. Surgical precision was reflected by the mean 1.1 ± 0.29 mm distance between the virtual and real craniotomy, with submillimetre precision in 50%. Assessment of the global offset between virtual and actual craniotomy revealed an average shift of 4.5 ± 3.6 mm. The results validated the ‘elective single-stage cranioplasty’ technique as a state-of-the-art virtual planning method and surgical workflow. This patient-tailored workflow could significantly reduce surgical times compared to the traditional, intraoperative acrylic moulding method and may be an option for the reconstruction of bone defects in the craniofacial region.
Bone resection is often required during neurosurgical or craniofacial procedures for tumours or traumatic brain injuries, with a relatively high prevalence worldwide . In some cases, the patient’s autologous bone material cannot be used for reconstruction. Therefore alloplastic materials are frequently necessary, and these have to be adapted intraoperatively. This procedure extends the surgery time and often results in cranial asymmetry. Hence, implants for reconstruction that can be produced quickly and efficiently are urgently needed in order to reduce surgery times and improve aesthetics.
The reconstruction of craniofacial bone defects with alloplastic implants based on computer-aided design/computer-aided manufacturing (CAD/CAM) techniques was introduced in the 1990s . Furthermore, prefabricated implants based on materials such as polyether ether ketone (PEEK) have been used as an alternative to discarded autologous bone grafts . However, in cases of technically demanding reconstruction after the resection of skull-infiltrating tumours, non-porous allografts such as hand-moulded polymethyl methacrylate (PMMA) are still often used. Current research efforts point towards prefabricated solutions to avoid the adverse events associated with intraoperatively moulded acrylics, such as thermal necrosis, material fracture, infections, and often unsatisfactory cosmetic results .
Single-stage reconstructive methods are subdivided into two categories: (1) preoperative template moulding, allowing the intraoperative creation of PMMA-based patient-specific implants; and (2) CAD/CAM-assisted prefabrication of the implant. These techniques offer superior cosmetic results compared to the traditional method of manually shaped bone cement . The published workflows often have an experimental character and are still lacking implementation in clinical routine . With both methods, physicians have to provide the implant manufacturer with standard imaging datasets ahead of surgery. Thereafter, company designers create a virtual bone defect according to the surgeon’s specifications, fabricate an implant, and send it to the hospital.
In this study, a dedicated software program was developed to optimize intraoperative reconstruction workflows with prefabricated implants. The intention was to empower surgeons to simulate the virtual craniotomy independently using commercially available hardware and to combine these modified datasets with an intraoperative navigation system during the procedure. To validate both the software and the operative workflow, a cadaveric feasibility study was performed, termed the Elective Single-stage Cranioplasty Study 1 (ELESC-1). Further, the intention of the study was to assess the accuracy and time-efficacy of this novel method in direct comparison to the traditional acrylic method in craniofacial reconstruction.
Methods
Ethical approval was obtained from the institutional ethics committee (EK No. 1941/2015). The cadaveric study was conducted in the training laboratories of the Department of Neurosurgery, Medical University of Vienna with industrial support (the PEEK implants and osteosynthesis material were provided by DePuy Synthes GmbH, Oberdorf, Switzerland).
Reference cases and cadaveric specimens
Three-dimensional volume-rendering models (3D-VR) of 10 representative clinical cases were selected as reference models (Supplementary Material Table S1). These patients had been treated for skull infiltrating pathologies between 2013 and 2015 by combined resection and large single-stage reconstruction with PMMA. For the study, high-resolution computed tomography (CT) scans were performed on five cadaveric head specimens provided by the Centre of Anatomy and Cell Biology, Medical University of Vienna. Each cadaveric specimen was matched with two clinical model cases ( Fig. 1 , Supplementary Material Table S1).

Virtual planning and implant manufacturing
The location of the intended craniotomy was virtually defined on the cadaver skull CT; the extent and shape were virtually delineated. For this purpose, a 3D medical visualization and analytic framework was developed by senior computer researchers. The software automatically performs bone segmentation and displays the cranial anatomy. The user marks points along the outline of the desired bone flap on the surface of the virtual skull ( Fig. 2 ). The curve is updated after each user interaction, which allows the neurosurgeon to refine the planned outline. Once the desired outline is achieved, a virtual craniotomy is created by removing the marked region from the skull ( Fig. 1 ). This system supports the specification of a slant angle, which allows the creation of virtual craniotomies with tapered rims ( Fig. 2 , Supplementary Material Video S1). The framework integrates seamlessly into current industrial workflows, since both the input and output are DICOM datasets (see Supplementary Material Table S2 for an overview of software characteristics, equipment used, and implants). The modified datasets were sent to the implant manufacturer and served as the basis for industrial designers to plan 10 PEEK implants. As a control, the authors received rotatable 3D images of the virtual implants in situ for surgeon approval. The final designs were transmitted to the production line for the fabrication of the actual implants.

Operative workflow and comparison with PMMA
The original and modified DICOM datasets were imported into the neuronavigation system (StealthStation 7; Medtronic Surgical Technologies, Jacksonville, FL, USA) and merged. A 3D model, demonstrating the delineation of the virtual craniotomy, was reconstructed to support faster methylene blue marking of the craniotomy delineation. After fixation of the specimen in the Mayfield head holder, neuronavigational registration was performed using the combined point-merged anatomic/surface-merged registration method. The skin incision and exact craniotomy boundaries were marked according to the delineations of the virtual craniotomy. Bone reconstruction was performed by PEEK implant insertion and fixation with sutures (cases 1–4) or titanium microfixation materials (available at a later experimental stage for cases 5–10; Fig. 2 C and Supplementary Material Video S1). Once the first PEEK case had been concluded, the cadaveric head was repositioned and fixated, and the second craniotomy and reconstruction were performed according to the same protocol. A postoperative CT scan of the head specimen was then obtained, following which both PEEK implants were removed. The same osteoclastic defects were subsequently reconstructed with PMMA. Finally, a second CT scan was performed. The surgical times of the two reconstruction techniques were evaluated.
Postoperative image assessment and statistical analysis
The viability and precision of the method was evaluated through comparison of the outline of the bone defects on pre- and postoperative imaging. A special-purpose MATLAB script (MathWorks Inc., Natick, MA, USA) was developed to compute distance measurements between the bone flap outlines (Supplementary Material Table S2). 3D points were marked on the outline of the craniotomy in both the preoperative and postoperative imaging data using the OsiriX DICOM viewer (Pixmeo, Geneva, Switzerland). Operating on these points, our program performs several steps: (1) points are reordered along a one-dimensional outline curve, (2) which is fitted with a continuous curve, (3) from which a dense set of points on the outline are generated; (4) the dense point sets of the preoperative and postoperative data are matched to each other via a rigid transformation, thus describing the alignment between the virtual and real craniotomy; (5) the closest preoperative point is determined for each postoperative point on the outline. The respective distances were collected into a histogram from which the mean and standard deviation were computed.
Statistical calculations included descriptive analyses (mean ± standard deviation values). Differences between the groups were evaluated using the Mann–Whitney U -test and by Wilcoxon test for paired samples. Two-sided P -values of <0.05 were considered statistically significant. IBM SPSS Statistics version 23.0 (IBM Corp., Armonk, NY USA) was used for data administration and statistical calculations.
Results
Novel CT data computations on cadavers closely simulated patient cranioplasty
Ten representative clinical cases were selected that had previously been treated with large single-stage acrylic cranioplastic reconstructions. An equivalent number of 3D models were built based on postoperative CT, and the same approaches were applied to five cadaveric heads. CT data derived from the cadavers were inputted into a novel algorithm, which performed bone segmentation and displayed the cranial anatomy.
The algorithm was evaluated by superimposing the image-based data of clinical cases and cadaveric simulations in the DICOM viewer OsiriX. A close inspection of the superimposed surgical boundaries in the patient cases and the cadaveric study showed a close agreement ( Fig. 1 , right column). The planning time for the delineation and segmentation of the virtual approaches was an average of 4.7 ± 1.6 min (range 2.2–7.2 min).
PEEK implant fabrication workflow
The evaluation of the novel, software-based workflow showed that the surgeon-modified datasets were compatible with current industrial implant design and fabricating workflows ( Fig. 2 ).
For the implant manufacturer, the virtual craniotomy in the exported CT datasets appeared nearly identical to a real craniectomy. Manufacturer input helped the investigators define the optimal gap between the implant and the bone edge. This safety margin, initially set at 2 mm, could be reduced to 1 mm in the last six cases due to the satisfactory initial results. No interaction with the technical designers was necessary for the final emplacement of the intended virtual trephination.
Evaluation of surgical precision and navigational accuracy
The navigational accuracy of the craniotomy performed and the surgical precision were evaluated. Independent analysis was conducted through comparison of the overlaid preoperative and postoperative imaging datasets of the cadaveric heads, including the alloplastic implants. The most relevant metrics for the outlines of the virtual and real craniotomy were their location, size, and shape. While the former attribute describes the accuracy of the method, the size and shape define its ‘hand-made’ precision ( Fig. 3 ).

The results of the surgical precision were reflected in the mean local distance between virtual and real craniotomies of 1.1 ± 0.29 mm (range 0.7–1.6 mm). Submillimetre precision could be achieved in 50% of the cadaveric cases ( Fig. 4 ). The assessment of the global offset between virtual and actual craniotomy revealed an average shift of 4.5 ± 3.6 mm (range 1.1–13.1 mm). The six cases operated on in a supine position demonstrated less shift, with a mean global offset of 3.0 ± 1.1 mm (range 1.5–4.7 mm), than the four cases operated on in a prone position, with a mean global offset of 6.2 ± 5.2 mm (range 1.1–13.1 mm). Although this finding did not reach statistical significance ( P = 0.173), a trend towards reduced navigational accuracy for (sub-)occipitally located implantation sites was seen ( Fig. 3 , case 4).
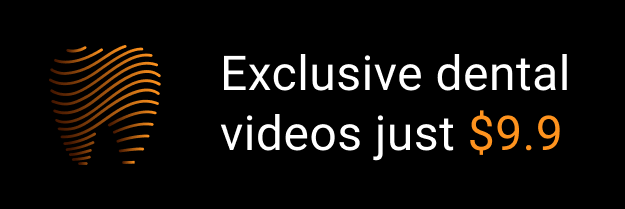