Abstract
Dental personnel often come into contact with composite dust upon polishing or grinding composites. Contemporary composites typically contain high amounts of (silica) nano-filler, but so far it has never been investigated whether composite dust may be hazardous.
Objectives
The objectives of this study were (1) to characterize composite dust in vitro and (2) to assess the clinical exposure.
Methods
Polymerized blocks of contemporary composites were ground with a diamond bur according to a clinically relevant protocol, and aerosolized dust was collected on 1-μm pore-size filters and gravimetrically quantified. In addition, the dust was characterized by transmission electron microscopy. Respirable dust was quantified with a mass particle counter in a dental operatory.
Results
All composites released respirable dust (<5 μm) in vitro. These observations were corroborated by the clinical measurements; however only short episodes of high concentrations of respirable dust upon polishing composites could be observed. Electron microscopic analysis showed that the size of the dust varied widely with particles larger than 10 μm, but submicron and even nano-sized particles could also be observed. The dust particles often consisted of multiple filler particles contained in resin, but single nano-filler particles could also frequently be distinguished.
Significance
This study showed that inhalation of composite dust is better avoided. Therefore, it is recommended to always use water-cooling upon polishing or removing composites, to use good aspiration, to frequently ventilate the dental operatory and to wear masks with high particle-filtration efficiency for small particle sizes.
1
Introduction
Tooth tissue lost due to trauma or caries is nowadays preferentially replaced with composite. Dental composites typically contain (photo-)polymerizable resins and inorganic filler particles that are functionalized with a silane coupling agent to attach them to the resin matrix . The size of the filler particles varies widely, but contemporary composites may contain large quantities of sub-micron and even nano-particles. Typically, 40-nm-sized pyrogenic silica (‘microfill’) is very often used . The most recent class of composites is coined ‘nano-composites’, as they contain larger amounts of amorphous nano-particles (<100 nm) than traditional ‘hybrid composites’. Beside pyrogenic silica, they also contain filler produced in a sol–gel process, which are typically composed of mixed oxides, like ZrO 2 –SiO 2 , and which can be added to a greater extent without overly increasing the viscosity of the unpolymerized composite.
Like in other fields of industry involving nano-technology , some dental researchers and practitioners have expressed concerns that there may be health risks connected to the use of these nano-particles . Dental personnel may inhale aerosolized composite dust while shaping and polishing new composite restorations, and upon removal of old composite restorations . Especially in case of inadequate aspiration and/or lack of assistance, dental professionals (and patients) may be exposed to composite dust. In general, dust smaller than 5 μm and larger than 0.01 μm may be respired and can penetrate deep into the alveolar region of the lungs, beyond the body’s natural mechanisms of cilia and mucous clearing . Chronic inhalation of respirable dust (<5 μm) and nano-particles may provoke both local and systemic toxicity . Excessive and long-term exposure to respirable dusts can induce pneumoconiosis , a chronic lung condition marked by nodular fibrosis. Nano-particles (<100 nm) may also be absorbed in the blood or the lymphatic system resulting in systemic toxicity .
Two decades ago, Collard et al. showed that respirable crystalline silica could be released upon abrading traditional quartz-containing composites (which are now no longer commonly used) . So far, the potential of contemporary composites to release respirable (nano-)dust has never been explored.
The objective of this study was to assess the potential of contemporary composites including nano-composites to release respirable dust. The null hypothesis to be tested was that no potentially harmful respirable dust is released upon grinding. The potential of dental composites to release respirable dust upon grinding was investigated by measurements in both an in vitro and a clinical setting, and the composite dust was characterized by electron microscopy.
2
Materials and methods
Seven commercial composites (1 hybrid, 1 micro-hybrid, one nano-composite and 4 nano-hybrid composites) were included in this study. Their compositions and classifications (including definitions of the classifications) are given in Table 1 .
Composite | Manufacturer | Classification | Resin matrix | Filler | Filler loading |
---|---|---|---|---|---|
Filtek Supreme XT | 3M ESPE, Seefeld, Germany | Nano-composite | BisGMA, Bis-EMA, UDMA, TEGDMA | • SiO 2 (20 nm) • Zirconia-silica clusters (0.6–1.4 μm) with primary particles of 5–20 nm |
78.5 wt% 59.5 vol% |
Premise Enamel | Kerr, Orange, CA, USA | Nano-hybrid composite | Bis-EMA TEGDMA |
• SiO 2 (20 nm) • Barium silicate glass (0.4 μm) • Prepolymerized fillers with barium silicate glass (30–50 μm) |
84 wt% 70 vol% |
Ceram.X duo+ | Dentply DeTrey, Konstanz, Germany | Nano-hybrid composite | Dimethacrylate resin | • Barium boron alumino silicate glass (0.7 μm) • Methacrylate functionalized SiO 2 nano-filler (10 nm) • Methacrylate modified siloxane particles (organically modified ceramic) (2–3 nm) |
77 wt% 55 vol% 12 wt% |
Tetric EvoCeram | Ivoclar-Vivadent, Schaan, Liechtenstein | Nano-hybrid composite | Bis-GMA, UDMA, Bis-EMA | • Barium alumino silicate glass (0.6 μm) • Ytterbium trifluoride a • Mixed oxide (160 nm) • Prepolymerized filler a |
82–83 wt% 66–67 vol% |
Herculite XRV Ultra | Kerr, Orange, CA, USA | Nano-hybrid composite | BisGMA TEGDMA BisEMA |
SiO 2 (20–50 nm) • Barium silicate glass (0,4 μm) • Prepolymerized filler with barium silicate glass and silica (30–50 μm) |
71 wt% 54 vol% |
Gradia Direct | GC, Tokyo, Japan | Micro-filled hybrid composite | UDMA | • Silica prepolymerized filler (16 μm particles with 16 nm pyrogenic silica) • Silicate glass (850 nm) • Pyrogenic silica (16 nm) |
73 wt% 64 vol% |
Z100 MP | 3M ESPE, Seefeld, Germany | Hybrid composite | BisGMA, TEGDMA | • Zirconia silica (0.01 μm to 3.5 μm) | 84.5 wt% 66 vol% |
a Information on the particle-size not provided by the manufacturer.
For each composite, five standardized composite blocks with a size of 15 mm × 13 mm × 3 mm were prepared in a silicon mold according to a clinically relevant procedure. Each block weighed approximately 1 g, which corresponded to the size of a molar-crown . The composite was covered with a glass plate and light-cured on each side for 40 s with a light-curing unit (L.E. Demetron, Kerr, Orange, CA, USA) with an output above 1000 mW/cm 2 .
2.1
Gravimetrical analysis of supra-micron (>1 μm) respirable dust
Entire blocks of composite were ground in vitro using a dental bur, and the composite dust was collected using an IOM personal inhalable aerosol sampler (225-70A, SKC, Eighty Four, PA, USA) and quantified gravimetrically. The IOM sampler was connected to a personal sampling pump (SP 350 sidepack personal sampling pump, TSI, Shoreview, MN, USA). The IOM sampler contained a cassette in which two filters were fixed: (1) a polyurethane foam disc (Multidust foam disc, 225–772 SKC) and (2) a glass-fiber filter with a pore size of 1 μm (225–702 SKC). While the large inhalable size particles (5–10 μm) are deposited on the foam, the smaller respirable (<5 μm) particles pass the foam and are deposited on the glass fiber filter. The filters were acclimatized overnight. Before the experiment, the glass-fiber filters fixed in the cassette were carefully weighed with a microbalance (ME, Sartorius, Göttingen, Germany). The air flow of the pump (4 l/min) was calibrated with the Gilibrator-2 (Gilian, Sensidyne, Schauenburg International GmbH, Mülheim, Germany) .
The experiment was carried out in a custom-made well-sealed plexiglass box (27 mm × 27 mm × 42 mm) with two openings at the sides sealed with disposable gloves, enabling manipulation of the specimens and the bur ( Fig. 1 ). The composite block was held with a forceps during grinding with a rough diamond bur (842314014 Komet, Lemgo, Germany, grain size 100 μm) in a Kavo Intracompact handpiece (200,000 rpm) connected to an electric micromotor (EWL K9, Kavo, Biberach, Germany) and the entire block was ground ensuring that exactly the same amount of composite was ground for each block. The pump was started right before the composite was ground, and left on for 10 min. For each composite block, a new bur was used. After grinding each block of composite, the foam discs were carefully removed and the cassette with the glass-fiber filters was weighed. The weight increase due to respirable dust trapped on the glass-fiber filter was calculated. As the weight of the filters may vary according to the air humidity, the room temperature and humidity were checked not to differ for more than 5%. This experiment was done with five blocks ( n = 5) per composite. As control, 5 measurements were performed following exactly the same test set-up, but without grinding composite. One-way ANOVA and planned comparison of the least squares means at a significance level of 0.05 was carried out to compare the release of dust with the control group.
2.2
Micromorphological characterization of composite dust particles
Composite dust as obtained as described above was mixed with epoxy resin, after which ultra-thin sections (60–90 nm) were prepared with a diamond knife (Diatome, Bienne, Switzerland) in an ultra-microtome (Ultracut UCT, Leica, Vienna, Austria). Subsequently, composite dust particles were characterized by transmission electron microscopy (TEM) (JEOL JEM-1200 EX-II, Tokyo, Japan).
2.3
Semi-quantitative analysis of sub-micron (<1 μm) composite dust
Composite dust was directly collected on a formvar-film-coated copper mesh grid by sampling the composite dust deposited on the box floor (30 min after grinding) and analyzed by TEM (JEOL JEM-1200 EX-II, JEOL, Tokyo, Japan). High resolution photomicrographs of non-embedded dust were used for semi-quantitative analysis. For each grid hole, a very large (7800 × 7800 px), high-resolution (1.8 × 10 6 dpi) photomicrograph was generated out of 25 smaller (2048 × 2048 px) high-resolution pictures. Dust particles as small as 14 nm could be characterized. By means of an image-processing and analysis tool (ImageJ, National Institutes of Health (NIH), Rockville Pike, Bethesda, MD, USA ), dust particles were quantified and their sizes were measured. The minimal Feret distance ( Fig. 2 ) was taken as a measure for their size. Since the minimal Feret distance represents the smallest distance between any two opposing planes tangential to the particle’s boundary, it determines the risk of the particle entering deep into the respiratory system. Per composite, four grid holes were evaluated on different grids ( n = 4), and all particles were counted to make sure that at least 200 particles larger than 1 μm were counted (in total at least 1600 particles were counted for each composite). Kruskal–Wallis’ statistics ( α = 0.05) were run to compare the amount of dust released by the different composites.
2.4
Clinical measurements
The Aerocet-531 Mass Particle Counter (Met One Instruments Inc, Shanghai, China), which utilizes a laser diode, was used to determine the release of dust in a university dental operatory in real-time. Its resolution was 0.5 μm. The sampler was attached to the collar of the dentist, and the device registered the concentrations (in mg/m 3 ) of PM1 (‘particulate matter ≤1 μm’), PM2.5 (‘particulate matter < 2.5 μm’), PM7 (‘particulate matter < 7 μm’) and PM10 (‘particulate matter < 10 μm’) per time intervals of 2 min. Measurements were performed during extensive restorative/esthetic treatments with the nano-composite Filtek Supreme XTE (3M ESPE), like veneering of the front teeth. Final contouring and finishing of the composite restorations was standardly performed by a diamond bur (roughness of 100 μm) without water cooling and high-flow suctioning (which is the standard protocol in our university operatory), while Sof-Lex polishing disks (coarse, medium, fine and ultrafine grid, 3M ESPE, Seefeld, Germany), rubber points (Eve, Ernst Vetter, Pforzhein, Germany) and polishing paste (Prisma Gloss, Densply Detrey, Konstanz, Germany) were used for polishing. As control, measurements were done in an administrative office in the same building at the same moment. Since the results were expressed as mass concentrations, we estimated the amount of particles with a diameter between s 1 and s 2 using following approximation (based on the assumption that the particles are spherical):
n ( [ s 1 , s 2 ] ) = 6 π ρ 4 a 4 s + 3 a 3 ln s − 2 a 2 s − a 1 2 s 2 s 1 s 2
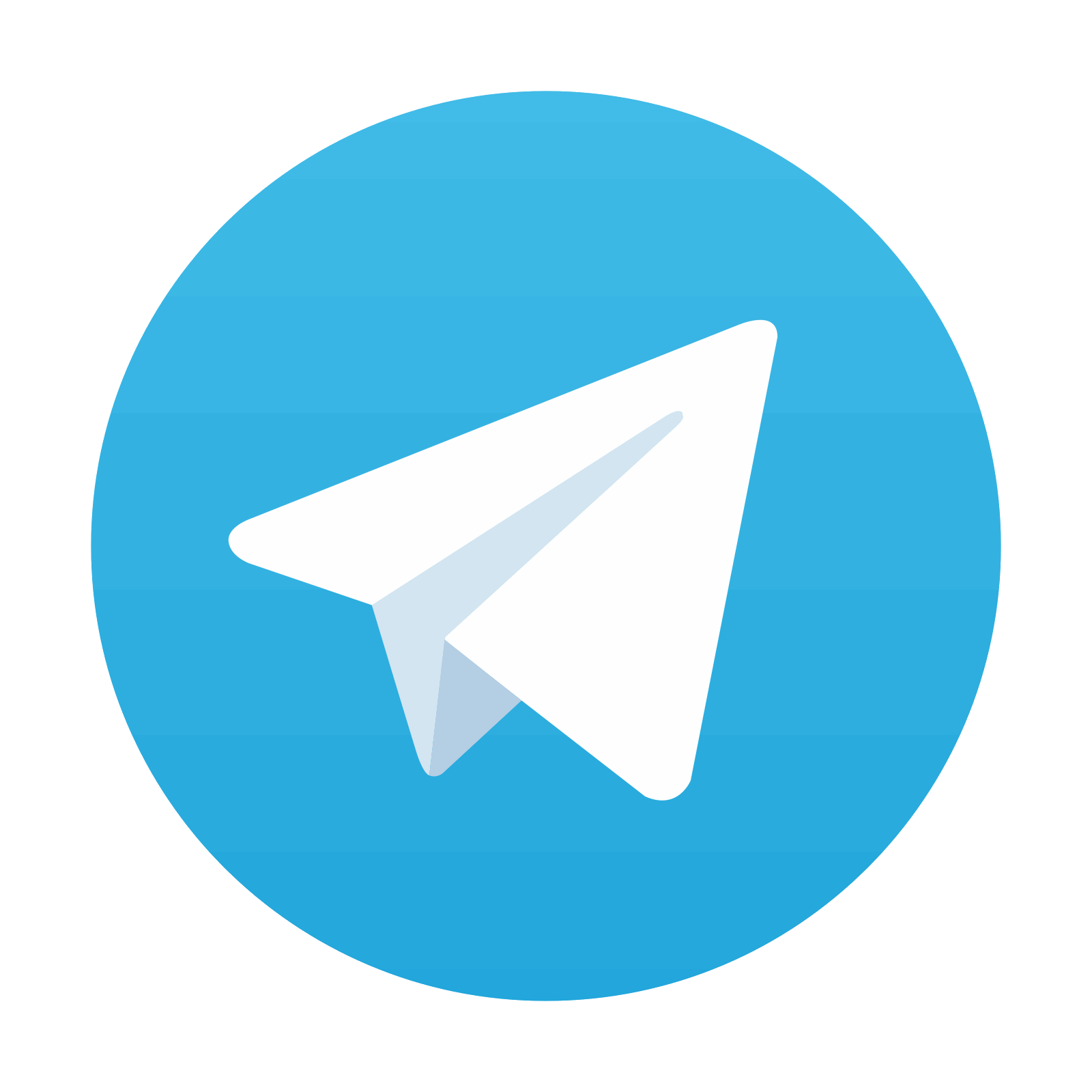
Stay updated, free dental videos. Join our Telegram channel

VIDEdental - Online dental courses
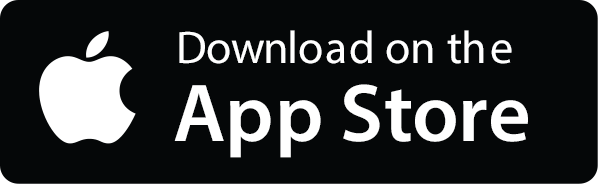
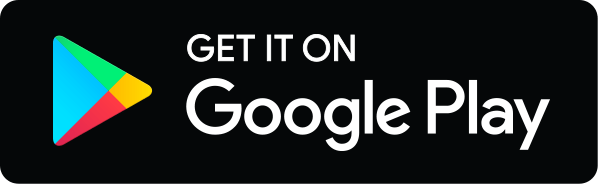